Abstract
The neural plate in the amphibian embryo is induced in the ectoderm by signals from the dorsal mesoderm. In the extensively studied species Xenopus laevis, such signals are believed to proceed along two alternate pathways, defined as vertical and planar induction. We have studied the relative importance of these pathways in Rana pipiens. In the embryo of this frog, dorsal mesoderm involution can be diverted from its normal course by injection of peptides that inhibit interaction of fibronectin with its receptor. In such embryos, dorsal mesoderm failed to migrate across the blastocoel roof but moved bilaterally along the equator, leading to the formation of two notochords. Neural tissue differentiation occurred in close association with each notochord, but no neural tissue formed along the dorsal midline as might have been predicted by a predominantly planar induction model. While in X. laevis planar induction has been reported to be a major pathway in neuralizing the ecoderm, the results presented here indicate that vertical induction predominates in initiating neural development in R. pipiens embryos.
Full text
PDF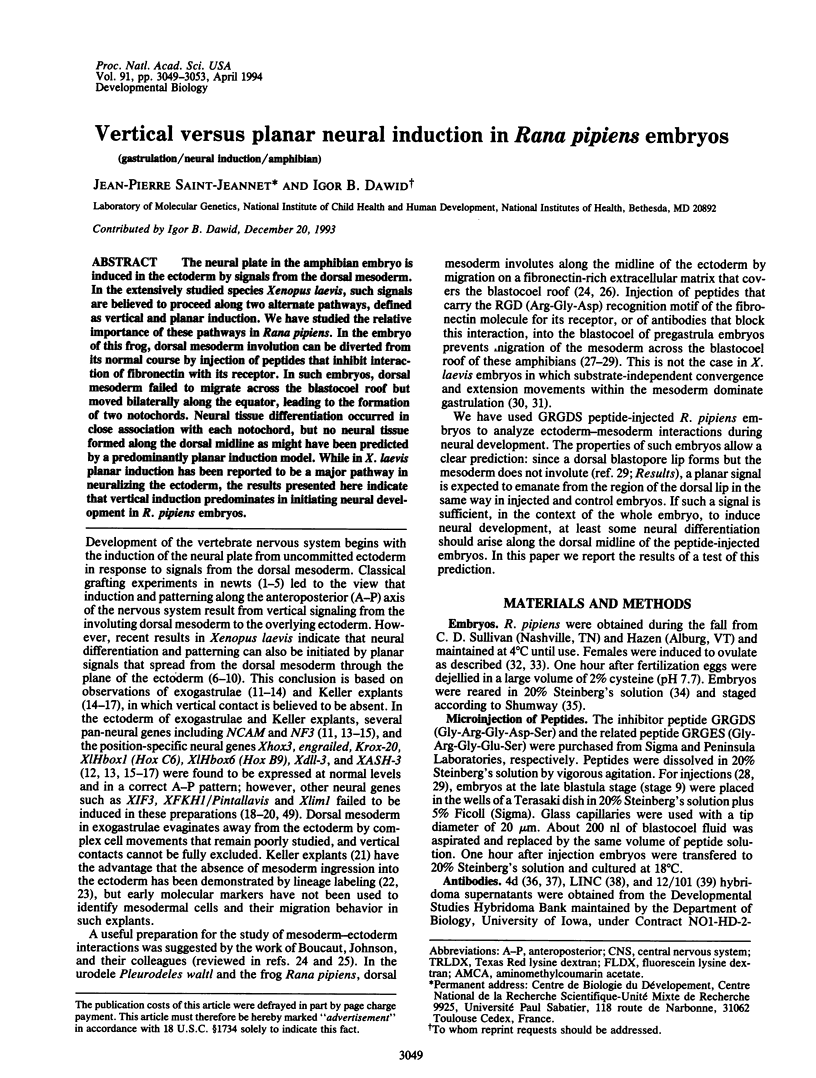
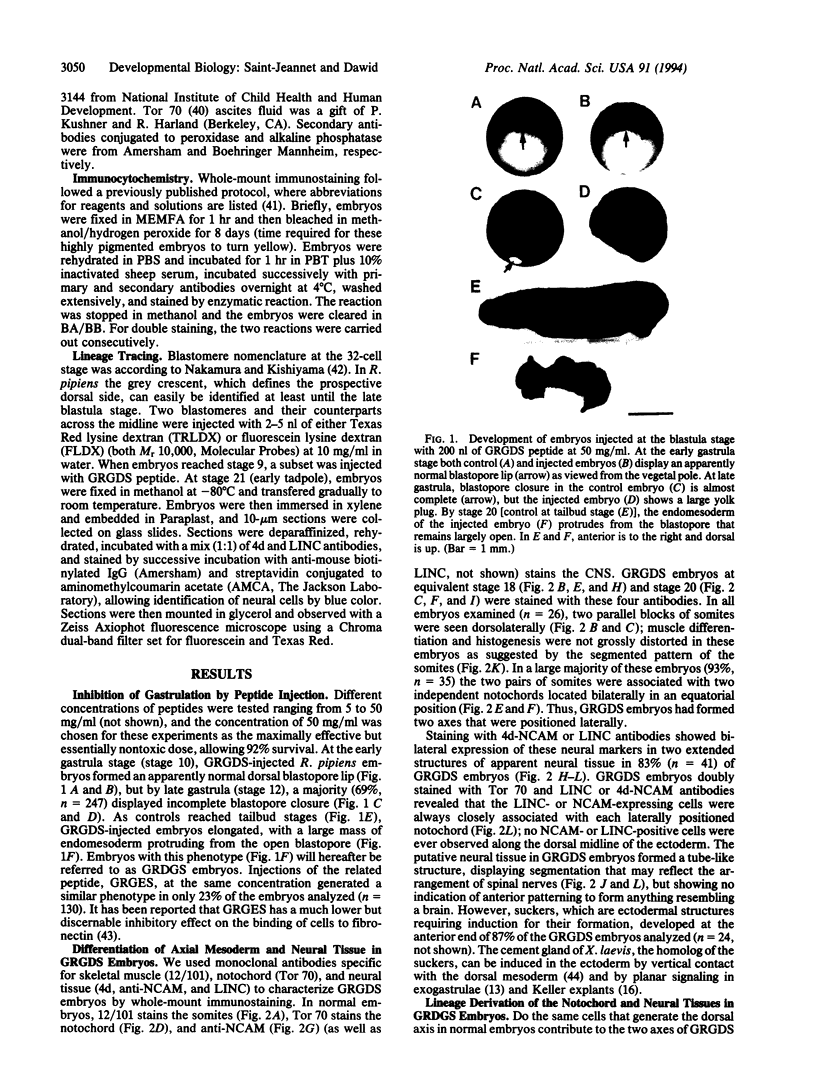
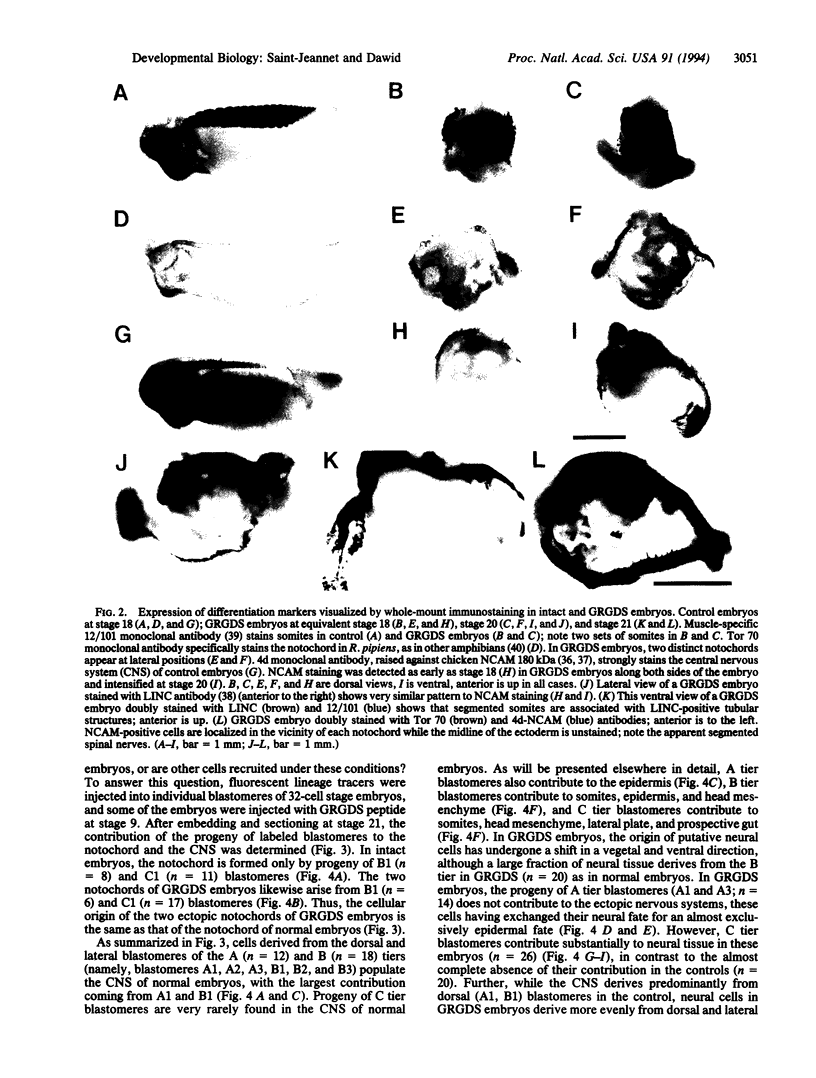
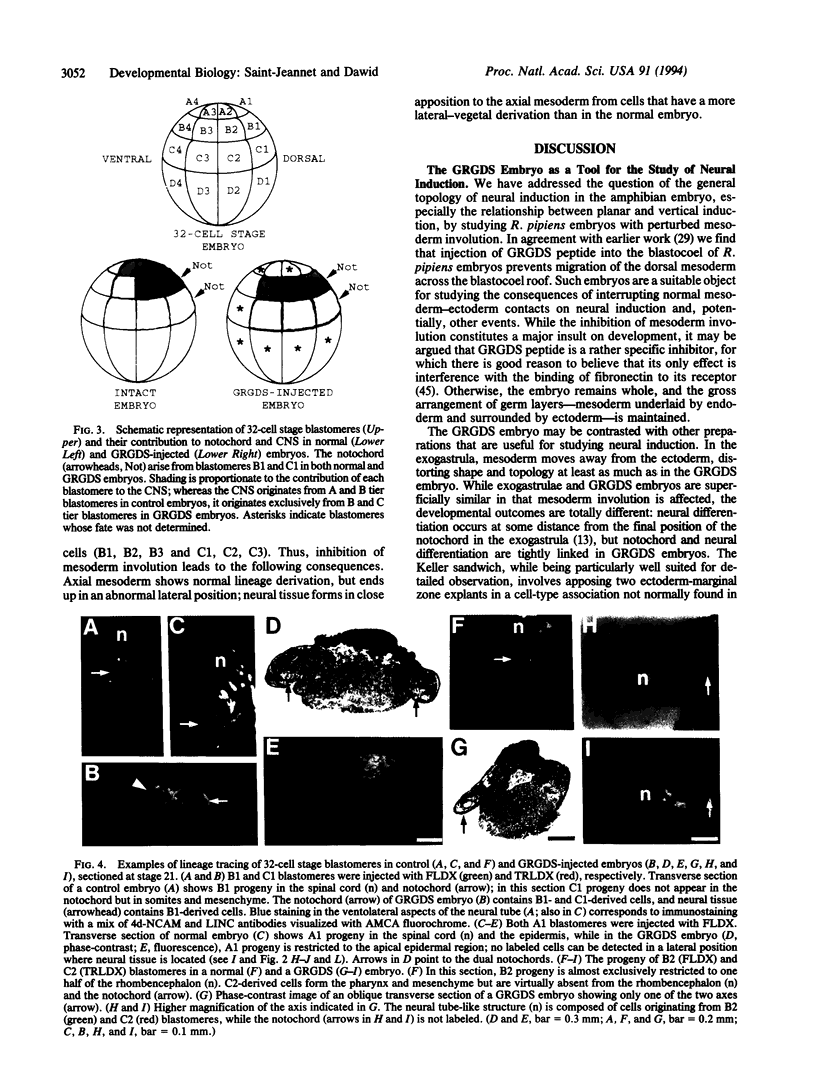
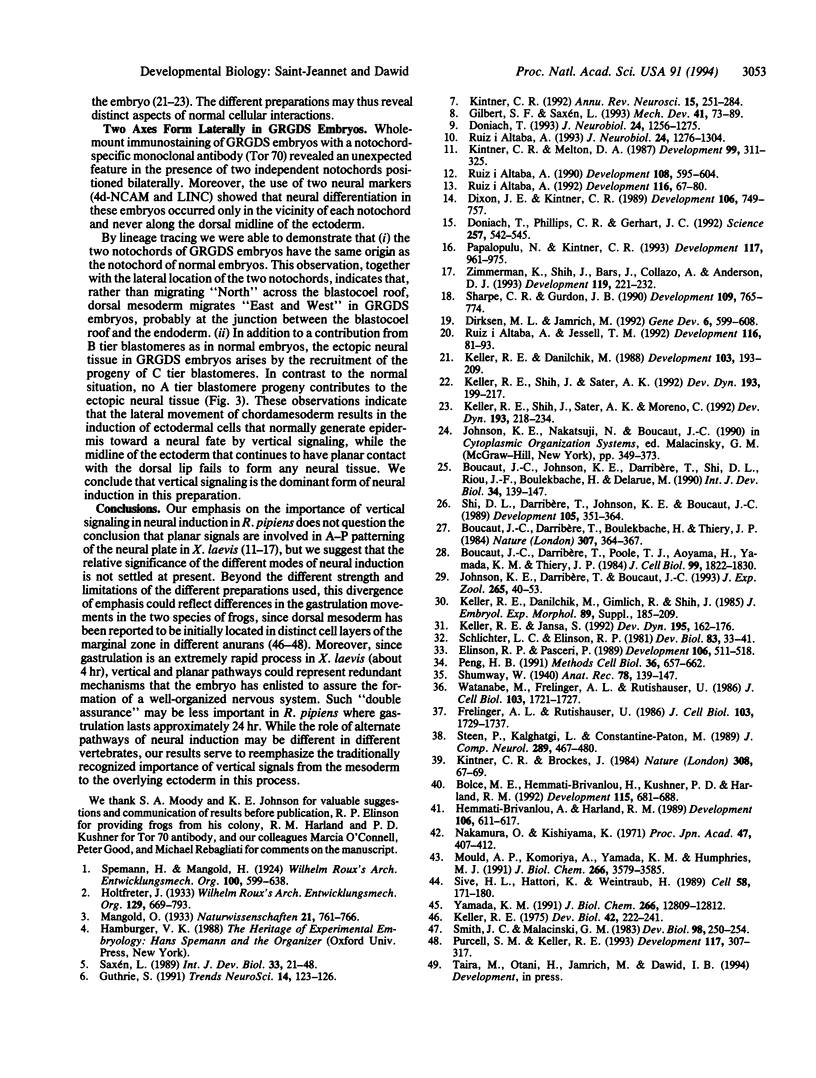
Images in this article
Selected References
These references are in PubMed. This may not be the complete list of references from this article.
- Bolce M. E., Hemmati-Brivanlou A., Kushner P. D., Harland R. M. Ventral ectoderm of Xenopus forms neural tissue, including hindbrain, in response to activin. Development. 1992 Jul;115(3):681–688. doi: 10.1242/dev.115.3.681. [DOI] [PubMed] [Google Scholar]
