Abstract
The transport mechanism of urate and p-aminohippurate (PAH) was evaluated in microvillus membrane vesicles isolated from the renal cortex of the mongrel dog. Imposition of a transmembrane pH gradient (pHo less than pH1) markedly accelerated the uptake of [14C]urate and [3H]PAH and caused the transient accumulation ("overshoot") of each anion above its final level of uptake. The transport of urate and PAH under both stimulated (pHo less than pHi) and basal (pHo = pHi) conditions was insensitive to valinomycin-induced K+ diffusion potentials. The pH gradient-stimulated uptake of 25 microM [14C]urate and 1.0 microM [3H]PAH was significantly inhibited by 1.2 mM PAH, urate, furosemide, salicylate, or probenecid. The effect of each inhibitor on [14C]urate transport was identical to the effect of the same inhibitor on [3H]PAH flux. We conclude that the transport of urate and PAH in dog renal microvillus membrane vesicles occurs via a pH gradient-stimulated electroneutral carrier-mediated process such as 1:1 H+-anion cotransport or OH-anion exchange. Such a transport mechanism may possibly play a role in effecting uphill urate reabsorption in the proximal tubule. Moreover, this study demonstrates that secondary active solute transport in epithelial membranes may be coupled to the electrochemical gradient of an ion other than Na+.
Full text
PDF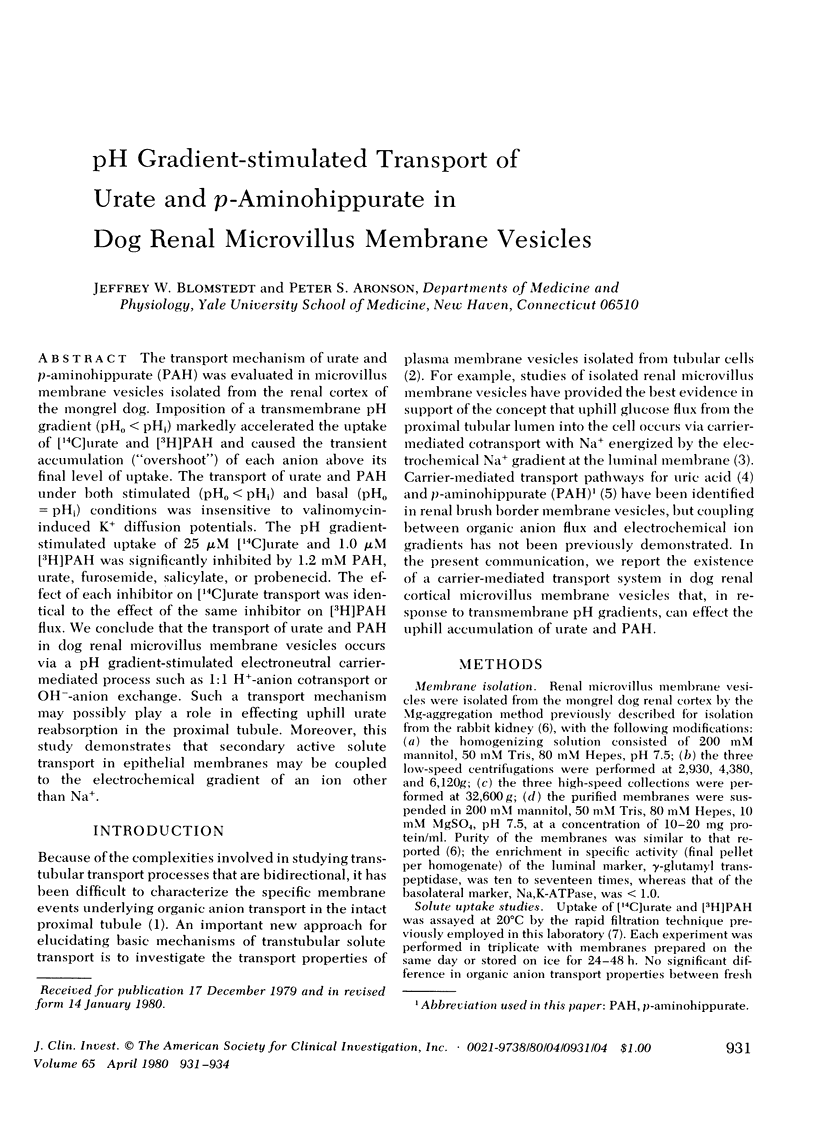
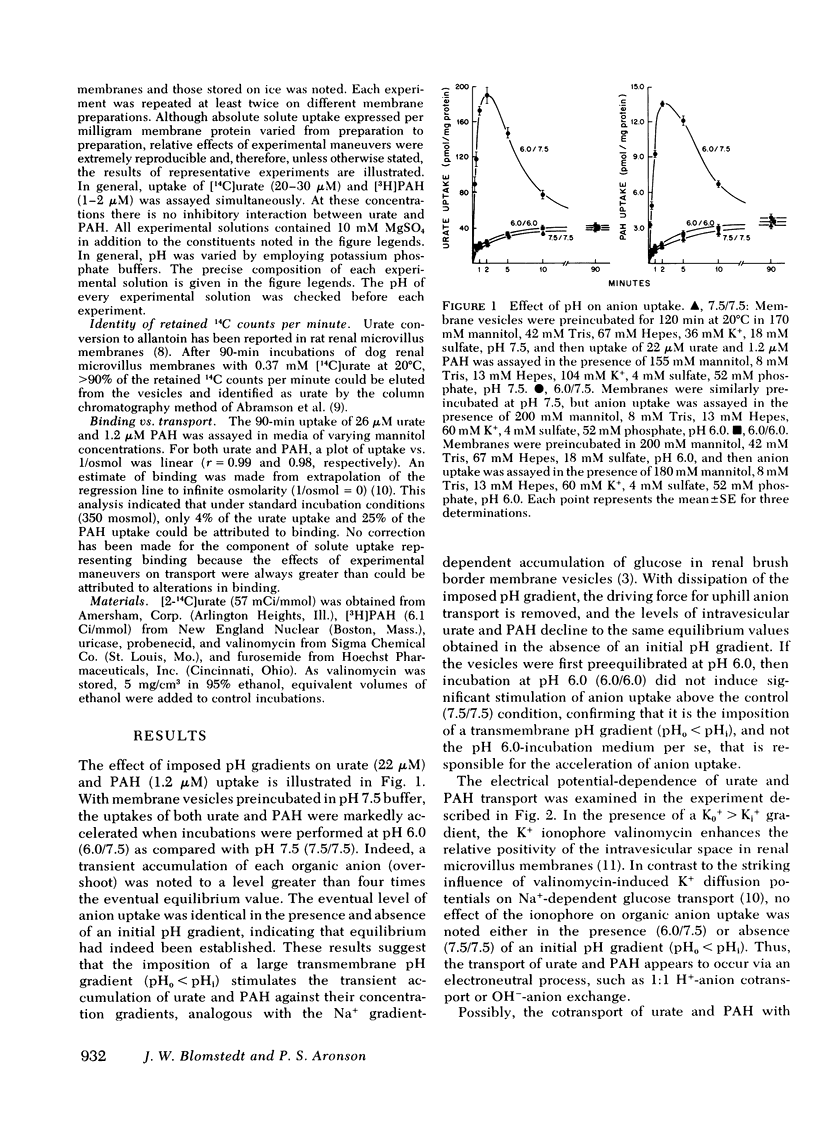
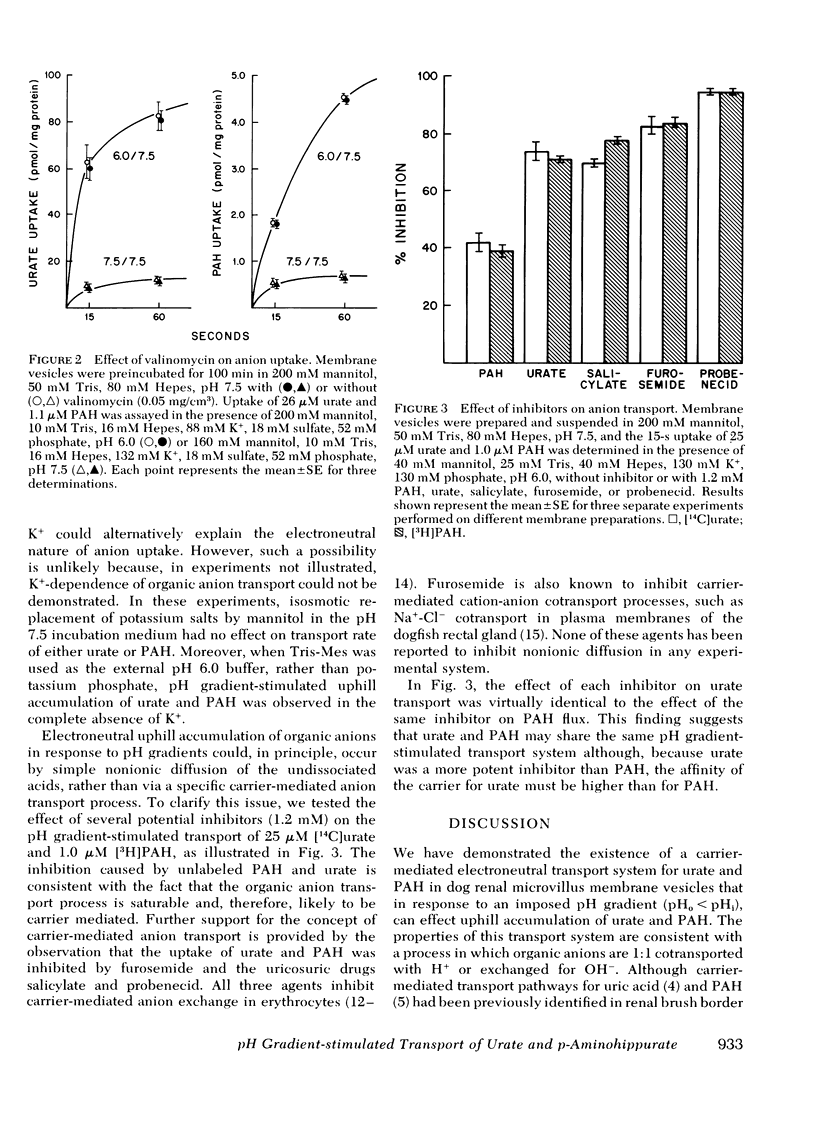
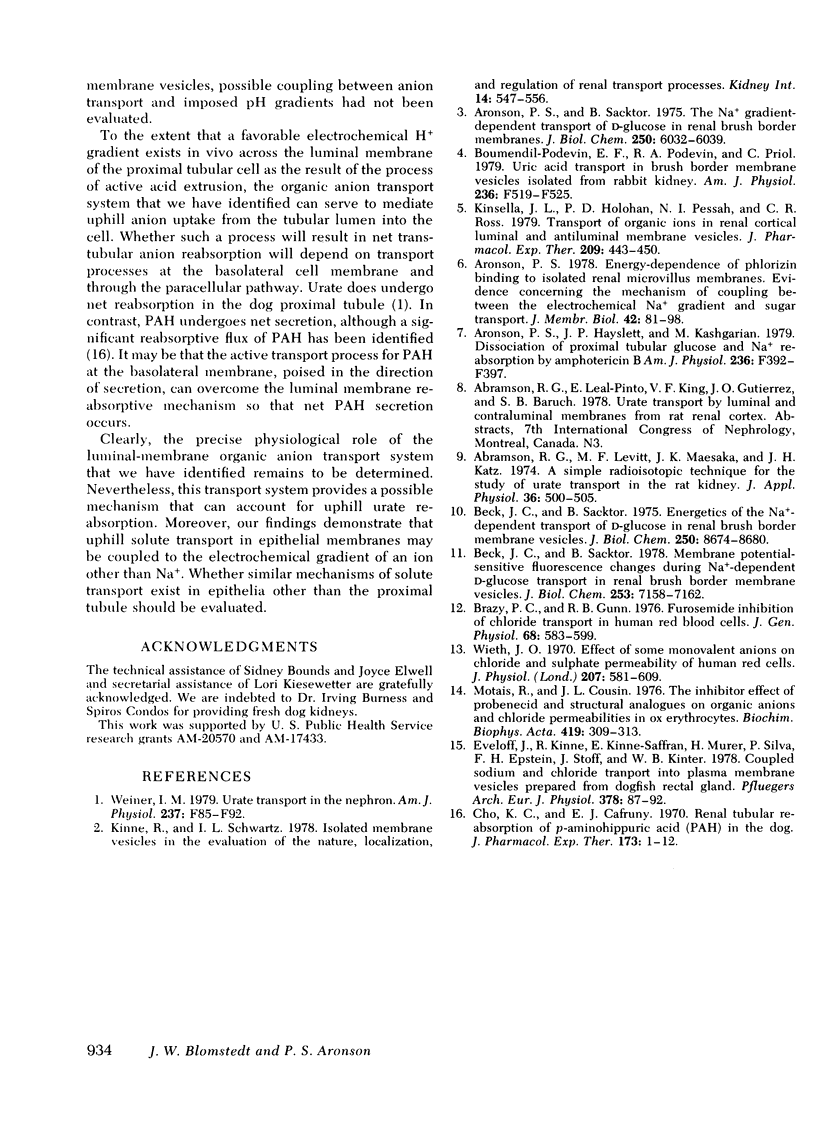
Selected References
These references are in PubMed. This may not be the complete list of references from this article.
