Abstract
The elastic protein titin comprises a tandem array of fibronectin type III and immunoglobulin domains, which are structurally similar 7-strand beta-sandwiches. A proposed mechanism for stretching titin, by sequential denaturation of individual fibronectin type III-immunoglobulin domains in response to applied tension, is analyzed here quantitatively. The folded domain is approximately 4 nm long, and the unraveled polypeptide can extend to 29 nm, providing a 7-fold stretch over the relaxed length. Elastic recoil is achieved by refolding of the denatured domains when the force is released. The critical force required to denature a domain is calculated to be 3.5-5 pN, based on a net free energy for denaturation of 7-14 kcal/mol, plus 5 kcal/mol to extend the polypeptide (1 cal = 4.184 J). This force is comparable to the 2- to 7-pN force generated by single myosin or kinesin molecules. The force needed to pull apart a noncovalent protein-protein interface is estimated here to be 10-30 pN, implying that titin will stretch internally before the molecule is pulled from its attachment at the Z band. Many extracellular matrix and cell adhesion molecules, such as fibronectin, contain tandem arrays of fibronectin type III domains. Both single molecules and matrix fibers should have elastic properties similar to titin.
Full text
PDF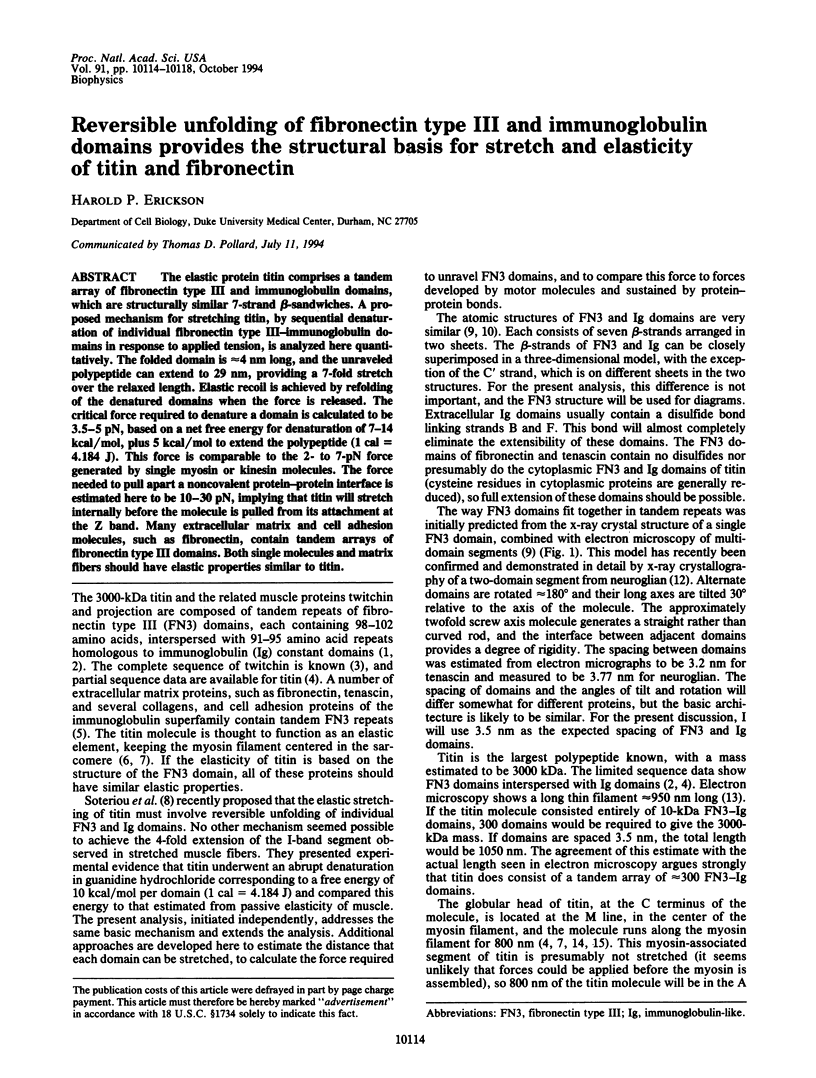
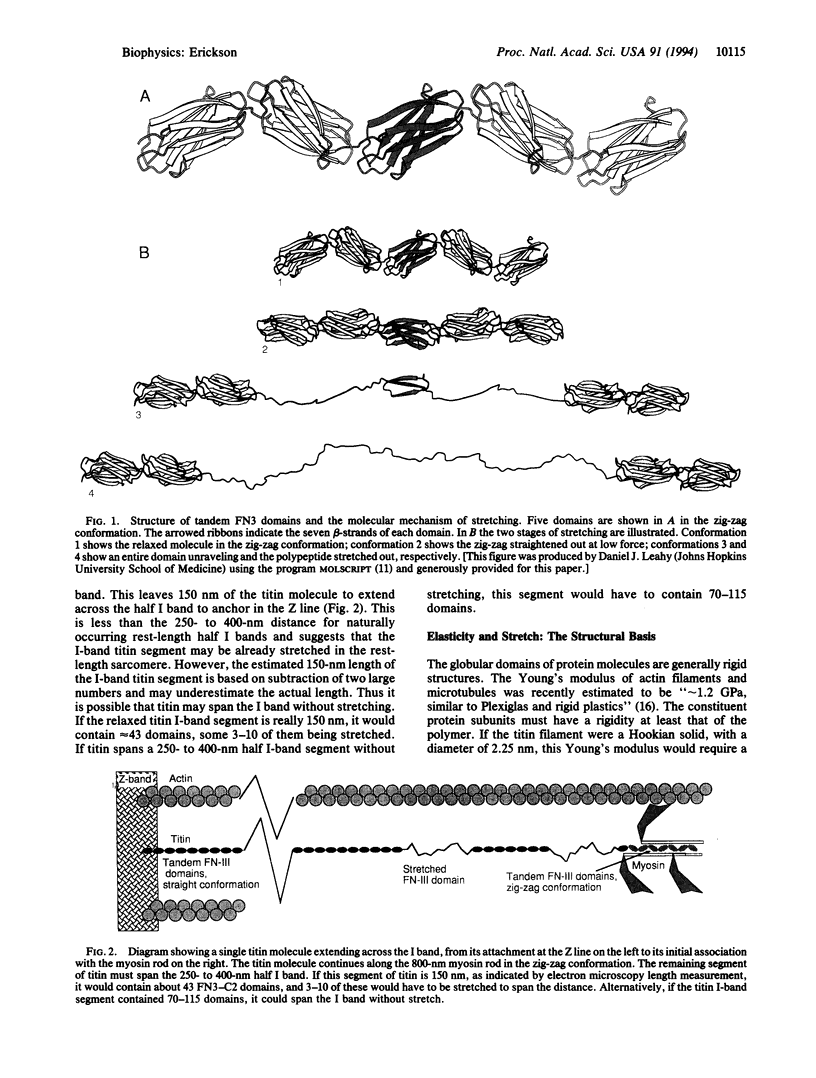
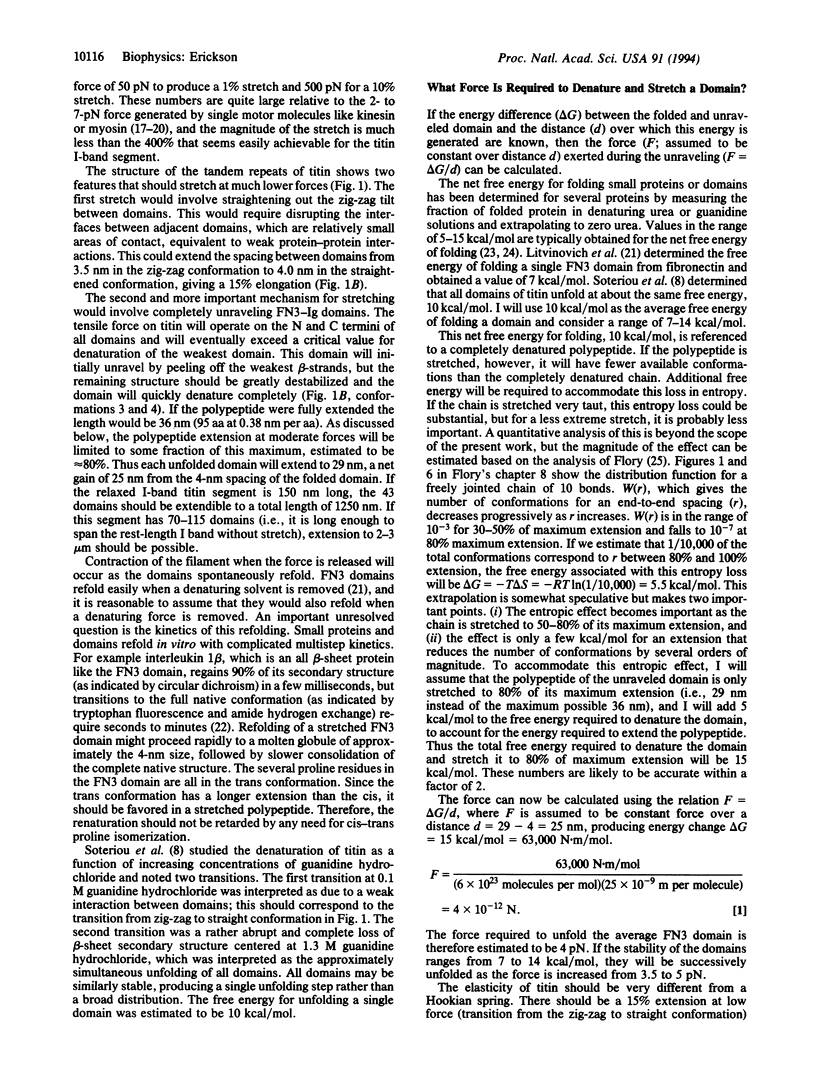
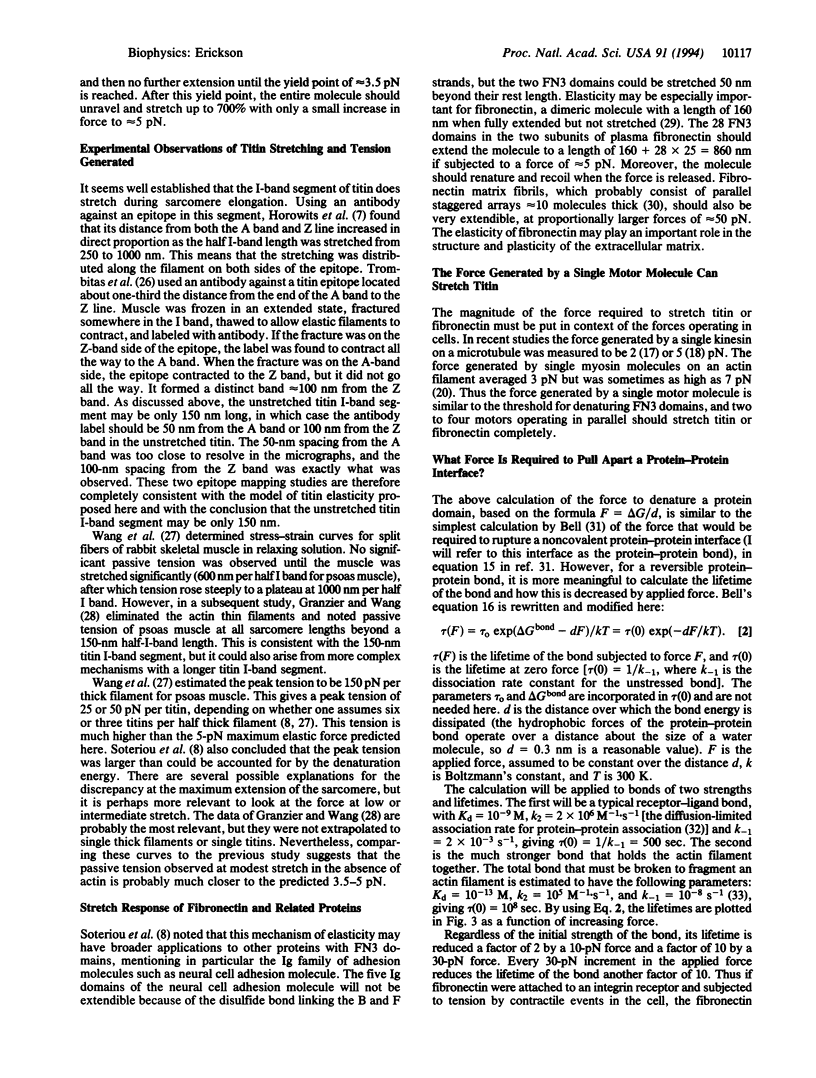
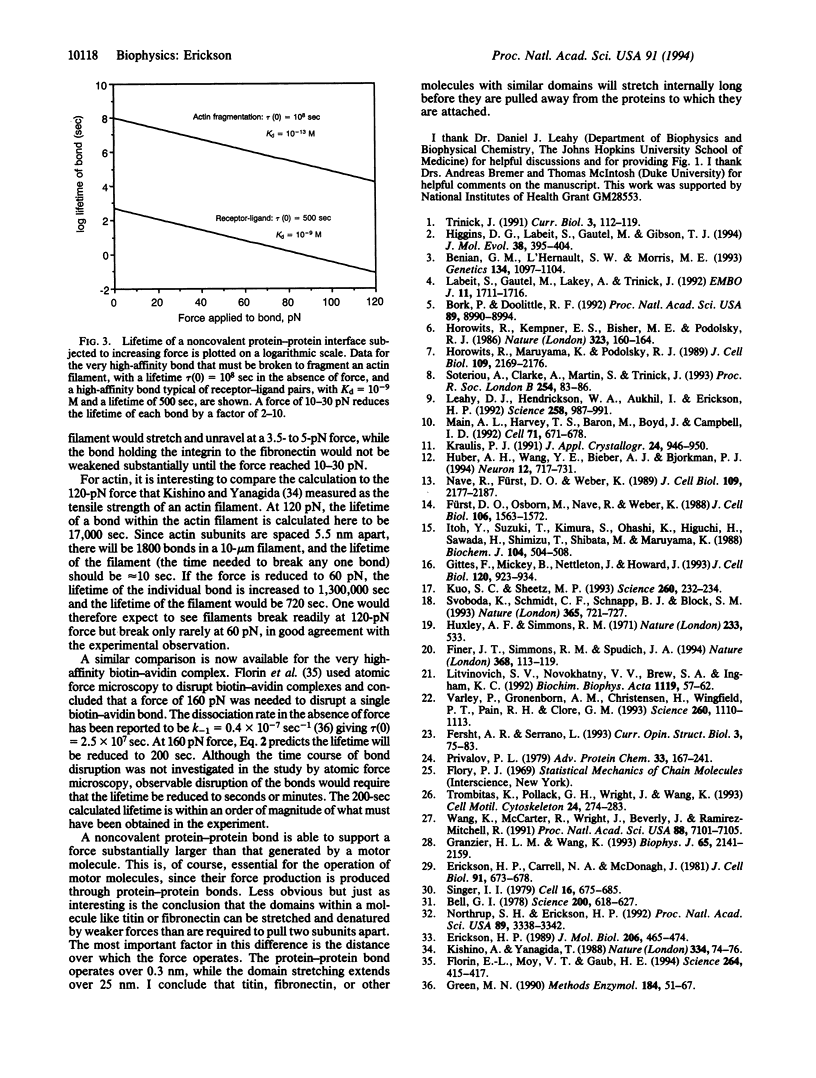
Images in this article
Selected References
These references are in PubMed. This may not be the complete list of references from this article.