- Boucaut J. C., Darribère T., Boulekbache H., Thiery J. P. Prevention of gastrulation but not neurulation by antibodies to fibronectin in amphibian embryos. 1984 Jan 26-Feb 1Nature. 307(5949):364–367. doi: 10.1038/307364a0. [DOI] [PubMed] [Google Scholar]
- Boucaut J. C., Darribère T., Poole T. J., Aoyama H., Yamada K. M., Thiery J. P. Biologically active synthetic peptides as probes of embryonic development: a competitive peptide inhibitor of fibronectin function inhibits gastrulation in amphibian embryos and neural crest cell migration in avian embryos. J Cell Biol. 1984 Nov;99(5):1822–1830. doi: 10.1083/jcb.99.5.1822. [DOI] [PMC free article] [PubMed] [Google Scholar]
- Boucaut J. C., Johnson K. E., Darribère T., Shi D. L., Riou J. F., Bache H. B., Delarue M. Fibronectin-rich fibrillar extracellular matrix controls cell migration during amphibian gastrulation. Int J Dev Biol. 1990 Mar;34(1):139–147. [PubMed] [Google Scholar]
- Brivanlou A. H., Harland R. M. Expression of an engrailed-related protein is induced in the anterior neural ectoderm of early Xenopus embryos. Development. 1989 Jul;106(3):611–617. doi: 10.1242/dev.106.3.611. [DOI] [PubMed] [Google Scholar]
- Dirksen M. L., Jamrich M. A novel, activin-inducible, blastopore lip-specific gene of Xenopus laevis contains a fork head DNA-binding domain. Genes Dev. 1992 Apr;6(4):599–608. doi: 10.1101/gad.6.4.599. [DOI] [PubMed] [Google Scholar]
- Dixon J. E., Kintner C. R. Cellular contacts required for neural induction in Xenopus embryos: evidence for two signals. Development. 1989 Aug;106(4):749–757. doi: 10.1242/dev.106.4.749. [DOI] [PubMed] [Google Scholar]
- Doniach T., Phillips C. R., Gerhart J. C. Planar induction of anteroposterior pattern in the developing central nervous system of Xenopus laevis. Science. 1992 Jul 24;257(5069):542–545. doi: 10.1126/science.1636091. [DOI] [PubMed] [Google Scholar]
