Abstract
Alkaline phosphatase of E. coli, isolated by procedures which do not alter its intrinsic metal content, contains 1.3 +/- 0.3 g-atom of magnesium and 4.0 +/- 0.2 g-atom of zinc per molecule of molecular weight 89,000. Magnesium, the role of which has been unappreciated, significantly affects the function and structure of alkaline phosphatase containing either 2 or 4 g-atom of zinc per mole. Magnesium does not activate the apoenzyme but increases the activity of the enzyme containing 2 g-atom of zinc 4.4-fold and that of the enzyme containing 4 g-atom 1.2-fold. The results obtained with enzyme in which cobalt is substituted for zinc are analogous. Moreover, the absorption and electron paramagnetic resonance spectra of cobalt phosphatases reveal the effects of magnesium on cobalt coordination geometry. Addition of magnesium changes the spectral characteristics of the apoenzyme reconstituted with 2 g-atom of cobalt from predominantly octahedral to 4- or 5-coordinate geometry. These two classes of cobalt binding sites have been associated with catalysis and structure stabilization, respectively. Therefore, magnesium controls the occupancy of the catalytic and structural binding sites and modulates the resultant enzymatic activity. Hydrogen-tritium exchange was employed to determine the effects of magnesium on the conformational stability of phosphatase. Magnesium stabilizes the dynamic structural properties, both of apophosphatase and of enzyme containing 2 g-atom of zinc, which is further stabilized by 2 more zinc atoms. The role of magnesium