Abstract
After reduction by dithiothreitol and removal of the reductant by molecular sieve chromatography, the four interchain disulfide bonds of the human IgGlk protein Fro reoxidize in the presence of oxygen and trace metal ions. The six molecular components of the reoxidation--L (light chain), H (heavy chain), HL, H2, H2L, H2L2--are quantitatively determined from polyacrylamide gels containing sodium dodecyl sulfate and the time-dependent sulfhydryl titer is measured with 5,5'-dithiobis-(2-nitrobenzoic acid). The rates of H2L2 covalent assembly depend on pH in an unexpected way: If the reduced protein is chromatographed at pH 3.2 and then adjusted to pH 7.5 (25 degrees, ionic strength equals 0.14), H2L2 formation proceeds rapidly, with half-times ranging between 20 and 40 min. In contrast, if chromatography is carried out at pH 5.5 before adjusting to the same final conditions, the half-times for H2L2 formation are considerably longer (120-180 min). The half-times in the former case approach the somewhat faster rates of H2L2 assembly observed in pulse-chase experiments with various types of mouse, IgG-producing cells [Baumal, et al. (1971) J. Exp. Med. 134, 1316-1334]. To facilitate comparison of experiments and models, we plot the concentrations of the six components against the corresponding number of sulfhydryl equivalents per mole of Fro. The respective plots for the pH 3.2 leads to 7.5 and 5.5 leads to 7.5 experiments are very similar despite the rate differences. Moreover, these plots differ significantly from the calculated plot for a hypothetical random reoxidation in which the intrinsic probability for formation of each correct HL and H2 disulfide bond is assumed equal and independent. It is concluded that the in vitro reoxidation of Fro (i) is other than random; (ii) involved a pathway of pathways with HL, H2, and H2L precursors; and (iii) involves at least some kinetic cooperativity in bond formation, since no model bases solely on independent bond formation adequately accounts for the results. The models were used also to examine the cellular assembly pathways of mouse IgG proteins.
Full text
PDF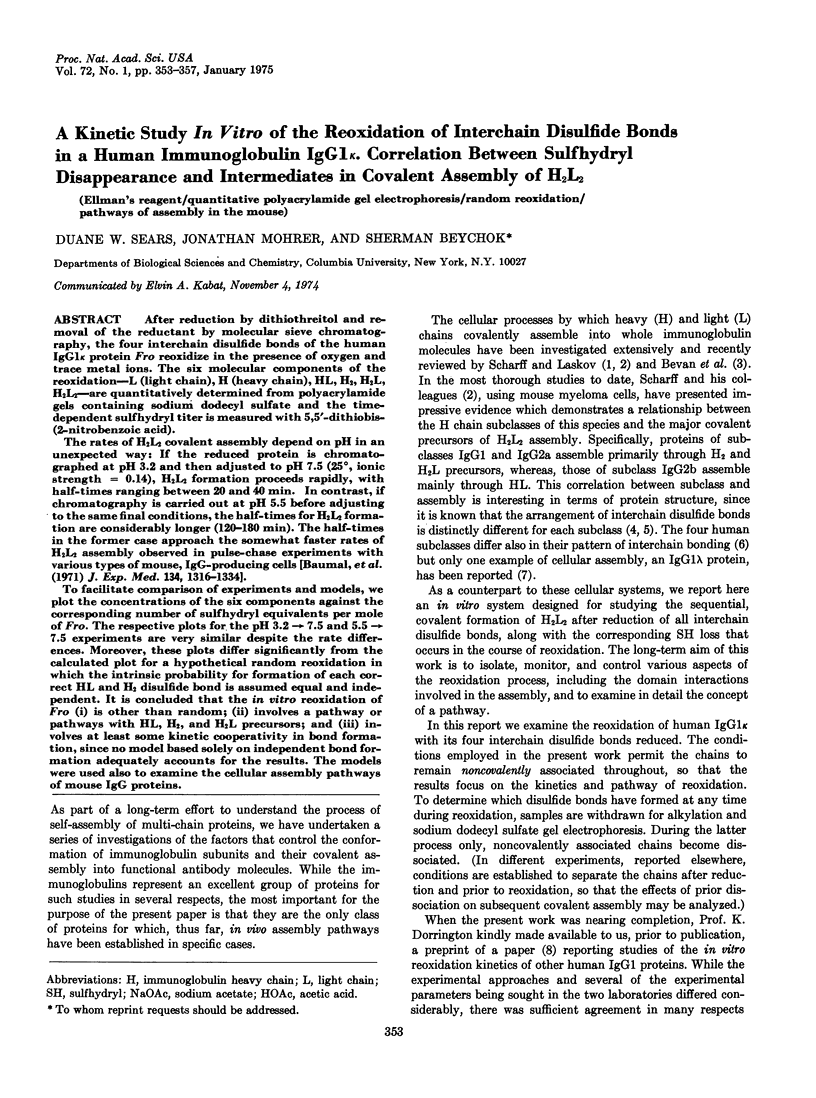
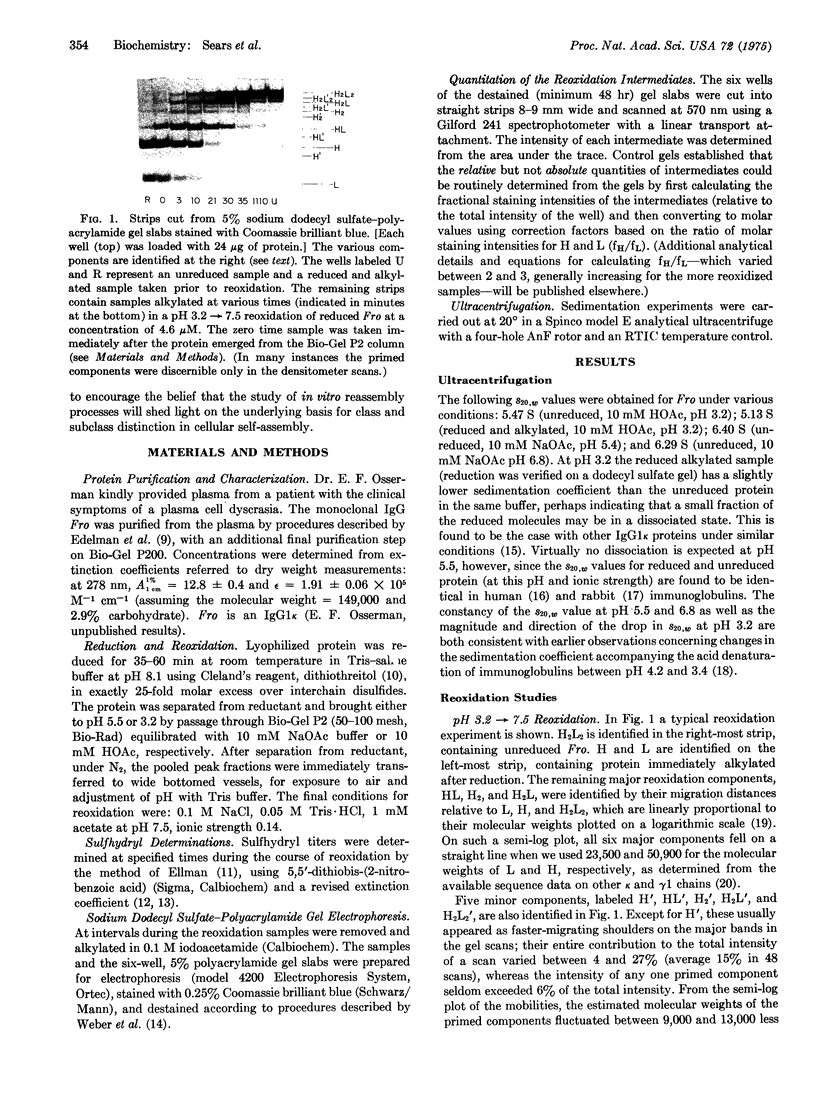
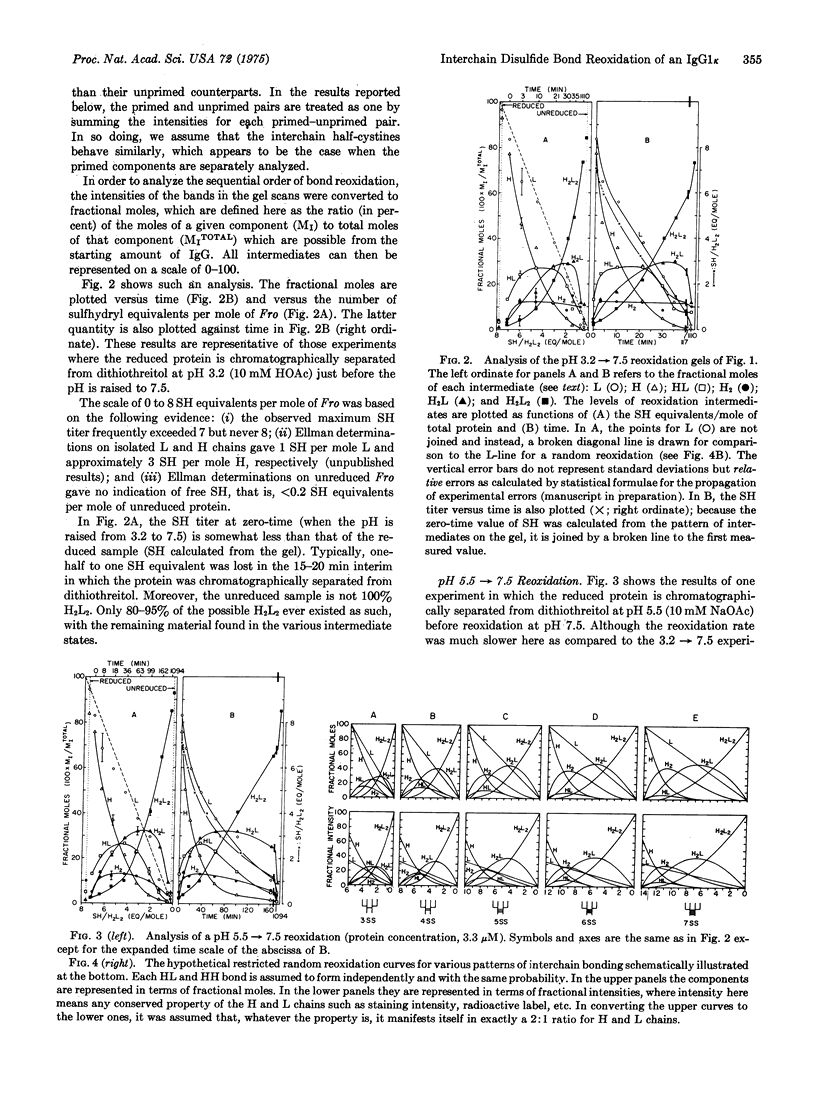
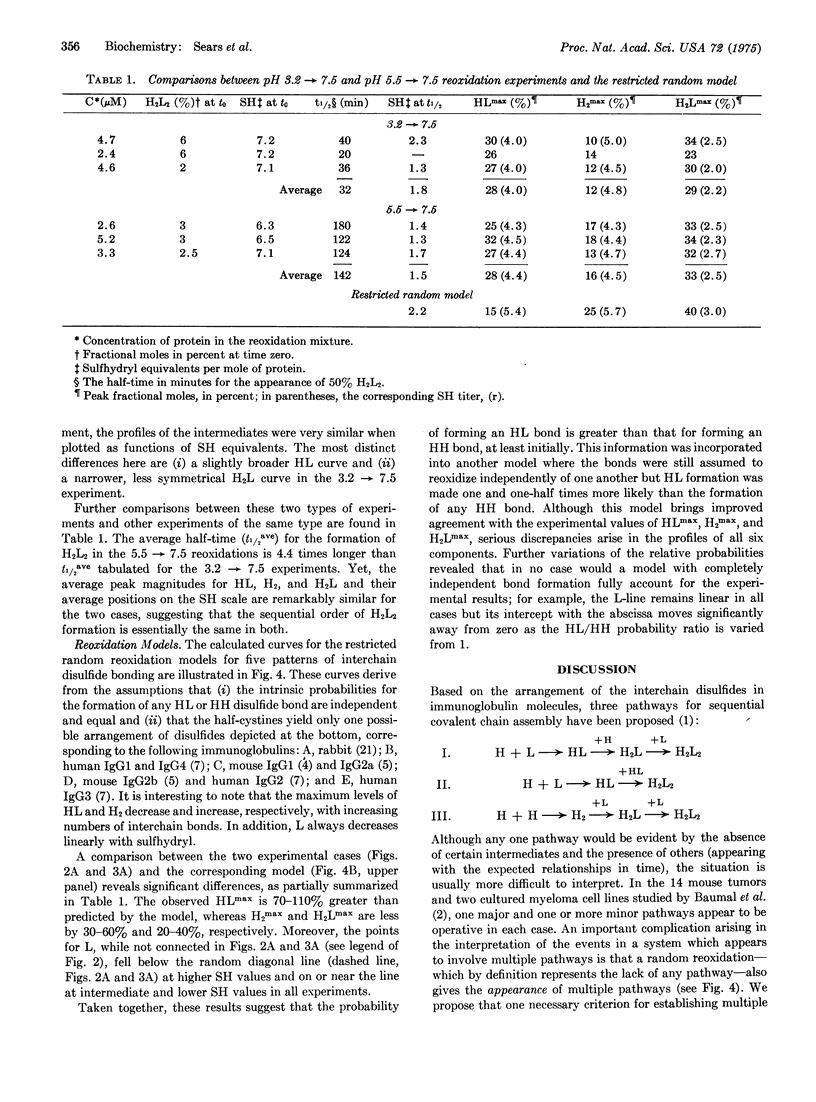
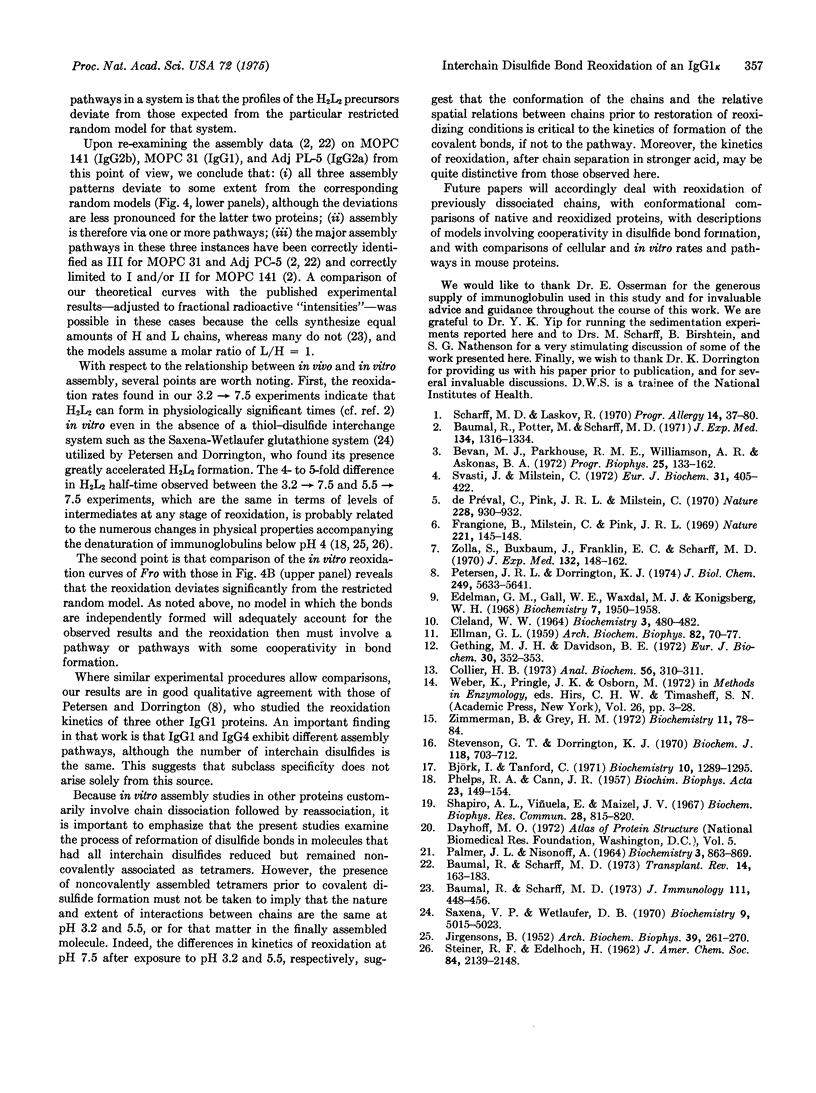
Images in this article
Selected References
