Abstract
Transport of vinylglycolate (2-hydroxy-3-butenoic acid) via the lactate transport system is the limiting step for covalent labeling of membrane vesicles prepared from E. coli ML 308-225. Thus, the rate and extent of vinylglycolate labeling is stimulated about 10-fold by ascorbate-phenazine methosulfate, and stimulation is abolished by 2,4-dinitrophenol and by phospholipase treatment, neither of which affect the rate of vinylglycolate oxidation. [3H]Vinylglycolate of high specific activity has been prepared, and vesicles have been labeled with this compound in the presence of ascorbate-phenazine methosulfate. Examination of these preparations by high resolution radioautography in the electron microscope demonstrates that virtually all of the vesicles are labeled. The experiments provide a strong indication that most, if not all, of the membrane vesicles in these preparations catalyze active transport.
Keywords: vinylglycolate, 2-hydroxy-3-butenoate, D-lactate, radioautography, electron microscopy
Full text
PDF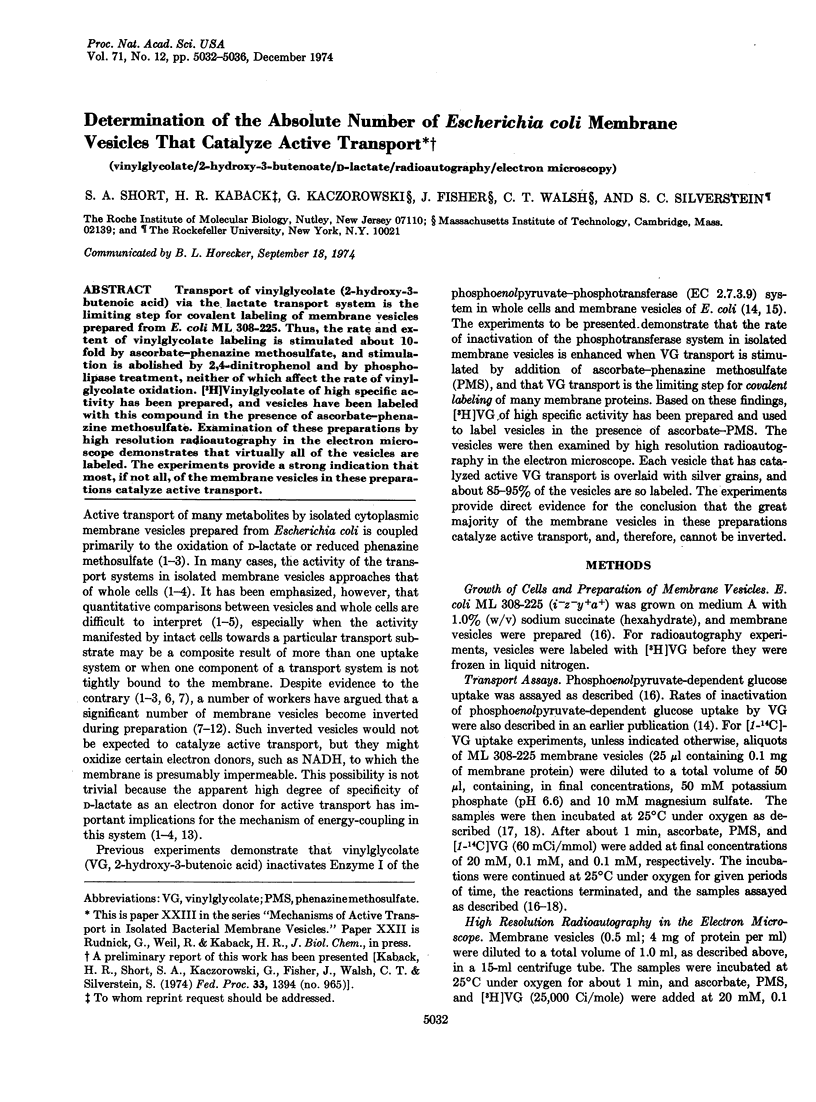
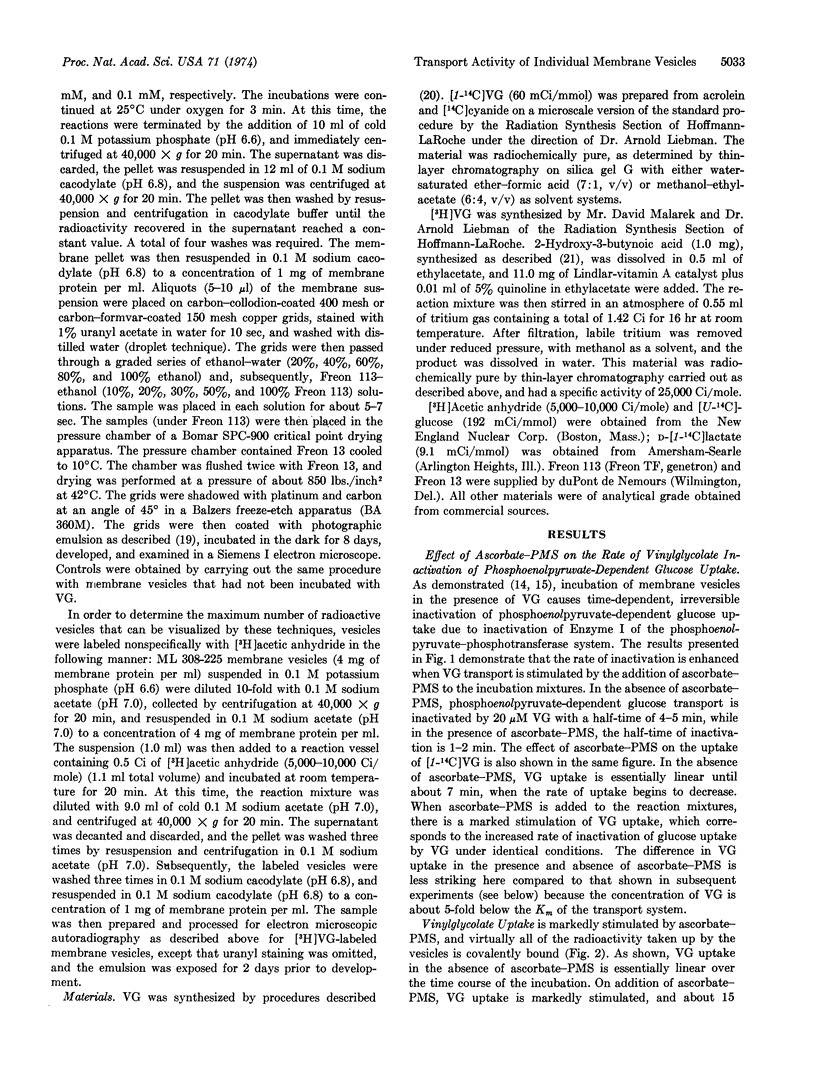
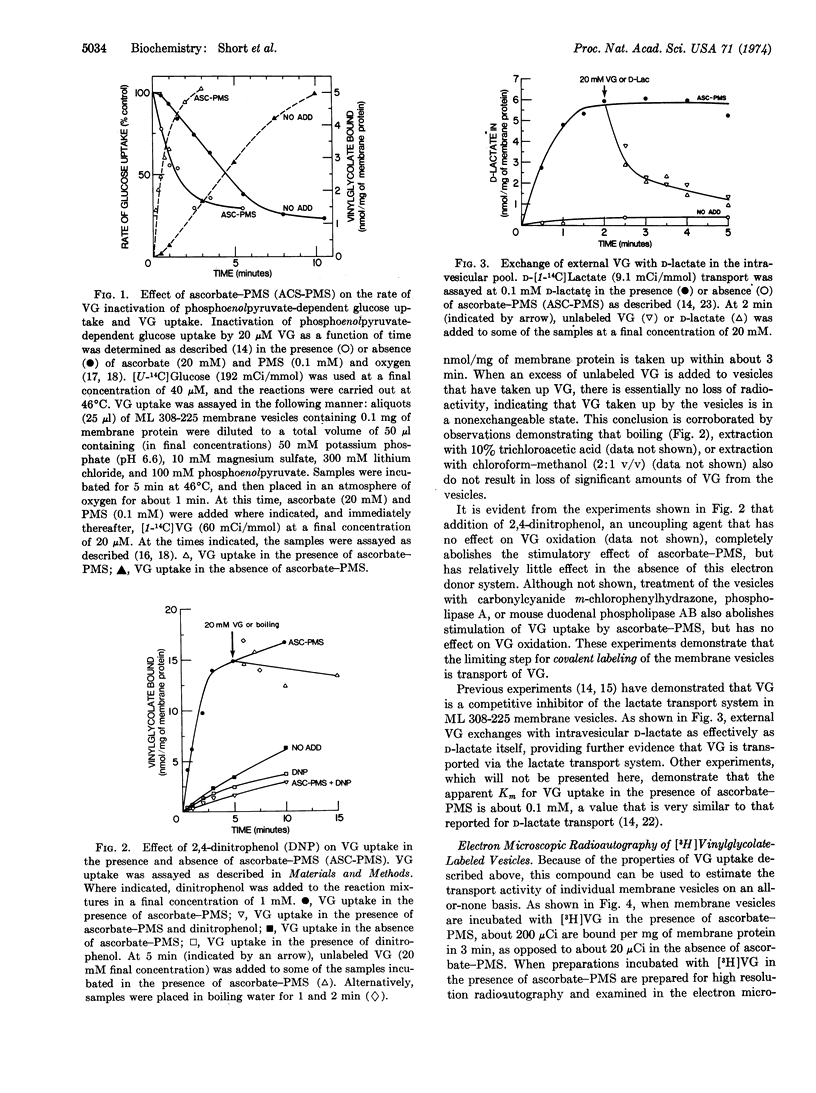
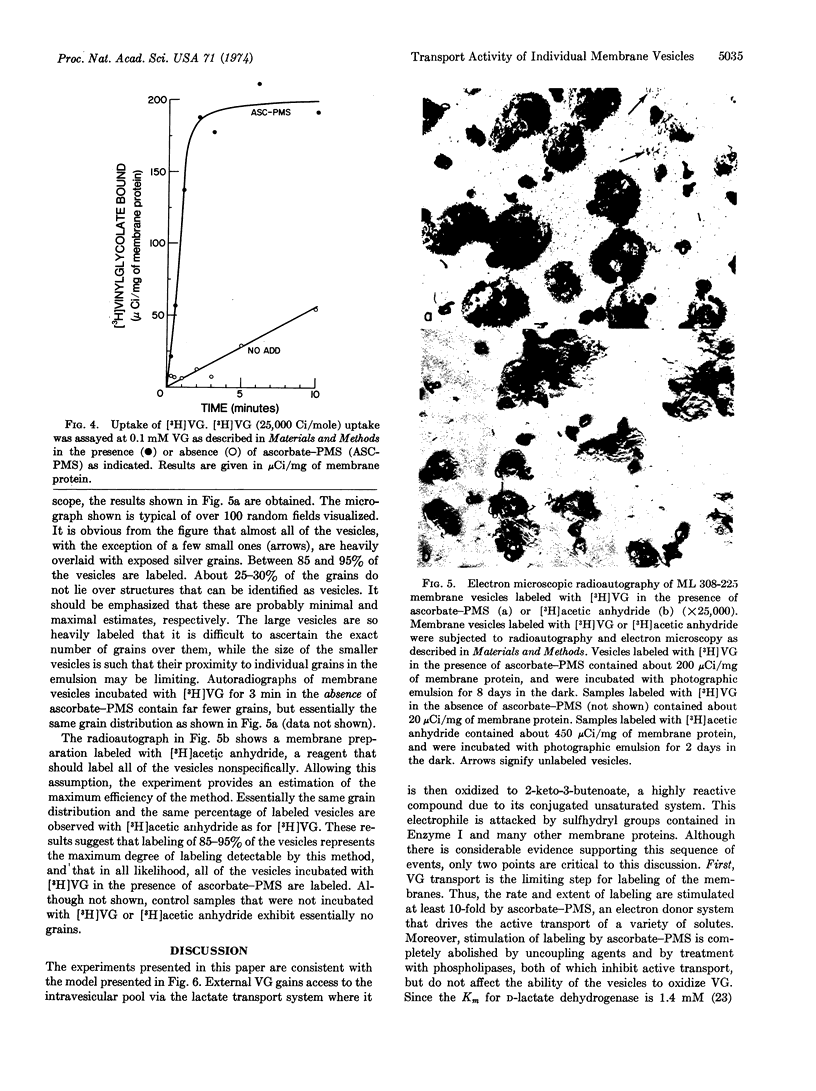
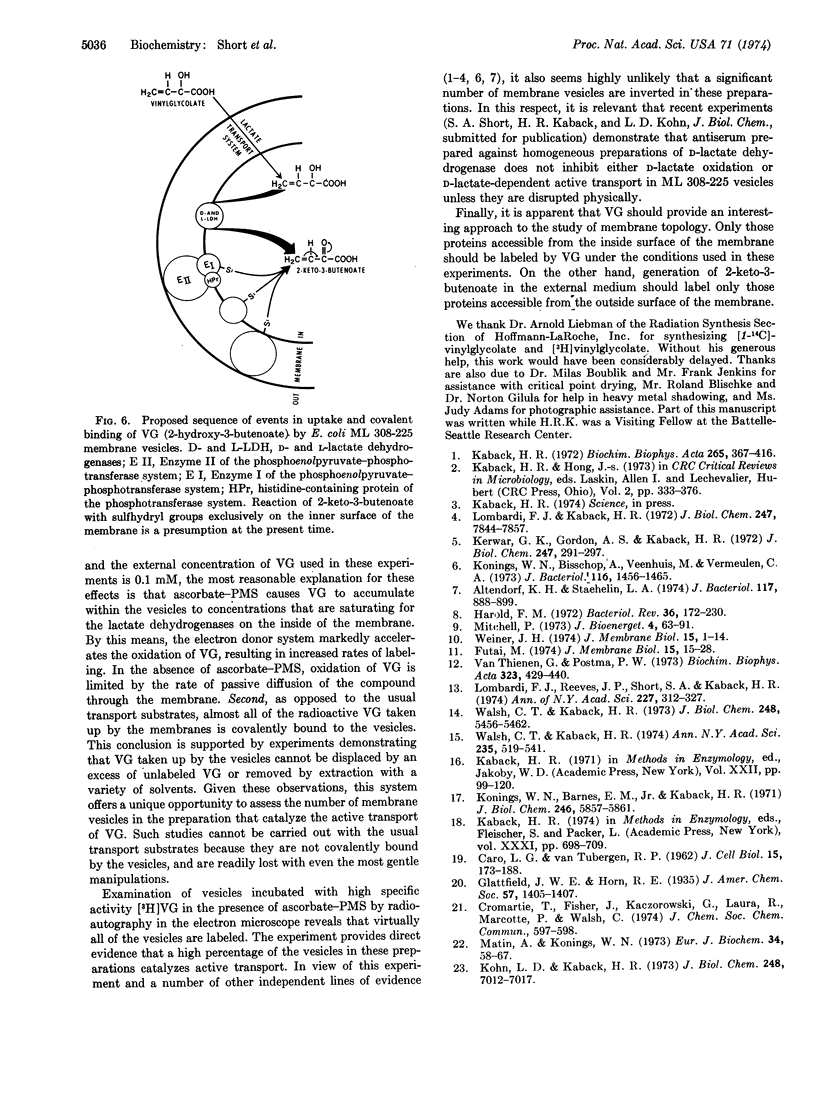
Images in this article
Selected References
These references are in PubMed. This may not be the complete list of references from this article.