- Doniach T. Planar and vertical induction of anteroposterior pattern during the development of the amphibian central nervous system. J Neurobiol. 1993 Oct;24(10):1256–1275. doi: 10.1002/neu.480241003. [DOI] [PubMed] [Google Scholar]
- Elinson R. P., Pasceri P. Two UV-sensitive targets in dorsoanterior specification of frog embryos. Development. 1989 Jul;106(3):511–518. doi: 10.1242/dev.106.3.511. [DOI] [PubMed] [Google Scholar]
- Frelinger A. L., 3rd, Rutishauser U. Topography of N-CAM structural and functional determinants. II. Placement of monoclonal antibody epitopes. J Cell Biol. 1986 Nov;103(5):1729–1737. doi: 10.1083/jcb.103.5.1729. [DOI] [PMC free article] [PubMed] [Google Scholar]
- Gilbert S. F., Saxén L. Spemann's organizer: models and molecules. Mech Dev. 1993 May;41(2-3):73–89. doi: 10.1016/0925-4773(93)90039-z. [DOI] [PubMed] [Google Scholar]
- Guthrie S. Horizontal and vertical pathways in neural induction. Trends Neurosci. 1991 Apr;14(4):123–126. doi: 10.1016/0166-2236(91)90077-8. [DOI] [PubMed] [Google Scholar]
- Johnson K. E., Darribère T., Boucaut J. C. Mesodermal cell adhesion to fibronectin-rich fibrillar extracellular matrix is required for normal Rana pipiens gastrulation. J Exp Zool. 1993 Jan 1;265(1):40–53. doi: 10.1002/jez.1402650107. [DOI] [PubMed] [Google Scholar]
- Keller R. E., Danilchik M., Gimlich R., Shih J. The function and mechanism of convergent extension during gastrulation of Xenopus laevis. J Embryol Exp Morphol. 1985 Nov;89 (Suppl):185–209. [PubMed] [Google Scholar]