and other metal ions in regulatory processes, only now beginning to be explored fully, will likely emerge as an important avenue for achievement of regulatory effects in metalloenzymes.
Full text
PDF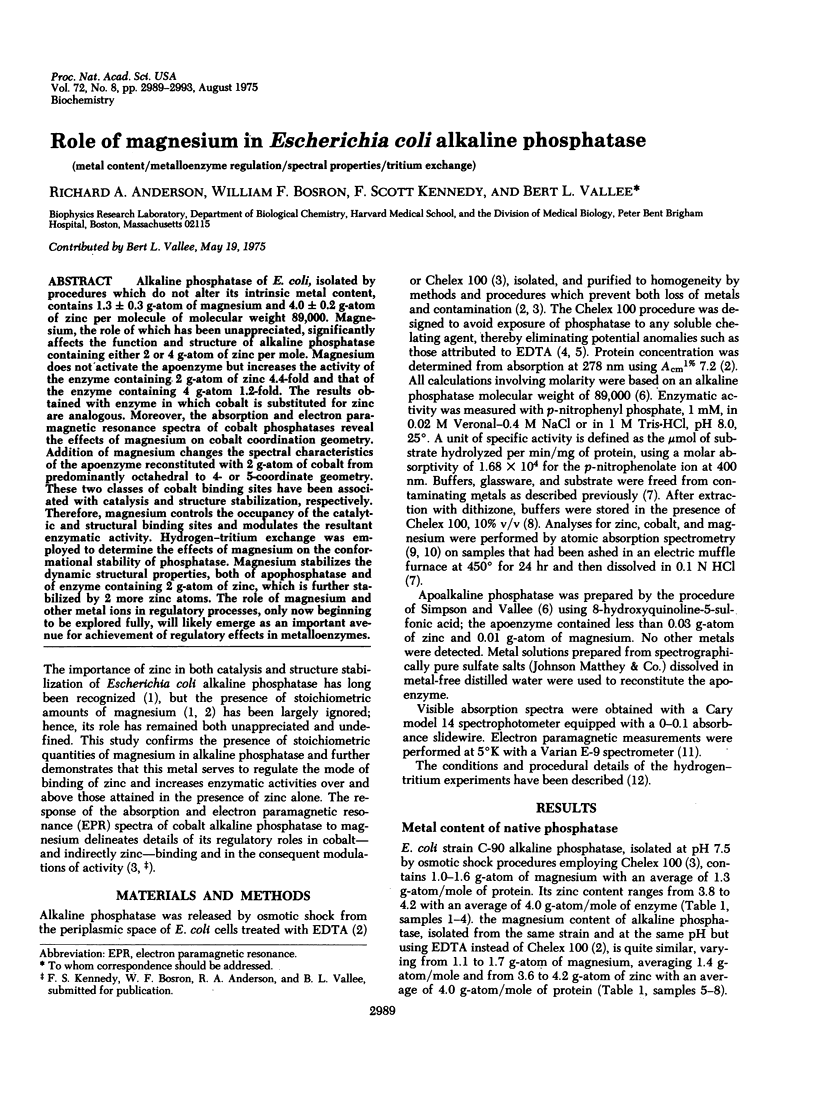
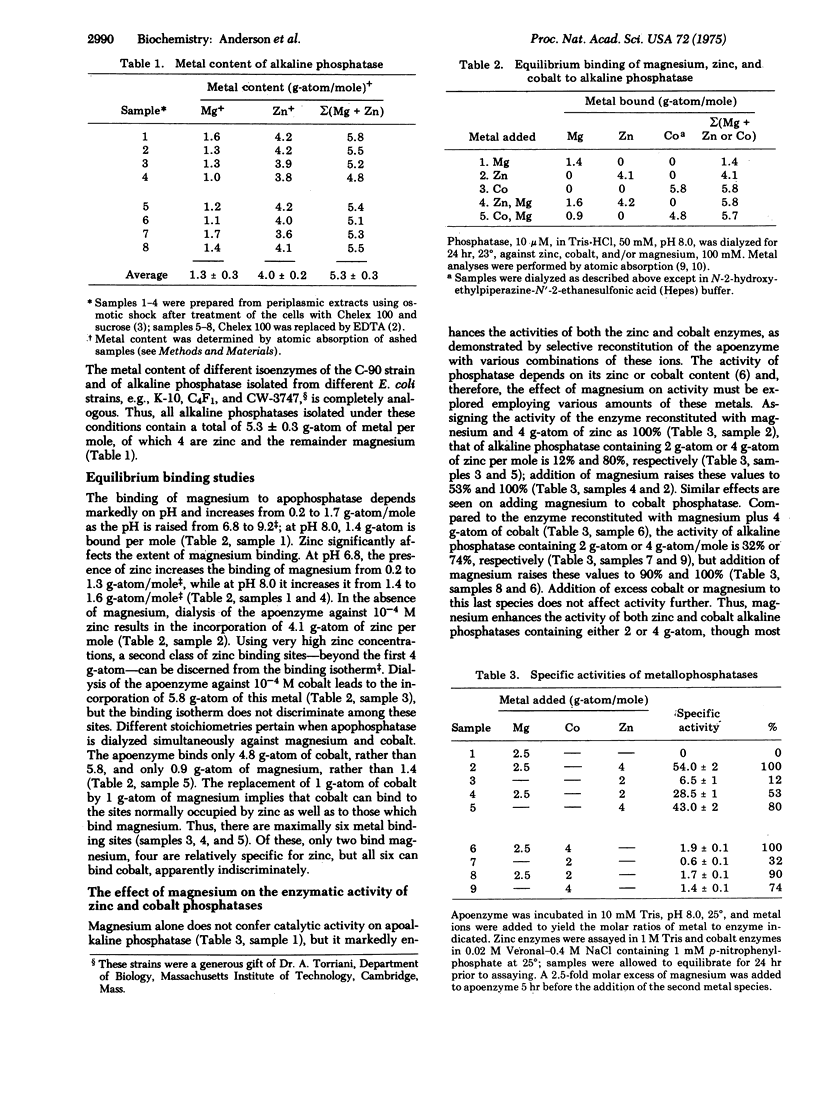
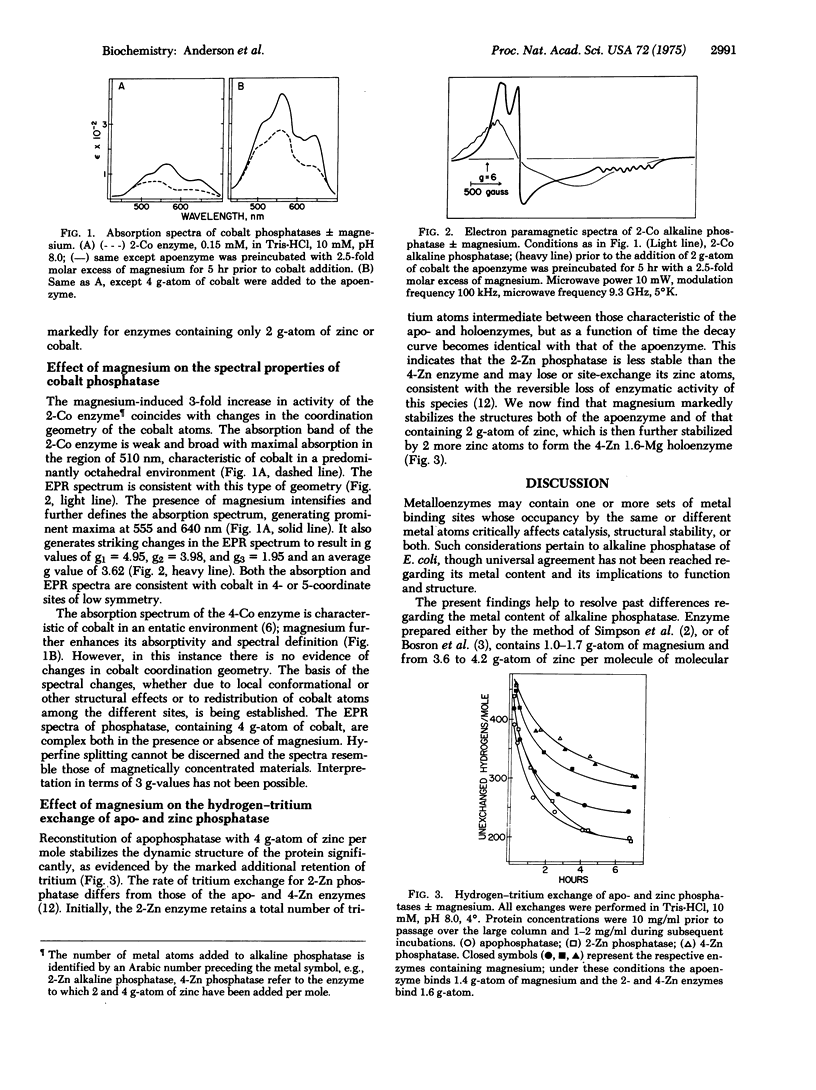
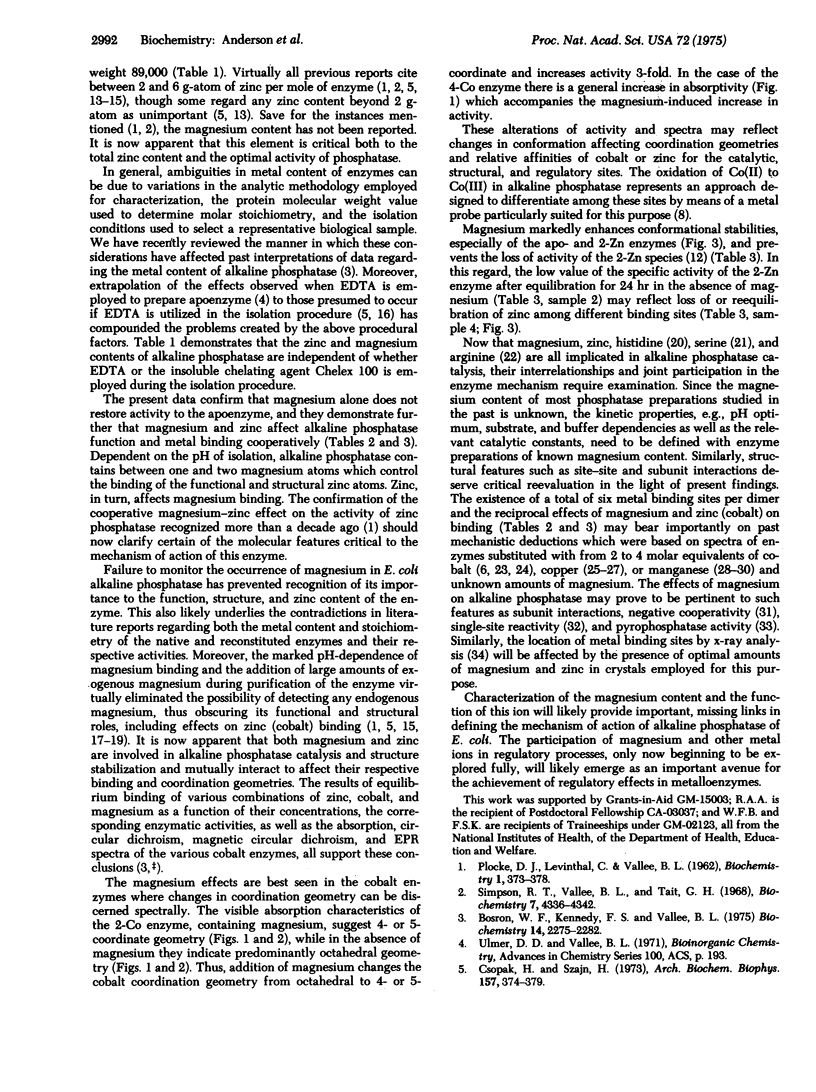
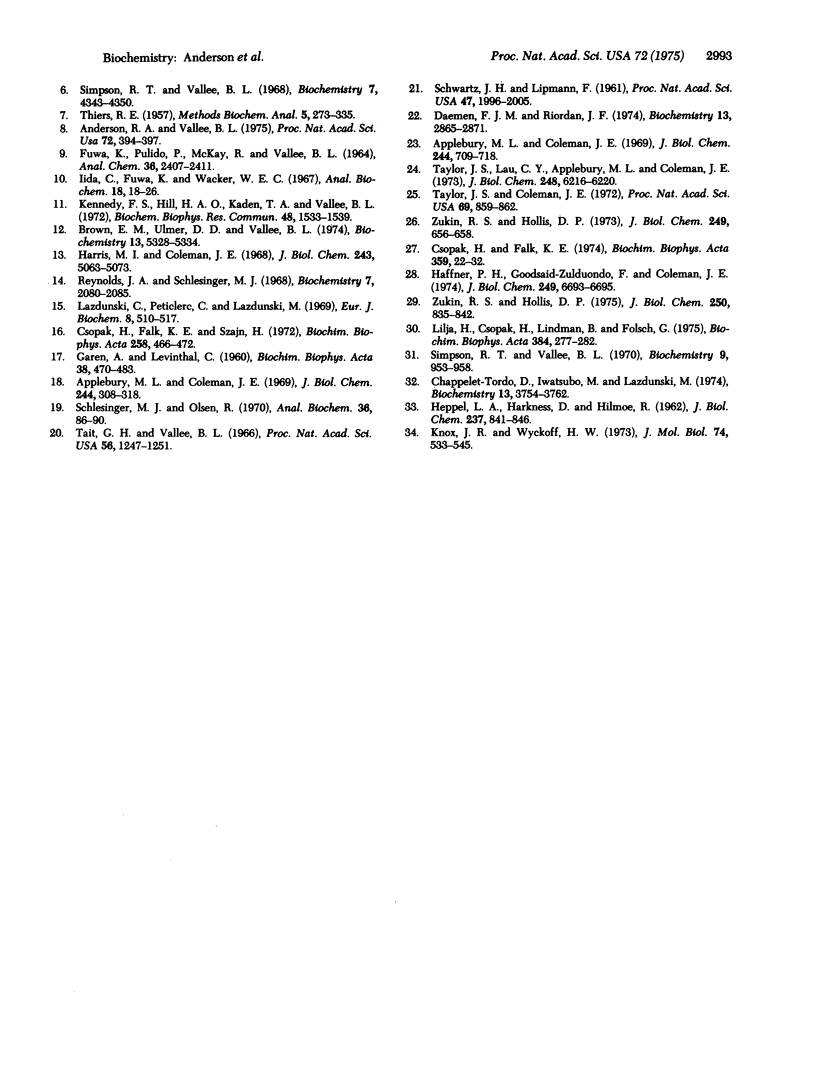
Selected References
These references are in PubMed. This may not be the complete list of references from this article.