- Bell G. I. Models for the specific adhesion of cells to cells. Science. 1978 May 12;200(4342):618–627. doi: 10.1126/science.347575. [DOI] [PubMed] [Google Scholar]
- Benian G. M., L'Hernault S. W., Morris M. E. Additional sequence complexity in the muscle gene, unc-22, and its encoded protein, twitchin, of Caenorhabditis elegans. Genetics. 1993 Aug;134(4):1097–1104. doi: 10.1093/genetics/134.4.1097. [DOI] [PMC free article] [PubMed] [Google Scholar]
- Bork P., Doolittle R. F. Proposed acquisition of an animal protein domain by bacteria. Proc Natl Acad Sci U S A. 1992 Oct 1;89(19):8990–8994. doi: 10.1073/pnas.89.19.8990. [DOI] [PMC free article] [PubMed] [Google Scholar]
- Erickson H. P., Carrell N., McDonagh J. Fibronectin molecule visualized in electron microscopy: a long, thin, flexible strand. J Cell Biol. 1981 Dec;91(3 Pt 1):673–678. doi: 10.1083/jcb.91.3.673. [DOI] [PMC free article] [PubMed] [Google Scholar]
- Erickson H. P. Co-operativity in protein-protein association. The structure and stability of the actin filament. J Mol Biol. 1989 Apr 5;206(3):465–474. doi: 10.1016/0022-2836(89)90494-4. [DOI] [PubMed] [Google Scholar]
- Finer J. T., Simmons R. M., Spudich J. A. Single myosin molecule mechanics: piconewton forces and nanometre steps. Nature. 1994 Mar 10;368(6467):113–119. doi: 10.1038/368113a0. [DOI] [PubMed] [Google Scholar]
- Florin E. L., Moy V. T., Gaub H. E. Adhesion forces between individual ligand-receptor pairs. Science. 1994 Apr 15;264(5157):415–417. doi: 10.1126/science.8153628. [DOI] [PubMed] [Google Scholar]
- Fürst D. O., Osborn M., Nave R., Weber K. The organization of titin filaments in the half-sarcomere revealed by monoclonal antibodies in immunoelectron microscopy: a map of ten nonrepetitive epitopes starting at the Z line extends close to the M line. J Cell Biol. 1988 May;106(5):1563–1572. doi: 10.1083/jcb.106.5.1563. [DOI] [PMC free article] [PubMed] [Google Scholar]
- Gittes F., Mickey B., Nettleton J., Howard J. Flexural rigidity of microtubules and actin filaments measured from thermal fluctuations in shape. J Cell Biol. 1993 Feb;120(4):923–934. doi: 10.1083/jcb.120.4.923. [DOI] [PMC free article] [PubMed] [Google Scholar]
- Granzier H. L., Wang K. Passive tension and stiffness of vertebrate skeletal and insect flight muscles: the contribution of weak cross-bridges and elastic filaments. Biophys J. 1993 Nov;65(5):2141–2159. doi: 10.1016/S0006-3495(93)81262-1. [DOI] [PMC free article] [PubMed] [Google Scholar]
- Green N. M. Avidin and streptavidin. Methods Enzymol. 1990;184:51–67. doi: 10.1016/0076-6879(90)84259-j. [DOI] [PubMed] [Google Scholar]
- Higgins D. G., Labeit S., Gautel M., Gibson T. J. The evolution of titin and related giant muscle proteins. J Mol Evol. 1994 Apr;38(4):395–404. doi: 10.1007/BF00163156. [DOI] [PubMed] [Google Scholar]
- Horowits R., Kempner E. S., Bisher M. E., Podolsky R. J. A physiological role for titin and nebulin in skeletal muscle. Nature. 1986 Sep 11;323(6084):160–164. doi: 10.1038/323160a0. [DOI] [PubMed] [Google Scholar]
- Horowits R., Maruyama K., Podolsky R. J. Elastic behavior of connectin filaments during thick filament movement in activated skeletal muscle. J Cell Biol. 1989 Nov;109(5):2169–2176. doi: 10.1083/jcb.109.5.2169. [DOI] [PMC free article] [PubMed] [Google Scholar]
- Huber A. H., Wang Y. M., Bieber A. J., Bjorkman P. J. Crystal structure of tandem type III fibronectin domains from Drosophila neuroglian at 2.0 A. Neuron. 1994 Apr;12(4):717–731. doi: 10.1016/0896-6273(94)90326-3. [DOI] [PubMed] [Google Scholar]