- Keller R. E. Vital dye mapping of the gastrula and neurula of Xenopus laevis. I. Prospective areas and morphogenetic movements of the superficial layer. Dev Biol. 1975 Feb;42(2):222–241. doi: 10.1016/0012-1606(75)90331-0. [DOI] [PubMed] [Google Scholar]
- Keller R., Danilchik M. Regional expression, pattern and timing of convergence and extension during gastrulation of Xenopus laevis. Development. 1988 May;103(1):193–209. doi: 10.1242/dev.103.1.193. [DOI] [PubMed] [Google Scholar]
- Keller R., Jansa S. Xenopus Gastrulation without a blastocoel roof. Dev Dyn. 1992 Nov;195(3):162–176. doi: 10.1002/aja.1001950303. [DOI] [PubMed] [Google Scholar]
- Keller R., Shih J., Sater A. K., Moreno C. Planar induction of convergence and extension of the neural plate by the organizer of Xenopus. Dev Dyn. 1992 Mar;193(3):218–234. doi: 10.1002/aja.1001930303. [DOI] [PubMed] [Google Scholar]
- Keller R., Shih J., Sater A. The cellular basis of the convergence and extension of the Xenopus neural plate. Dev Dyn. 1992 Mar;193(3):199–217. doi: 10.1002/aja.1001930302. [DOI] [PubMed] [Google Scholar]
- Kintner C. R., Brockes J. P. Monoclonal antibodies identify blastemal cells derived from dedifferentiating limb regeneration. Nature. 1984 Mar 1;308(5954):67–69. doi: 10.1038/308067a0. [DOI] [PubMed] [Google Scholar]
- Kintner C. R., Melton D. A. Expression of Xenopus N-CAM RNA in ectoderm is an early response to neural induction. Development. 1987 Mar;99(3):311–325. doi: 10.1242/dev.99.3.311. [DOI] [PubMed] [Google Scholar]
- Kintner C. Molecular bases of early neural development in Xenopus embryos. Annu Rev Neurosci. 1992;15:251–284. doi: 10.1146/annurev.ne.15.030192.001343. [DOI] [PubMed] [Google Scholar]
- Mould A. P., Komoriya A., Yamada K. M., Humphries M. J. The CS5 peptide is a second site in the IIICS region of fibronectin recognized by the integrin alpha 4 beta 1. Inhibition of alpha 4 beta 1 function by RGD peptide homologues. J Biol Chem. 1991 Feb 25;266(6):3579–3585. [PubMed] [Google Scholar]