- Abramson R. G., Levitt M. F., Maesaka J. K., Katz J. H. A simple radioisotopic technique for the study of urate transport in the rat kidney. J Appl Physiol. 1974 Apr;36(4):500–505. doi: 10.1152/jappl.1974.36.4.500. [DOI] [PubMed] [Google Scholar]
- Aronson P. S. Energy-dependence of phlorizin binding to isolated renal microvillus membranes. Evidence concerning the mechanism of coupling between the electrochemical Na+ gradient the sugar transport. J Membr Biol. 1978 Jul 21;42(1):81–98. doi: 10.1007/BF01870395. [DOI] [PubMed] [Google Scholar]
- Aronson P. S., Hayslett J. P., Kashgarian M. Dissociation of proximal tubular glucose and Na+ reabsorption by amphotericin B. Am J Physiol. 1979 Apr;236(4):F392–F397. doi: 10.1152/ajprenal.1979.236.4.F392. [DOI] [PubMed] [Google Scholar]
- Aronson P. S., Sacktor B. The Na+ gradient-dependent transport of D-glucose in renal brush border membranes. J Biol Chem. 1975 Aug 10;250(15):6032–6039. [PubMed] [Google Scholar]
- Beck J. C., Sacktor B. Energetics of the Na+-dependent transport of D-glucose in renal brush border membrane vesicles. J Biol Chem. 1975 Nov 25;250(22):8674–8680. [PubMed] [Google Scholar]
- Beck J. C., Sacktor B. Membrane potential-sensitive fluorescence changes during Na+-dependent D-glucose transport in renal brush border membrane vesicles. J Biol Chem. 1978 Oct 25;253(20):7158–7162. [PubMed] [Google Scholar]
- Boumendil-Podevin E. F., Podevin R. A., Priol C. Uric acid transport in brush border membrane vesicles isolated from rabbit kidney. Am J Physiol. 1979 Jun;236(6):F519–F525. doi: 10.1152/ajprenal.1979.236.6.F519. [DOI] [PubMed] [Google Scholar]
- Brazy P. C., Gunn R. B. Furosemide inhibition of chloride transport in human red blood cells. J Gen Physiol. 1976 Dec;68(6):583–599. doi: 10.1085/jgp.68.6.583. [DOI] [PMC free article] [PubMed] [Google Scholar]
- Cho K. C., Cafruny E. J. Renal tubular reabsorption of p-aminohippuric acid (PAH)in the dog. J Pharmacol Exp Ther. 1970 May;173(1):1–12. [PubMed] [Google Scholar]
- Eveloff J., Kinne R., Kinne-Saffran E., Murer H., Silva P., Epstein F. H., Stoff J., Kinter W. B. Coupled sodium and chloride transport into plasma membrane vesicles prepared from dogfish rectal gland. Pflugers Arch. 1978 Dec 28;378(2):87–92. doi: 10.1007/BF00584439. [DOI] [PubMed] [Google Scholar]
- Kinne R., Schwartz I. L. Isolated membrane vesicles in the evaluation of the nature, localization, and regulation of renal transport processes. Kidney Int. 1978 Dec;14(6):547–556. doi: 10.1038/ki.1978.163. [DOI] [PubMed] [Google Scholar]
- Kinsella J. L., Holohan P. D., Pessah N. I., Ross C. R. Transport of organic ions in renal cortical luminal and antiluminal membrane vesicles. J Pharmacol Exp Ther. 1979 Jun;209(3):443–450. [PubMed] [Google Scholar]
- Motais R., Cousin J. L. The inhibitor effect of probencid and structural analogues on organic anions and chloride permeabilities in ox erythrocytes. Biochim Biophys Acta. 1976 Jan 21;419(2):309–313. doi: 10.1016/0005-2736(76)90356-4. [DOI] [PubMed] [Google Scholar]
- Wieth J. O. Effect of some monovalent anions on chloride and sulphate permeability of human red cells. J Physiol. 1970 May;207(3):581–609. doi: 10.1113/jphysiol.1970.sp009082. [DOI] [PMC free article] [PubMed] [Google Scholar]