These references are in PubMed. This may not be the complete list of references from this article.
- Baumal R., Scharff M. D. Synthesis, assembly and secretion of gamma-globulin by mouse myeloma cells. V. Balanced and unbalanced synthesis of heavy and light chains by IgG-producing tumors and cell lines. J Immunol. 1973 Aug;111(2):448–456. [PubMed] [Google Scholar]
- Baumal R., Scharff M. D. Synthesis, assembly and secretion of mouse immunoglobulin. Transplant Rev. 1973;14:163–183. doi: 10.1111/j.1600-065x.1973.tb00106.x. [DOI] [PubMed] [Google Scholar]
- Bevan M. J., Parkhouse R. M., Williamson A. R., Askonas B. A. Biosynthesis of immunoglobulins. Prog Biophys Mol Biol. 1972;25:133–162. doi: 10.1016/0079-6107(72)90018-1. [DOI] [PubMed] [Google Scholar]
- Björk I., Tanford C. Recovery of native conformation of rabbit immunoglobulin G upon recombination of separately renatured heavy and light chains at near-neutral pH. Biochemistry. 1971 Apr 13;10(8):1289–1295. doi: 10.1021/bi00784a003. [DOI] [PubMed] [Google Scholar]
- CLELAND W. W. DITHIOTHREITOL, A NEW PROTECTIVE REAGENT FOR SH GROUPS. Biochemistry. 1964 Apr;3:480–482. doi: 10.1021/bi00892a002. [DOI] [PubMed] [Google Scholar]