- Papalopulu N., Kintner C. Xenopus Distal-less related homeobox genes are expressed in the developing forebrain and are induced by planar signals. Development. 1993 Mar;117(3):961–975. doi: 10.1242/dev.117.3.961. [DOI] [PubMed] [Google Scholar]
- Peng H. B. Xenopus laevis: Practical uses in cell and molecular biology. Solutions and protocols. Methods Cell Biol. 1991;36:657–662. [PubMed] [Google Scholar]
- Purcell S. M., Keller R. A different type of amphibian mesoderm morphogenesis in Ceratophrys ornata. Development. 1993 Jan;117(1):307–317. doi: 10.1242/dev.117.1.307. [DOI] [PubMed] [Google Scholar]
- Ruiz i Altaba A. Induction and axial patterning of the neural plate: planar and vertical signals. J Neurobiol. 1993 Oct;24(10):1276–1304. doi: 10.1002/neu.480241004. [DOI] [PubMed] [Google Scholar]
- Ruiz i Altaba A., Jessell T. M. Pintallavis, a gene expressed in the organizer and midline cells of frog embryos: involvement in the development of the neural axis. Development. 1992 Sep;116(1):81–93. doi: 10.1242/dev.116.Supplement.81. [DOI] [PubMed] [Google Scholar]
- Ruiz i Altaba A. Neural expression of the Xenopus homeobox gene Xhox3: evidence for a patterning neural signal that spreads through the ectoderm. Development. 1990 Apr;108(4):595–604. doi: 10.1242/dev.108.4.595. [DOI] [PubMed] [Google Scholar]
- Ruiz i Altaba A. Planar and vertical signals in the induction and patterning of the Xenopus nervous system. Development. 1992 Sep;116(1):67–80. doi: 10.1242/dev.116.1.67. [DOI] [PubMed] [Google Scholar]