- Anderson R. A., Vallee B. L. Cobalt(III), a probe of metal binding sites of Escherichia coli alkaline phosphatase. Proc Natl Acad Sci U S A. 1975 Jan;72(1):394–397. doi: 10.1073/pnas.72.1.394. [DOI] [PMC free article] [PubMed] [Google Scholar]
- Applebury M. L., Coleman J. E. Escherichia coli alkaline phosphatase. Metal binding, protein conformation, and quaternary structure. J Biol Chem. 1969 Jan 25;244(2):308–318. [PubMed] [Google Scholar]
- Applebury M. L., Coleman J. E. Escherichia coli co (II) alkaline phsophatase. J Biol Chem. 1969 Feb 25;244(4):709–718. [PubMed] [Google Scholar]
- Bosron W. F., Kennedy F. S., Vallee B. L. Zinc and magnesium content of alkaline phosphatase from Escherichia coli. Biochemistry. 1975 May 20;14(10):2275–2282. doi: 10.1021/bi00681a036. [DOI] [PubMed] [Google Scholar]
- Brown E. M., Ulmer D. D., Vallee B. L. Hydrogen-tritium exchange of partially and fully reconstituted zinc and cobalt alkaline phosphatase of Escherichia coli. Biochemistry. 1974 Dec 17;13(26):5328–5334. doi: 10.1021/bi00723a012. [DOI] [PubMed] [Google Scholar]
- Chappelet-Tordo D., Iwatsubo M., Lazdunski M. Negative cooperativity and half of the sites reactivity. Alkaline phosphatases of Escherichia coli with Zn2+, Co2+, Cd2+, Mn2+, and Cu2+ in the active sites. Biochemistry. 1974 Aug 27;13(18):3754–3762. doi: 10.1021/bi00715a022. [DOI] [PubMed] [Google Scholar]
- Csopak H., Falk K. E. Electron paramagnetic resonance studies on the copper(II) substituted alkaline phosphatase from Escherichia coli. Biochim Biophys Acta. 1974 Jul 7;359(1):22–32. doi: 10.1016/0005-2795(74)90128-7. [DOI] [PubMed] [Google Scholar]
- Csopak H., Falk K. E., Szajn H. Effect of EDTA on Escherichia coli alkaline phosphatase. Biochim Biophys Acta. 1972 Feb 28;258(2):466–472. doi: 10.1016/0005-2744(72)90238-0. [DOI] [PubMed] [Google Scholar]
- Csopak H., Szajn H. Factors affecting the zinc content of E. coli alkaline phosphatase. Arch Biochem Biophys. 1973 Aug;157(2):374–379. doi: 10.1016/0003-9861(73)90652-8. [DOI] [PubMed] [Google Scholar]
- Daemen F. J., Riordan J. F. Essential arginyl residues in Escherichia coli alkaline phosphatase. Biochemistry. 1974 Jul 2;13(14):2865–2871. doi: 10.1021/bi00711a014. [DOI] [PubMed] [Google Scholar]
- GAREN A., LEVINTHAL C. A fine-structure genetic and chemical study of the enzyme alkaline phosphatase of E. coli. I. Purification and characterization of alkaline phosphatase. Biochim Biophys Acta. 1960 Mar 11;38:470–483. doi: 10.1016/0006-3002(60)91282-8. [DOI] [PubMed] [Google Scholar]
- Gryglewski R., Vane J. R. The release of prostaglandins and rabbit aorta contracting substance (RCS) from rabbit spleen and its antagonism by anti-inflammatory drugs. Br J Pharmacol. 1972 May;45(1):37–47. doi: 10.1111/j.1476-5381.1972.tb09574.x. [DOI] [PMC free article] [PubMed] [Google Scholar]