- Collier H. B. Letter: A note on the molar absorptivity of reduced Ellman's reagent, 3-carboxylato-4-nitrothiophenolate. Anal Biochem. 1973 Nov;56(1):310–311. doi: 10.1016/0003-2697(73)90196-6. [DOI] [PubMed] [Google Scholar]
- De Préval C., Pink J. R., Milstein C. Variability of interchain binding of immunoglobulins. Interchain bridges of mouse IgG2a and IgG2b. Nature. 1970 Dec 5;228(5275):930–932. doi: 10.1038/228930a0. [DOI] [PubMed] [Google Scholar]
- ELLMAN G. L. Tissue sulfhydryl groups. Arch Biochem Biophys. 1959 May;82(1):70–77. doi: 10.1016/0003-9861(59)90090-6. [DOI] [PubMed] [Google Scholar]
- Edelman G. M., Gall W. E., Waxdal M. J., Konigsberg W. H. The covalent structure of a human gamma G-immunoglobulin. I. Isolation and characterization of the whole molecule, the polypeptide chains, and the tryptic fragments. Biochemistry. 1968 May;7(5):1950–1958. doi: 10.1021/bi00845a045. [DOI] [PubMed] [Google Scholar]
- Frangione B., Milstein C., Pink J. R. Structural studies of immunoglobulin G. Nature. 1969 Jan 11;221(5176):145–148. doi: 10.1038/221145a0. [DOI] [PubMed] [Google Scholar]
