Abstract
Ten mutants of bacteriorhodopsin, each containing a single cysteine residue regularly spaced along helix D and facing the lipid bilayer, were derivatized with a nitroxide spin label. Collision rates of the nitroxide with apolar oxygen increased with distance from the membrane/solution interface. Collision rates with polar metal ion complexes decreased over the same distance. Although the collision rates depend on steric constraints imposed by the local protein structure and on the depth in the membrane, the ratio of the collision rate of oxygen to those of a polar metal ion complex is independent of structural features of the protein. The logarithm of the ratio is a linear function of depth within the membrane. Calibration of this ratio parameter with spin-labeled phospholipids allows localization of the individual nitroxides, and hence the bacteriorhodopsin molecule, relative to the plane of the phosphate groups of the bilayer. The spacing between residues is consistent with the pitch of an alpha-helix. These results provide a general strategy for determining the immersion depth of nitroxides in bilayers.
Full text
PDF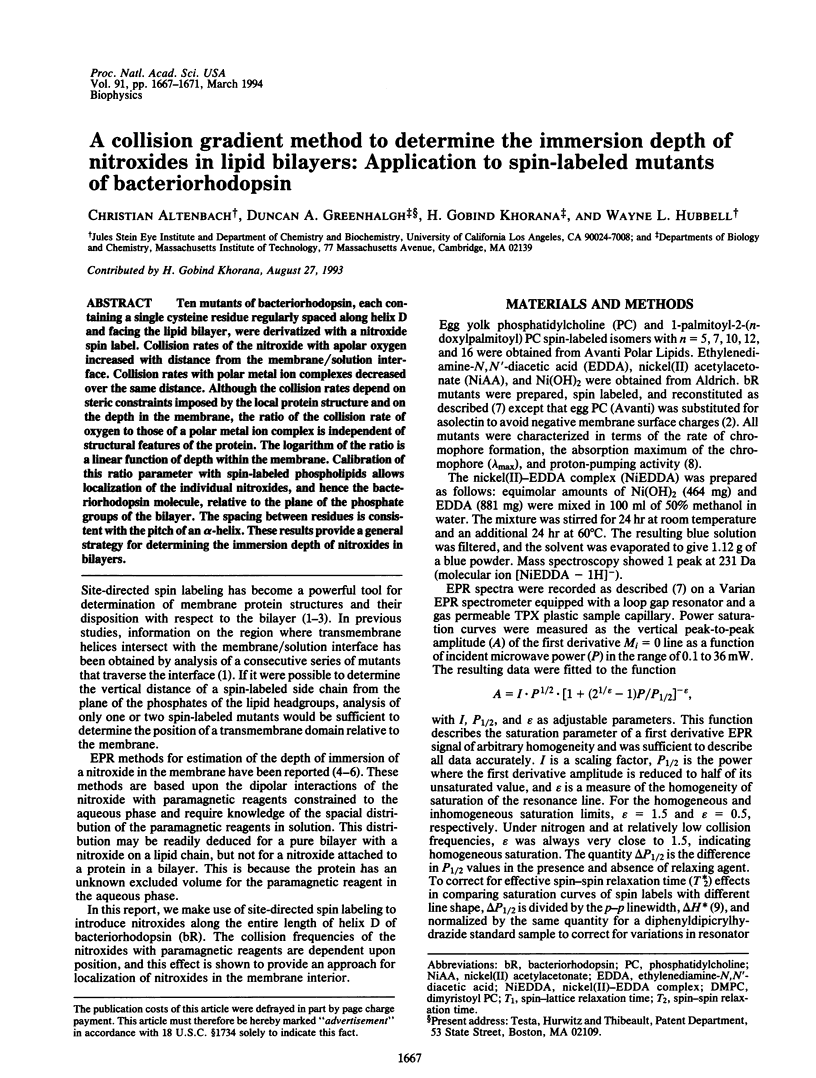
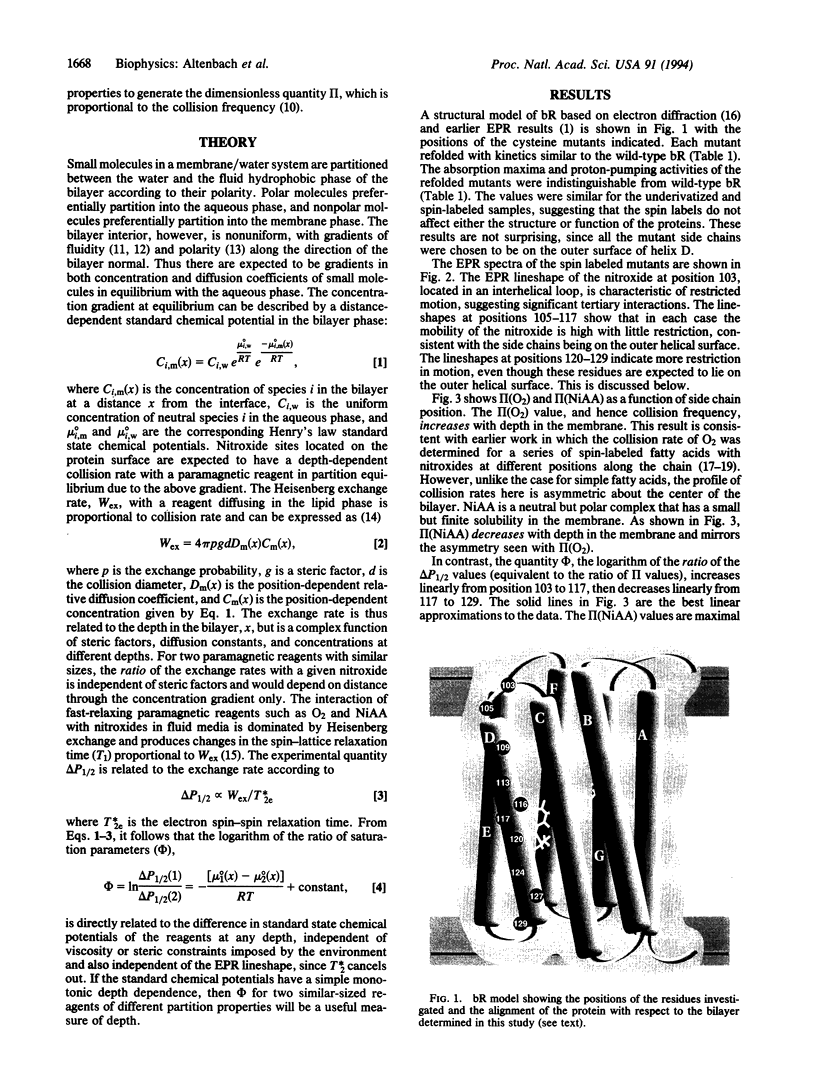
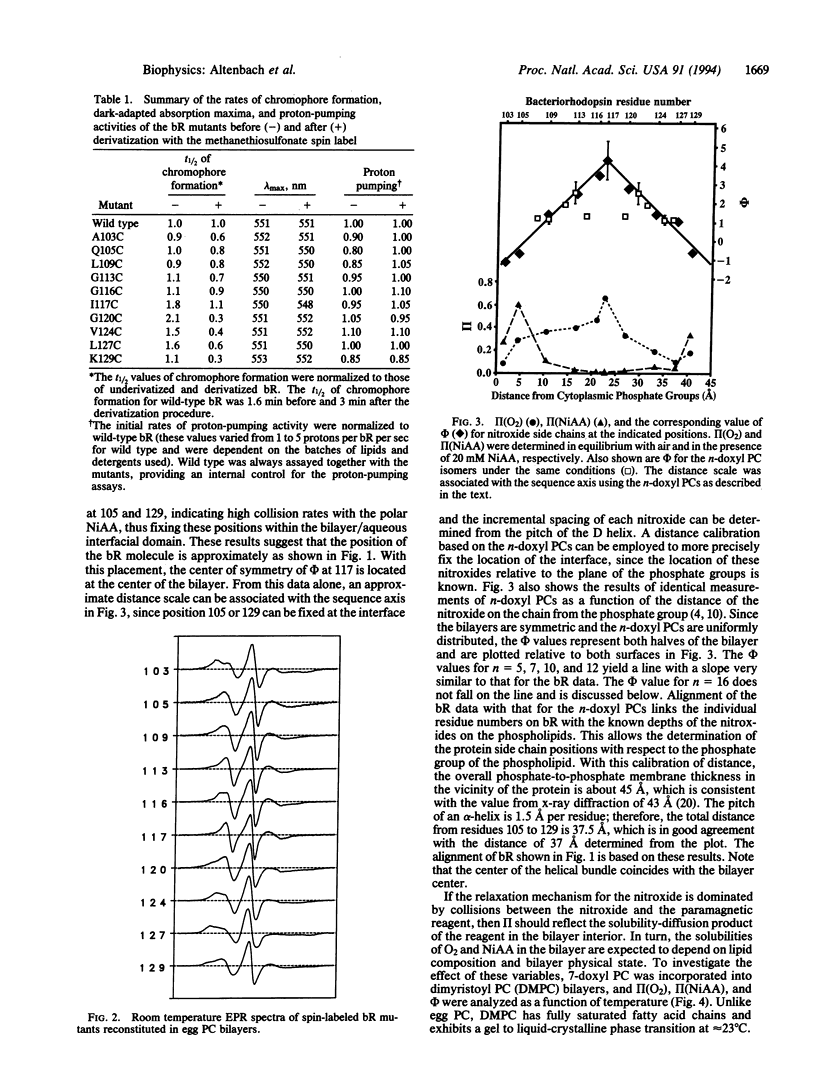
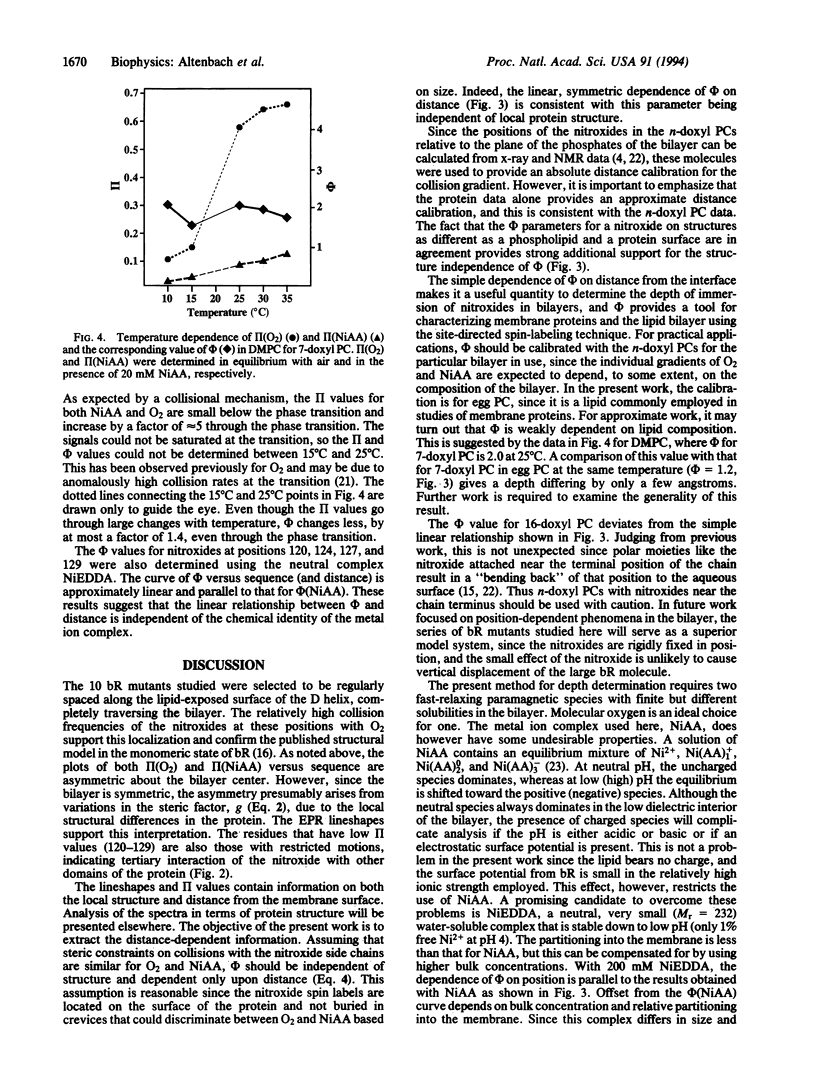
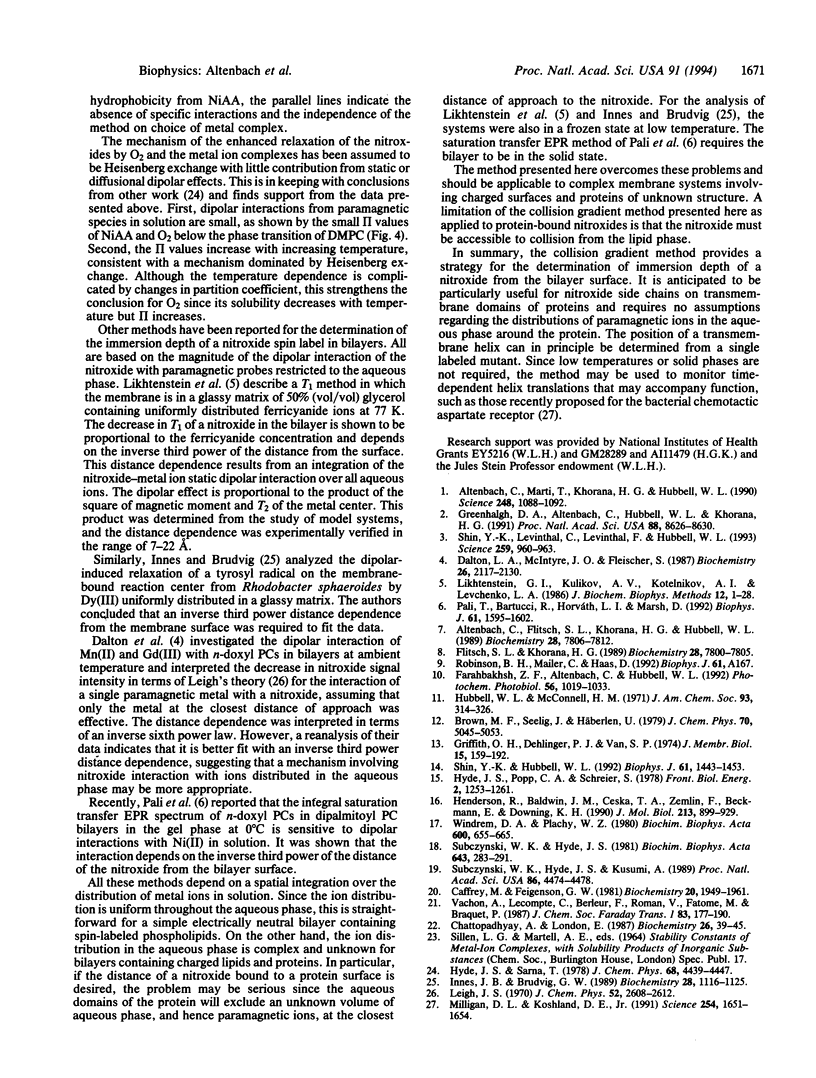