- HEPPEL L. A., HARKNESS D. R., HILMOE R. J. A study of the substrate specificity and other properties of the alkaline phosphatase of Escherichia coli. J Biol Chem. 1962 Mar;237:841–846. [PubMed] [Google Scholar]
- Haffner P. H., Goodsaid-Zalduondo F., Coleman J. E. Electron spin resonance of manganese(II)-substituted zinc(II) metalloenzymes. J Biol Chem. 1974 Oct 25;249(20):6693–6695. [PubMed] [Google Scholar]
- Hamberg M., Samuelsson B. Prostaglandin endoperoxides. Novel transformations of arachidonic acid in human platelets. Proc Natl Acad Sci U S A. 1974 Sep;71(9):3400–3404. doi: 10.1073/pnas.71.9.3400. [DOI] [PMC free article] [PubMed] [Google Scholar]
- Hamberg M., Svensson J., Samuelsson B. Prostaglandin endoperoxides. A new concept concerning the mode of action and release of prostaglandins. Proc Natl Acad Sci U S A. 1974 Oct;71(10):3824–3828. doi: 10.1073/pnas.71.10.3824. [DOI] [PMC free article] [PubMed] [Google Scholar]
- Hamberg M., Svensson J., Wakabayashi T., Samuelsson B. Isolation and structure of two prostaglandin endoperoxides that cause platelet aggregation. Proc Natl Acad Sci U S A. 1974 Feb;71(2):345–349. doi: 10.1073/pnas.71.2.345. [DOI] [PMC free article] [PubMed] [Google Scholar]
- Harris M. I., Coleman J. E. The biosynthesis of apo- and metalloalkaline phosphatases of Escherichia coli. J Biol Chem. 1968 Oct 10;243(19):5063–5073. [PubMed] [Google Scholar]
- Kennedy F. C., Hill H. A., Kaden T. A., Vallee B. L. Electron paramagnetic resonance spectra of some active cobalt(II) substituted metalloenzymes and other cobalt(II) complexes. Biochem Biophys Res Commun. 1972 Sep 26;48(6):1533–1539. doi: 10.1016/0006-291x(72)90888-1. [DOI] [PubMed] [Google Scholar]
- Knox J. R., Wyckoff H. W. A crystallographic study of alkaline phosphatase at 7-7 Angstrom resolution. J Mol Biol. 1973 Mar 15;74(4):533–545. doi: 10.1016/0022-2836(73)90045-4. [DOI] [PubMed] [Google Scholar]
- Lazdunski C., Petitclerc C., Lazdunski M. Structure-function relationships for some metalloalkaline phosphatases of E. coli. Eur J Biochem. 1969 Apr;8(4):510–517. doi: 10.1111/j.1432-1033.1969.tb00556.x. [DOI] [PubMed] [Google Scholar]
- Lilja H., Csopak H., Lindman B., Fölsch G. 19-F NMR studies of the binding of a fluorine-labeled phosphonate ion to E. coli alkaline phosphatase. Biochim Biophys Acta. 1975 Mar 28;384(1):277–282. doi: 10.1016/0005-2744(75)90116-3. [DOI] [PubMed] [Google Scholar]
- PLOCKE D. J., LEVINTHAL C., VALLEE B. L. Alkaline phosphatase of Escherichia coli: a zinc metalloenzyme. Biochemistry. 1962 May 25;1:373–378. doi: 10.1021/bi00909a001. [DOI] [PubMed] [Google Scholar]
- Piper P. J., Vane J. R. Release of additional factors in anaphylaxis and its antagonism by anti-inflammatory drugs. Nature. 1969 Jul 5;223(5201):29–35. doi: 10.1038/223029a0. [DOI] [PubMed] [Google Scholar]
- Reynolds J. A., Schlesinger M. J. Hydrogen ion equilibria of conformational states of Escherichia coli alkaline phosphatase. Biochemistry. 1968 Jun;7(6):2080–2085. doi: 10.1021/bi00846a009. [DOI] [PubMed] [Google Scholar]