- Schlichter L. C., Elinson R. P. Electrical responses of immature and mature Rana pipiens oocytes to sperm and other activating stimuli. Dev Biol. 1981 Apr 15;83(1):33–41. doi: 10.1016/s0012-1606(81)80005-x. [DOI] [PubMed] [Google Scholar]
- Sharpe C. R., Gurdon J. B. The induction of anterior and posterior neural genes in Xenopus laevis. Development. 1990 Aug;109(4):765–774. doi: 10.1242/dev.109.4.765. [DOI] [PubMed] [Google Scholar]
- Sive H. L., Hattori K., Weintraub H. Progressive determination during formation of the anteroposterior axis in Xenopus laevis. Cell. 1989 Jul 14;58(1):171–180. doi: 10.1016/0092-8674(89)90413-3. [DOI] [PubMed] [Google Scholar]
- Smith J. C., Malacinski G. M. The origin of the mesoderm in an anuran, Xenopus laevis, and a urodele, Ambystoma mexicanum. Dev Biol. 1983 Jul;98(1):250–254. doi: 10.1016/0012-1606(83)90354-8. [DOI] [PubMed] [Google Scholar]
- Steen P., Kalghatgi L., Constantine-Paton M. Monoclonal antibody markers for amphibian oligodendrocytes and neurons. J Comp Neurol. 1989 Nov 15;289(3):467–480. doi: 10.1002/cne.902890311. [DOI] [PubMed] [Google Scholar]
- Watanabe M., Frelinger A. L., 3rd, Rutishauser U. Topography of N-CAM structural and functional determinants. I. Classification of monoclonal antibody epitopes. J Cell Biol. 1986 Nov;103(5):1721–1727. doi: 10.1083/jcb.103.5.1721. [DOI] [PMC free article] [PubMed] [Google Scholar]
- Yamada K. M. Adhesive recognition sequences. J Biol Chem. 1991 Jul 15;266(20):12809–12812. [PubMed] [Google Scholar]
- Zimmerman K., Shih J., Bars J., Collazo A., Anderson D. J. XASH-3, a novel Xenopus achaete-scute homolog, provides an early marker of planar neural induction and position along the mediolateral axis of the neural plate. Development. 1993 Sep;119(1):221–232. doi: 10.1242/dev.119.1.221. [DOI] [PubMed] [Google Scholar]