Images in this article
Selected References
These references are in PubMed. This may not be the complete list of references from this article.
- Altenbach C., Flitsch S. L., Khorana H. G., Hubbell W. L. Structural studies on transmembrane proteins. 2. Spin labeling of bacteriorhodopsin mutants at unique cysteines. Biochemistry. 1989 Sep 19;28(19):7806–7812. doi: 10.1021/bi00445a042. [DOI] [PubMed] [Google Scholar]
- Altenbach C., Marti T., Khorana H. G., Hubbell W. L. Transmembrane protein structure: spin labeling of bacteriorhodopsin mutants. Science. 1990 Jun 1;248(4959):1088–1092. doi: 10.1126/science.2160734. [DOI] [PubMed] [Google Scholar]
- Caffrey M., Feigenson G. W. Fluorescence quenching in model membranes. 3. Relationship between calcium adenosinetriphosphatase enzyme activity and the affinity of the protein for phosphatidylcholines with different acyl chain characteristics. Biochemistry. 1981 Mar 31;20(7):1949–1961. doi: 10.1021/bi00510a034. [DOI] [PubMed] [Google Scholar]
- Chattopadhyay A., London E. Parallax method for direct measurement of membrane penetration depth utilizing fluorescence quenching by spin-labeled phospholipids. Biochemistry. 1987 Jan 13;26(1):39–45. doi: 10.1021/bi00375a006. [DOI] [PubMed] [Google Scholar]