- Altendorf K. H., Staehelin L. A. Orientation of membrane vesicles from Escherichia coli as detected by freeze-cleave electron microscopy. J Bacteriol. 1974 Feb;117(2):888–899. doi: 10.1128/jb.117.2.888-899.1974. [DOI] [PMC free article] [PubMed] [Google Scholar]
- CARO L. G., VAN TUBERGEN R. P., KOLB J. A. High-resolution autoradiography. I. Methods. J Cell Biol. 1962 Nov;15:173–188. doi: 10.1083/jcb.15.2.173. [DOI] [PMC free article] [PubMed] [Google Scholar]
- Futai M. Orientation of membrane vesicles from Escherichia coli prepared by different procedures. J Membr Biol. 1974;15(1):15–28. doi: 10.1007/BF01870079. [DOI] [PubMed] [Google Scholar]
- Harold F. M. Conservation and transformation of energy by bacterial membranes. Bacteriol Rev. 1972 Jun;36(2):172–230. doi: 10.1128/br.36.2.172-230.1972. [DOI] [PMC free article] [PubMed] [Google Scholar]
- Kaback H. R. Transport across isolated bacterial cytoplasmic membranes. Biochim Biophys Acta. 1972 Aug 4;265(3):367–416. doi: 10.1016/0304-4157(72)90014-7. [DOI] [PubMed] [Google Scholar]
- Kerwar G. K., Gordon A. S., Kaback H. R. Mechanisms of active transport in isolated membrane vesicles. IV. Galactose transport by isolated membrane vesicles from Escherichia coli. J Biol Chem. 1972 Jan 10;247(1):291–297. [PubMed] [Google Scholar]
- Kohn L. D., Kaback H. R. Mechanisms of active transport in isolated bacterial membrane vesicles. XV. Purification and properties of the membrane-bound D-lactate dehydrogenase from Escherichia coli. J Biol Chem. 1973 Oct 25;248(20):7012–7017. [PubMed] [Google Scholar]
- Konings W. N., Barnes E. M., Jr, Kaback H. R. Mechanisms of active transport in isolated membrane vesicles. 2. The coupling of reduced phenazine methosulfate to the concentrative uptake of beta-galactosides and amino acids. J Biol Chem. 1971 Oct 10;246(19):5857–5861. [PubMed] [Google Scholar]
- Konings W. N., Bisschop A., Veenhuis M., Vermeulen C. A. New procedure for the isolation of membrane vesicles of Bacillus subtilis and an electron microscopy study of their ultrastructure. J Bacteriol. 1973 Dec;116(3):1456–1465. doi: 10.1128/jb.116.3.1456-1465.1973. [DOI] [PMC free article] [PubMed] [Google Scholar]
- Lombardi F. J., Kaback H. R. Mechanisms of active transport in isolated bacterial membrane vesicles. 8. The transport of amino acids by membranes prepared from Escherichia coli. J Biol Chem. 1972 Dec 25;247(24):7844–7857. [PubMed] [Google Scholar]
- Lombardi F. J., Reeves J. P., Short S. A., Kaback H. R. Evaluation of the chemiosmotic interpretation of active transport in bacterial membrane vesicles. Ann N Y Acad Sci. 1974 Feb 18;227:312–327. doi: 10.1111/j.1749-6632.1974.tb14396.x. [DOI] [PubMed] [Google Scholar]
- Matin A., Konings W. N. Transport of lactate and succinate by membrane vesicles of Escherichia coli, Bacillus subtilis and a pseudomonas species. Eur J Biochem. 1973 Apr 2;34(1):58–67. doi: 10.1111/j.1432-1033.1973.tb02728.x. [DOI] [PubMed] [Google Scholar]
- Mitchell P. Performance and conservation of osmotic work by proton-coupled solute porter systems. J Bioenerg. 1973 Jan;4(1):63–91. doi: 10.1007/BF01516051. [DOI] [PubMed] [Google Scholar]
- Walsh C. T., Kaback H. R. Membrane transport as a potential target for antibiotic action. Ann N Y Acad Sci. 1974 May 10;235(0):519–541. doi: 10.1111/j.1749-6632.1974.tb43288.x. [DOI] [PubMed] [Google Scholar]
- Walsh C. T., Kaback H. R. Vinylglycolic acid. An inactivator of the phosphoenolpyruvate-phosphate transferase system in Escherichia coli. J Biol Chem. 1973 Aug 10;248(15):5456–5462. [PubMed] [Google Scholar]
- Weiner J. H. The localization of glycerol-3-phosphate dehydrogenase in Escherichia coli. J Membr Biol. 1974;15(1):1–14. doi: 10.1007/BF01870078. [DOI] [PubMed] [Google Scholar]
- van Thienen G., Postma P. W. Coupling between energy conservation and active transport of serine in Escherichia coli. Biochim Biophys Acta. 1973 Oct 25;323(3):429–440. doi: 10.1016/0005-2736(73)90188-0. [DOI] [PubMed] [Google Scholar]