- SCHWARTZ J. H., LIPMANN F. Phosphate incorporation into alkaline phosphatase of E. coli. Proc Natl Acad Sci U S A. 1961 Dec 15;47:1996–2005. doi: 10.1073/pnas.47.12.1996. [DOI] [PMC free article] [PubMed] [Google Scholar]
- Schlesinger M. J., Olsen R. A new, simple, rapid procedure for purification of Escherichia coli alkaline phosphatase. Anal Biochem. 1970 Jul;36(1):86–90. doi: 10.1016/0003-2697(70)90334-9. [DOI] [PubMed] [Google Scholar]
- Simpson R. T., Valee B. L. Negative homotropic interactions in binding of substrate to alkaline phosphatase of Escherichia coli. Biochemistry. 1970 Feb 17;9(4):953–958. doi: 10.1021/bi00806a035. [DOI] [PubMed] [Google Scholar]
- Simpson R. T., Vallee B. L., Tait G. H. Alkaline phosphatase of Escherichia coli. Composition. Biochemistry. 1968 Dec;7(12):4336–4342. doi: 10.1021/bi00852a028. [DOI] [PubMed] [Google Scholar]
- Simpson R. T., Vallee B. L. Two differentiable classes of metal atoms in alkaline phosphatase of Escherichia coli. Biochemistry. 1968 Dec;7(12):4343–4350. doi: 10.1021/bi00852a029. [DOI] [PubMed] [Google Scholar]
- Tait G. H., Vallee B. L. Studies on the active center of alkaline phosphatase of E. coli. Proc Natl Acad Sci U S A. 1966 Oct;56(4):1247–1251. doi: 10.1073/pnas.56.4.1247. [DOI] [PMC free article] [PubMed] [Google Scholar]
- Taylor J. S., Coleman J. E. Nitrogen ligands at the active site of alkaline phosphatase. Proc Natl Acad Sci U S A. 1972 Apr;69(4):859–862. doi: 10.1073/pnas.69.4.859. [DOI] [PMC free article] [PubMed] [Google Scholar]
- Taylor J. S., Lau C. Y., Applebury M. L., Coleman J. E. Escherichia coli Co(II) alkaline phosphatase. Absorption, circular dichroism, and magnetic circular dichroism of the d-d electronic transitions. J Biol Chem. 1973 Sep 10;248(17):6216–6220. [PubMed] [Google Scholar]
- Vargaftig B. B., Dao N. Release of vasoactive substances from guinea-pig lungs by slow-reacting substance c and arachidonic acid. Its blockade by nonsteroid anti-inflammatory agents. Pharmacology. 1971;6(2):99–108. doi: 10.1159/000136231. [DOI] [PubMed] [Google Scholar]
- Vargaftig B. B., Zirinis P. Platelet aggregation induced by arachidonic acid is accompanied by release of potential inflammatory mediators distinct from PGE2 and PGF2. Nat New Biol. 1973 Jul 25;244(134):114–116. doi: 10.1038/newbio244114a0. [DOI] [PubMed] [Google Scholar]
- Zukin R. S., Hollis D. P. A proton relaxation rate study of the copper analog of Escherichia coli alkaline phosphatase. J Biol Chem. 1974 Jan 25;249(2):656–658. [PubMed] [Google Scholar]
- Zukin R. S., Hollis D. P. Role of metal ions in Escherichia coli alkaline phosphatase. A study of the metal-water interaction by nuclear relaxation rate measurements on water protons. J Biol Chem. 1975 Feb 10;250(3):835–842. [PubMed] [Google Scholar]