- Dalton L. A., McIntyre J. O., Fleischer S. Distance estimate of the active center of D-beta-hydroxybutyrate dehydrogenase from the membrane surface. Biochemistry. 1987 Apr 21;26(8):2117–2130. doi: 10.1021/bi00382a009. [DOI] [PubMed] [Google Scholar]
- Farahbakhsh Z. T., Altenbach C., Hubbell W. L. Spin labeled cysteines as sensors for protein-lipid interaction and conformation in rhodopsin. Photochem Photobiol. 1992 Dec;56(6):1019–1033. doi: 10.1111/j.1751-1097.1992.tb09725.x. [DOI] [PubMed] [Google Scholar]
- Flitsch S. L., Khorana H. G. Structural studies on transmembrane proteins. 1. Model study using bacteriorhodopsin mutants containing single cysteine residues. Biochemistry. 1989 Sep 19;28(19):7800–7805. doi: 10.1021/bi00445a041. [DOI] [PubMed] [Google Scholar]
- Greenhalgh D. A., Altenbach C., Hubbell W. L., Khorana H. G. Locations of Arg-82, Asp-85, and Asp-96 in helix C of bacteriorhodopsin relative to the aqueous boundaries. Proc Natl Acad Sci U S A. 1991 Oct 1;88(19):8626–8630. doi: 10.1073/pnas.88.19.8626. [DOI] [PMC free article] [PubMed] [Google Scholar]
- Griffith O. H., Dehlinger P. J., Van S. P. Shape of the hydrophobic barrier of phospholipid bilayers (evidence for water penetration in biological membranes). J Membr Biol. 1974;15(2):159–192. doi: 10.1007/BF01870086. [DOI] [PubMed] [Google Scholar]