- JIRGENSONS B. Optical rotation and viscosity of native and denatured proteins. I. Influence of pH, and the stability of various proteins toward several denaturing reagents. Arch Biochem Biophys. 1952 Aug;39(2):261–270. doi: 10.1016/0003-9861(52)90333-0. [DOI] [PubMed] [Google Scholar]
- PALMER J. L., NISONOFF A. DISSOCIATION OF RABBIT GAMMA-GLOBULIN INTO HALF-MOLECULES AFTER REDUCTION OF ONE LABILE DISULFIDE BOND. Biochemistry. 1964 Jun;3:863–869. doi: 10.1021/bi00894a024. [DOI] [PubMed] [Google Scholar]
- PHELPS R. A., CANN J. R. On the modification of gamma-globulin by acid. Biochim Biophys Acta. 1957 Jan;23(1):149–154. doi: 10.1016/0006-3002(57)90297-4. [DOI] [PubMed] [Google Scholar]
- Petersen J. G., Dorrington K. J. An in vitro system for studying the kinetics of interchain disulfide bond formation in immunoglobulin G. J Biol Chem. 1974 Sep 10;249(17):5633–5641. [PubMed] [Google Scholar]
- Saxena V. P., Wetlaufer D. B. Formation of three-dimensional structure in proteins. I. Rapid nonenzymic reactivation of reduced lysozyme. Biochemistry. 1970 Dec 8;9(25):5015–5023. doi: 10.1021/bi00827a028. [DOI] [PubMed] [Google Scholar]
- Scharff M. D., Laskov R. Synthesis and assembly of immunoglobulin polypeptide chains. Prog Allergy. 1970;14:37–80. [PubMed] [Google Scholar]