- Itoh Y., Suzuki T., Kimura S., Ohashi K., Higuchi H., Sawada H., Shimizu T., Shibata M., Maruyama K. Extensible and less-extensible domains of connectin filaments in stretched vertebrate skeletal muscle sarcomeres as detected by immunofluorescence and immunoelectron microscopy using monoclonal antibodies. J Biochem. 1988 Oct;104(4):504–508. doi: 10.1093/oxfordjournals.jbchem.a122499. [DOI] [PubMed] [Google Scholar]
- Kishino A., Yanagida T. Force measurements by micromanipulation of a single actin filament by glass needles. Nature. 1988 Jul 7;334(6177):74–76. doi: 10.1038/334074a0. [DOI] [PubMed] [Google Scholar]
- Labeit S., Gautel M., Lakey A., Trinick J. Towards a molecular understanding of titin. EMBO J. 1992 May;11(5):1711–1716. doi: 10.1002/j.1460-2075.1992.tb05222.x. [DOI] [PMC free article] [PubMed] [Google Scholar]
- Leahy D. J., Hendrickson W. A., Aukhil I., Erickson H. P. Structure of a fibronectin type III domain from tenascin phased by MAD analysis of the selenomethionyl protein. Science. 1992 Nov 6;258(5084):987–991. doi: 10.1126/science.1279805. [DOI] [PubMed] [Google Scholar]
- Litvinovich S. V., Novokhatny V. V., Brew S. A., Ingham K. C. Reversible unfolding of an isolated heparin and DNA binding fragment, the first type III module from fibronectin. Biochim Biophys Acta. 1992 Feb 13;1119(1):57–62. doi: 10.1016/0167-4838(92)90234-5. [DOI] [PubMed] [Google Scholar]
- Main A. L., Harvey T. S., Baron M., Boyd J., Campbell I. D. The three-dimensional structure of the tenth type III module of fibronectin: an insight into RGD-mediated interactions. Cell. 1992 Nov 13;71(4):671–678. doi: 10.1016/0092-8674(92)90600-h. [DOI] [PubMed] [Google Scholar]
- Nave R., Fürst D. O., Weber K. Visualization of the polarity of isolated titin molecules: a single globular head on a long thin rod as the M band anchoring domain? J Cell Biol. 1989 Nov;109(5):2177–2187. doi: 10.1083/jcb.109.5.2177. [DOI] [PMC free article] [PubMed] [Google Scholar]
- Northrup S. H., Erickson H. P. Kinetics of protein-protein association explained by Brownian dynamics computer simulation. Proc Natl Acad Sci U S A. 1992 Apr 15;89(8):3338–3342. doi: 10.1073/pnas.89.8.3338. [DOI] [PMC free article] [PubMed] [Google Scholar]
- Privalov P. L. Stability of proteins: small globular proteins. Adv Protein Chem. 1979;33:167–241. doi: 10.1016/s0065-3233(08)60460-x. [DOI] [PubMed] [Google Scholar]
- Singer I. I. The fibronexus: a transmembrane association of fibronectin-containing fibers and bundles of 5 nm microfilaments in hamster and human fibroblasts. Cell. 1979 Mar;16(3):675–685. doi: 10.1016/0092-8674(79)90040-0. [DOI] [PubMed] [Google Scholar]
- Soteriou A., Clarke A., Martin S., Trinick J. Titin folding energy and elasticity. Proc Biol Sci. 1993 Nov 22;254(1340):83–86. doi: 10.1098/rspb.1993.0130. [DOI] [PubMed] [Google Scholar]
- Svoboda K., Schmidt C. F., Schnapp B. J., Block S. M. Direct observation of kinesin stepping by optical trapping interferometry. Nature. 1993 Oct 21;365(6448):721–727. doi: 10.1038/365721a0. [DOI] [PubMed] [Google Scholar]
- Trinick J. Elastic filaments and giant proteins in muscle. Curr Opin Cell Biol. 1991 Feb;3(1):112–119. doi: 10.1016/0955-0674(91)90173-v. [DOI] [PubMed] [Google Scholar]
- Trombitás K., Pollack G. H., Wright J., Wang K. Elastic properties of titin filaments demonstrated using a "freeze-break" technique. Cell Motil Cytoskeleton. 1993;24(4):274–283. doi: 10.1002/cm.970240408. [DOI] [PubMed] [Google Scholar]
- Varley P., Gronenborn A. M., Christensen H., Wingfield P. T., Pain R. H., Clore G. M. Kinetics of folding of the all-beta sheet protein interleukin-1 beta. Science. 1993 May 21;260(5111):1110–1113. doi: 10.1126/science.8493553. [DOI] [PubMed] [Google Scholar]