- Henderson R., Baldwin J. M., Ceska T. A., Zemlin F., Beckmann E., Downing K. H. Model for the structure of bacteriorhodopsin based on high-resolution electron cryo-microscopy. J Mol Biol. 1990 Jun 20;213(4):899–929. doi: 10.1016/S0022-2836(05)80271-2. [DOI] [PubMed] [Google Scholar]
- Hubbell W. L., McConnell H. M. Molecular motion in spin-labeled phospholipids and membranes. J Am Chem Soc. 1971 Jan 27;93(2):314–326. doi: 10.1021/ja00731a005. [DOI] [PubMed] [Google Scholar]
- Innes J. B., Brudvig G. W. Location and magnetic relaxation properties of the stable tyrosine radical in photosystem II. Biochemistry. 1989 Feb 7;28(3):1116–1125. doi: 10.1021/bi00429a028. [DOI] [PubMed] [Google Scholar]
- Likhtenstein G. I., Kulikov A. V., Kotelnikov A. I., Levchenko L. A. Methods of physical labels--a combined approach to the study of microstructure and dynamics in biological systems. J Biochem Biophys Methods. 1986 Jan;12(1-2):1–28. doi: 10.1016/0165-022x(86)90047-3. [DOI] [PubMed] [Google Scholar]
- Milligan D. L., Koshland D. E., Jr Intrasubunit signal transduction by the aspartate chemoreceptor. Science. 1991 Dec 13;254(5038):1651–1654. doi: 10.1126/science.1661030. [DOI] [PubMed] [Google Scholar]