- Shapiro A. L., Viñuela E., Maizel J. V., Jr Molecular weight estimation of polypeptide chains by electrophoresis in SDS-polyacrylamide gels. Biochem Biophys Res Commun. 1967 Sep 7;28(5):815–820. doi: 10.1016/0006-291x(67)90391-9. [DOI] [PubMed] [Google Scholar]
- Stevenson G. T., Dorrington K. J. The recombination of dimers of immunoglobulin peptide chains. Biochem J. 1970 Aug;118(5):703–712. doi: 10.1042/bj1180703. [DOI] [PMC free article] [PubMed] [Google Scholar]
- Svasti J., Milstein C. The parallel nature of the interchain disulphide bonds of immunoglobulins. Studies on a mouse IgG1 myeloma protein. Eur J Biochem. 1972 Dec 18;31(3):405–422. doi: 10.1111/j.1432-1033.1972.tb02547.x. [DOI] [PubMed] [Google Scholar]
- Zimmerman B., Grey H. M. Noncovalent interactions between immunoglobulin polypeptide chains. Stability to dissociation by denaturants. Biochemistry. 1972 Jan 4;11(1):78–84. doi: 10.1021/bi00751a014. [DOI] [PubMed] [Google Scholar]
- Zolla S., Buxbaum J., Franklin E. C., Scharff M. D. Synthesis and assembly of immunoglobulins by malignant human plasmacytes. I. Myelomas producing gamma-chains and light chains. J Exp Med. 1970 Jul 1;132(1):148–162. doi: 10.1084/jem.132.1.148. [DOI] [PMC free article] [PubMed] [Google Scholar]