- Páli T., Bartucci R., Horváth L. I., Marsh D. Distance measurements using paramagnetic ion-induced relaxation in the saturation transfer electron spin resonance of spin-labeled biomolecules: Application to phospholipid bilayers and interdigitated gel phases. Biophys J. 1992 Jun;61(6):1595–1602. doi: 10.1016/S0006-3495(92)81963-X. [DOI] [PMC free article] [PubMed] [Google Scholar]
- Shin Y. K., Hubbell W. L. Determination of electrostatic potentials at biological interfaces using electron-electron double resonance. Biophys J. 1992 Jun;61(6):1443–1453. doi: 10.1016/S0006-3495(92)81950-1. [DOI] [PMC free article] [PubMed] [Google Scholar]
- Shin Y. K., Levinthal C., Levinthal F., Hubbell W. L. Colicin E1 binding to membranes: time-resolved studies of spin-labeled mutants. Science. 1993 Feb 12;259(5097):960–963. doi: 10.1126/science.8382373. [DOI] [PubMed] [Google Scholar]
- Subczynski W. K., Hyde J. S., Kusumi A. Oxygen permeability of phosphatidylcholine--cholesterol membranes. Proc Natl Acad Sci U S A. 1989 Jun;86(12):4474–4478. doi: 10.1073/pnas.86.12.4474. [DOI] [PMC free article] [PubMed] [Google Scholar]
- Subczynski W. K., Hyde J. S. The diffusion-concentration product of oxygen in lipid bilayers using the spin-label T1 method. Biochim Biophys Acta. 1981 May 6;643(2):283–291. [PubMed] [Google Scholar]
- Windrem D. A., Plachy W. Z. The diffusion-solubility of oxygen in lipid bilayers. Biochim Biophys Acta. 1980 Aug 14;600(3):655–665. doi: 10.1016/0005-2736(80)90469-1. [DOI] [PubMed] [Google Scholar]