Abstract
Using carboxypeptidase Y in Saccharomyces cerevisiae as a model system, the in vivo relationship between protein folding and N-glycosylation was studied. Seven new sites for N-glycosylation were introduced at positions buried in the folded protein structure. The level of glycosylation of such new acceptor sites was analysed by pulse-labelling under two sets of conditions that are known to reduce the rate of folding: (i) addition of dithiothreitol to the growth medium and (ii) introduction of deletions in the propeptide. A variety of effects was observed, depending on the position of the new acceptor sites. In some cases, all the newly synthesized mutant protein was modified at the novel site while in others no modification took place. In the most interesting category of mutants, the level of glycosylation was dependent on the conditions for folding. This shows that folding and glycosylation reactions can compete in vivo and that glycosylation does not necessarily precede folding. The approach described may be generally applicable for the analysis of protein folding in vivo.
Full text
PDF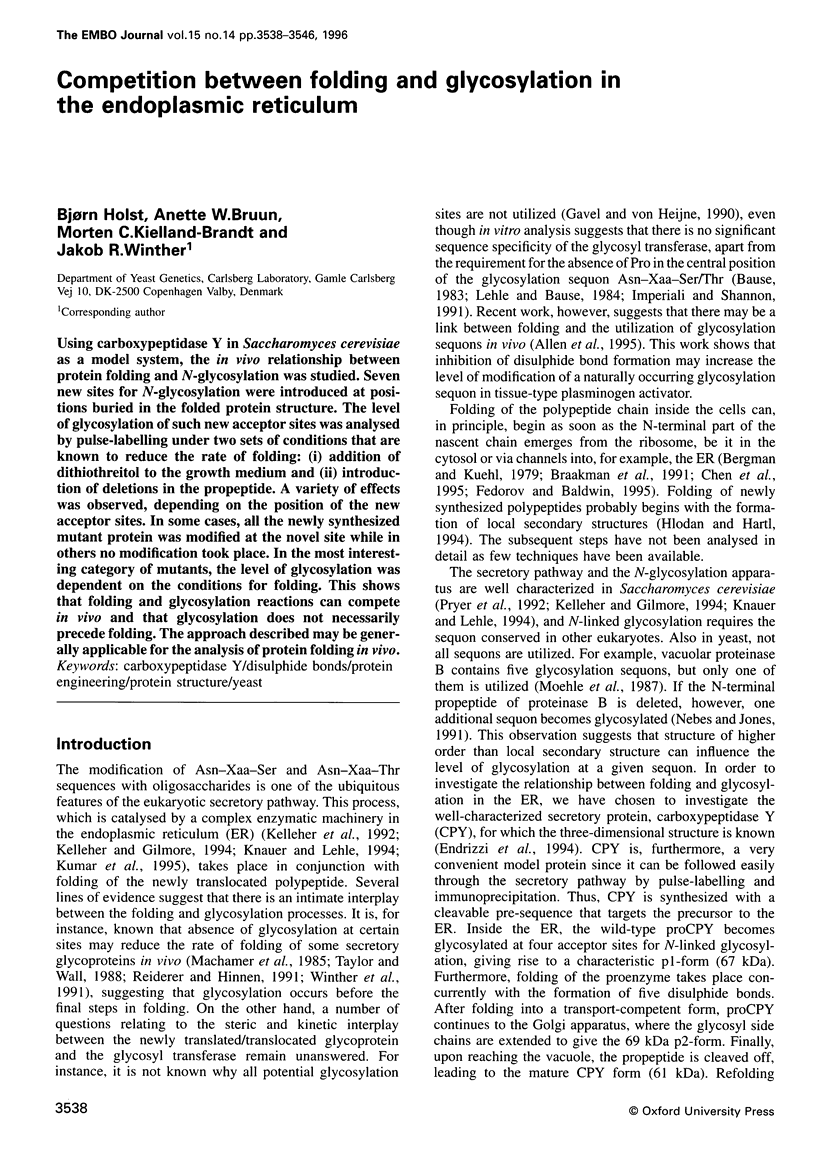
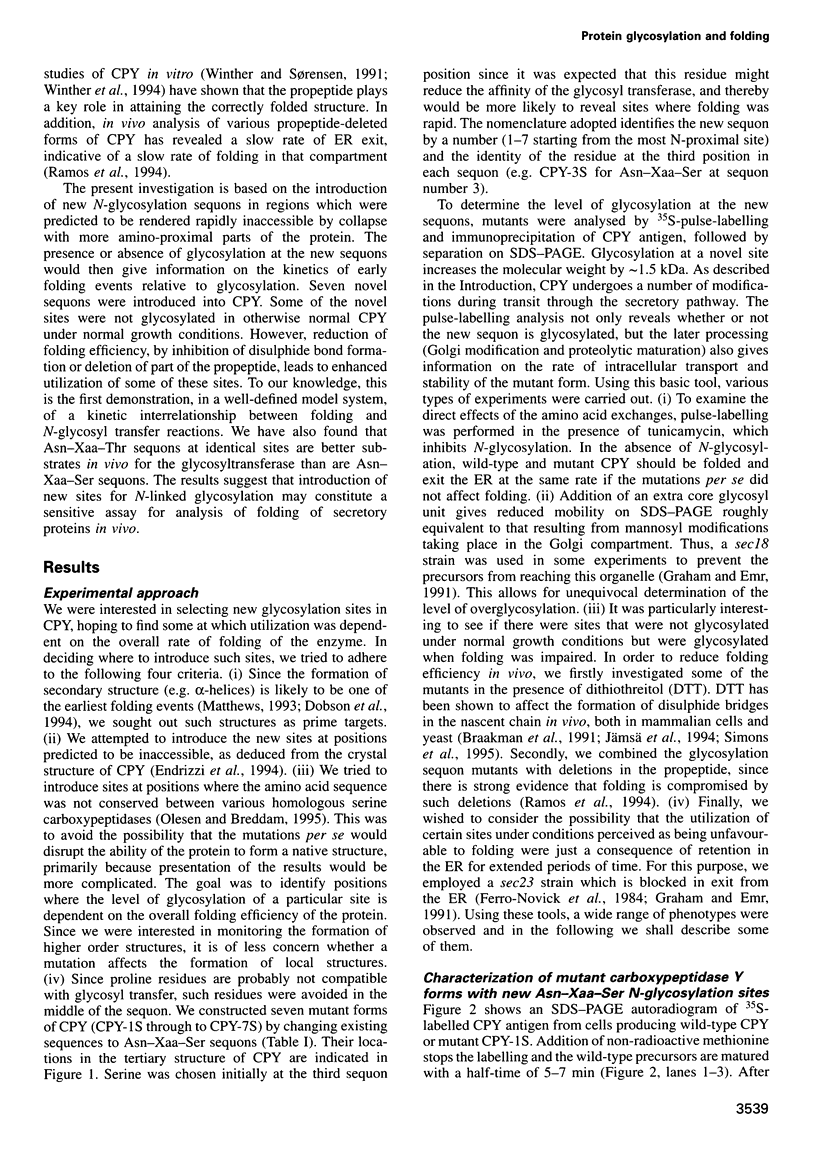
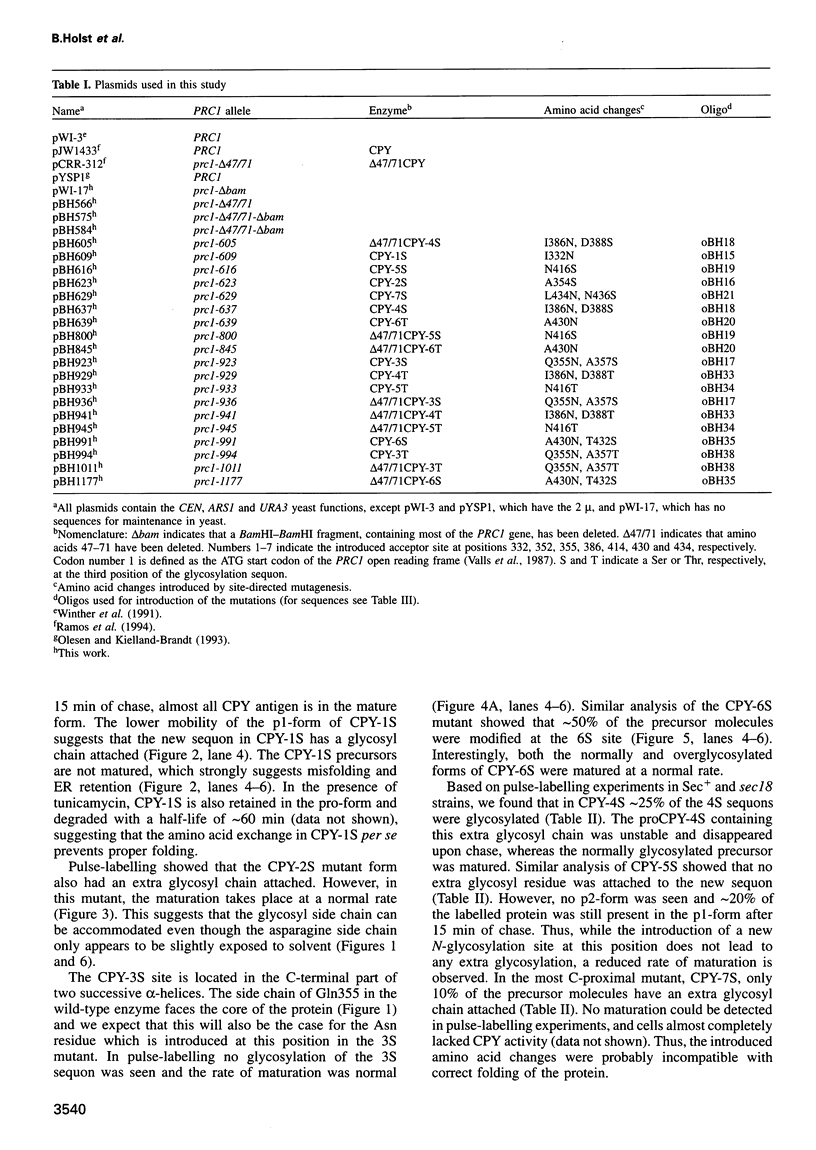
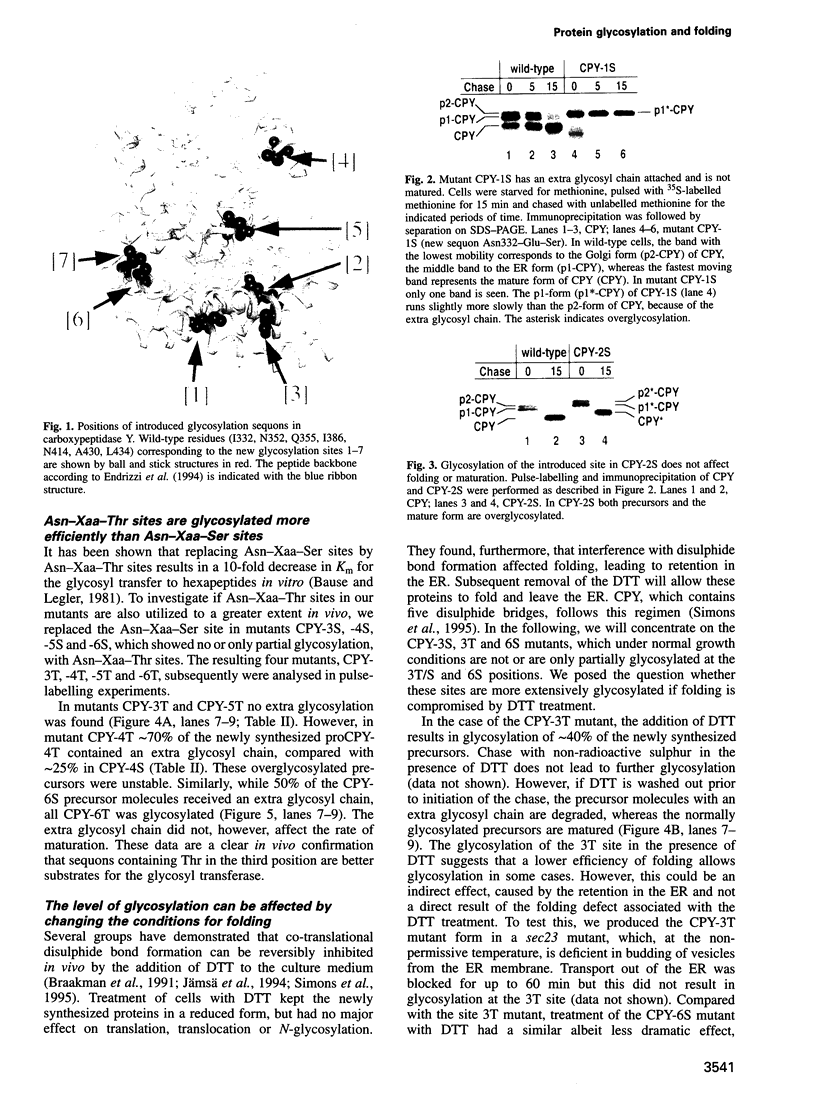
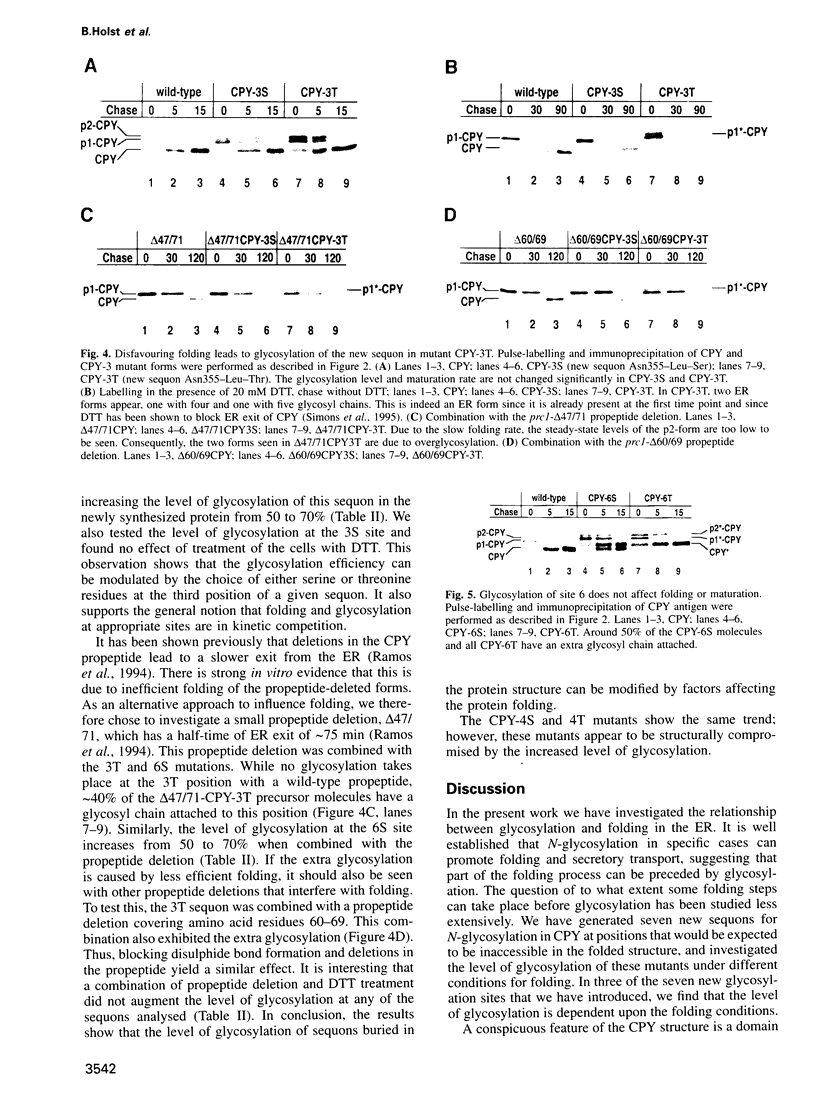
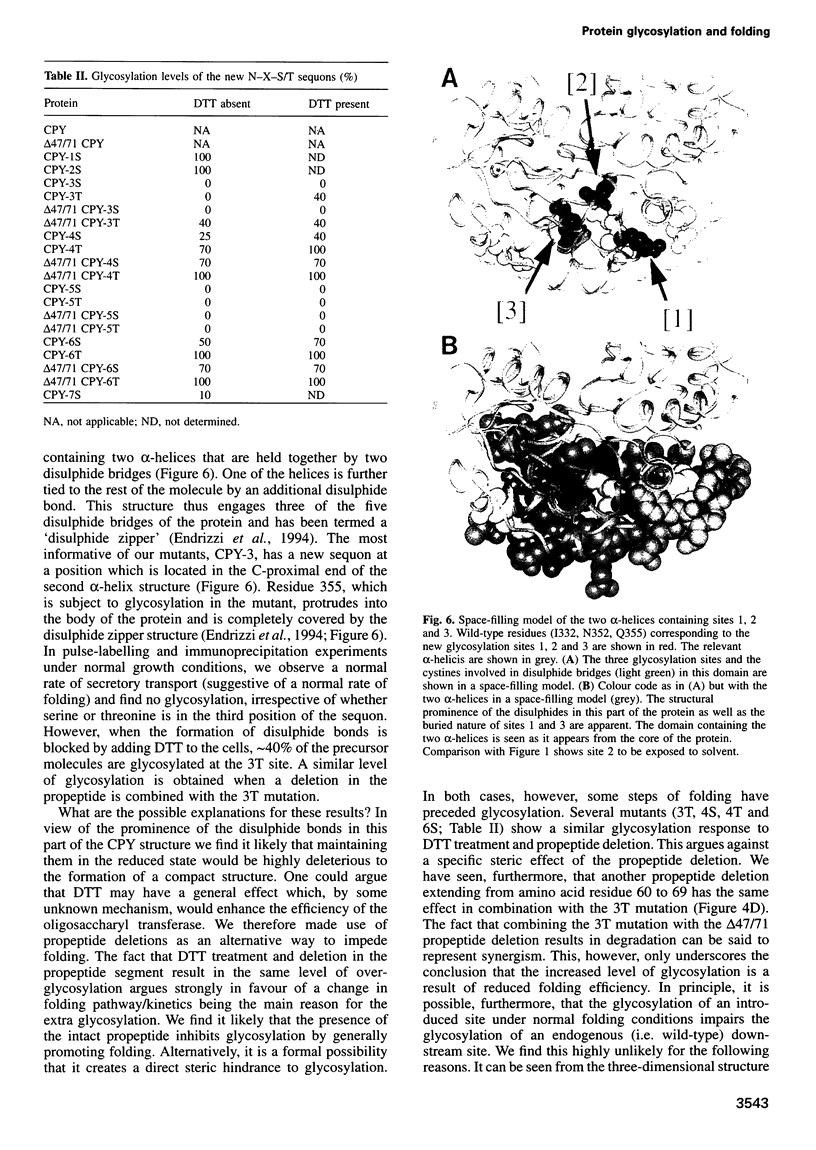
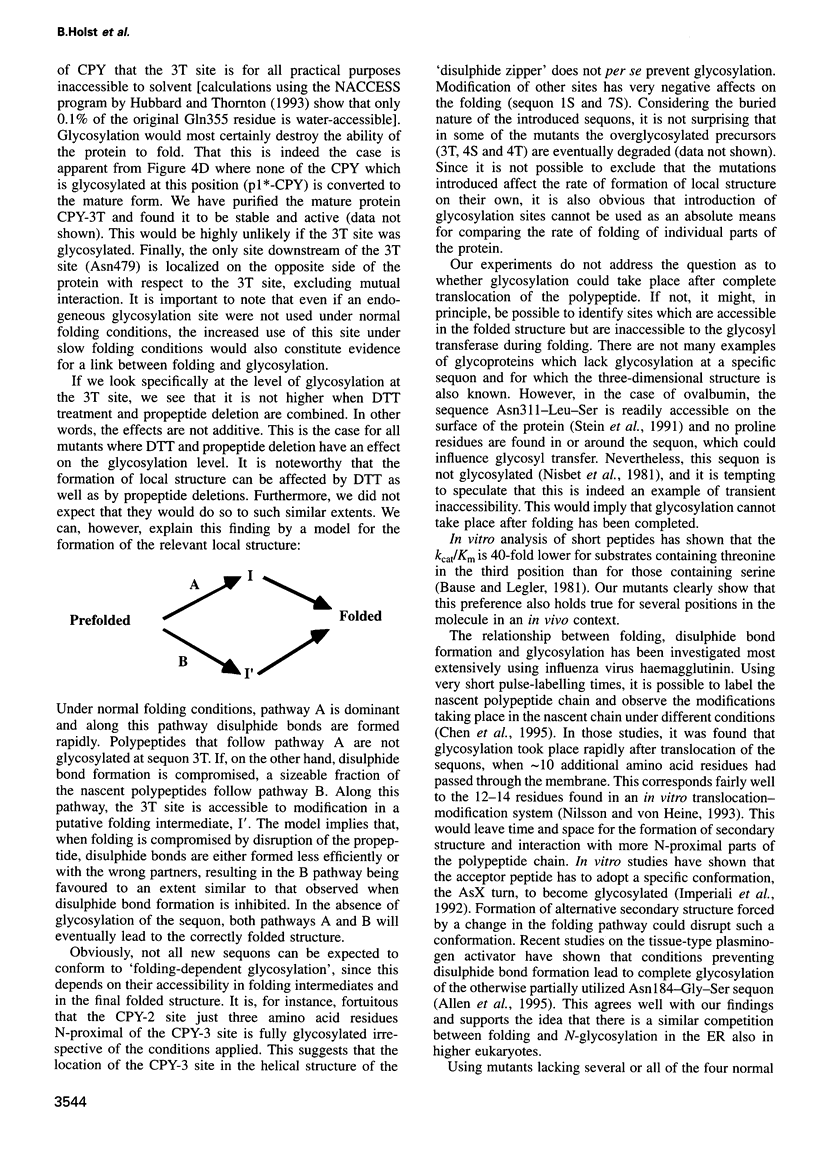
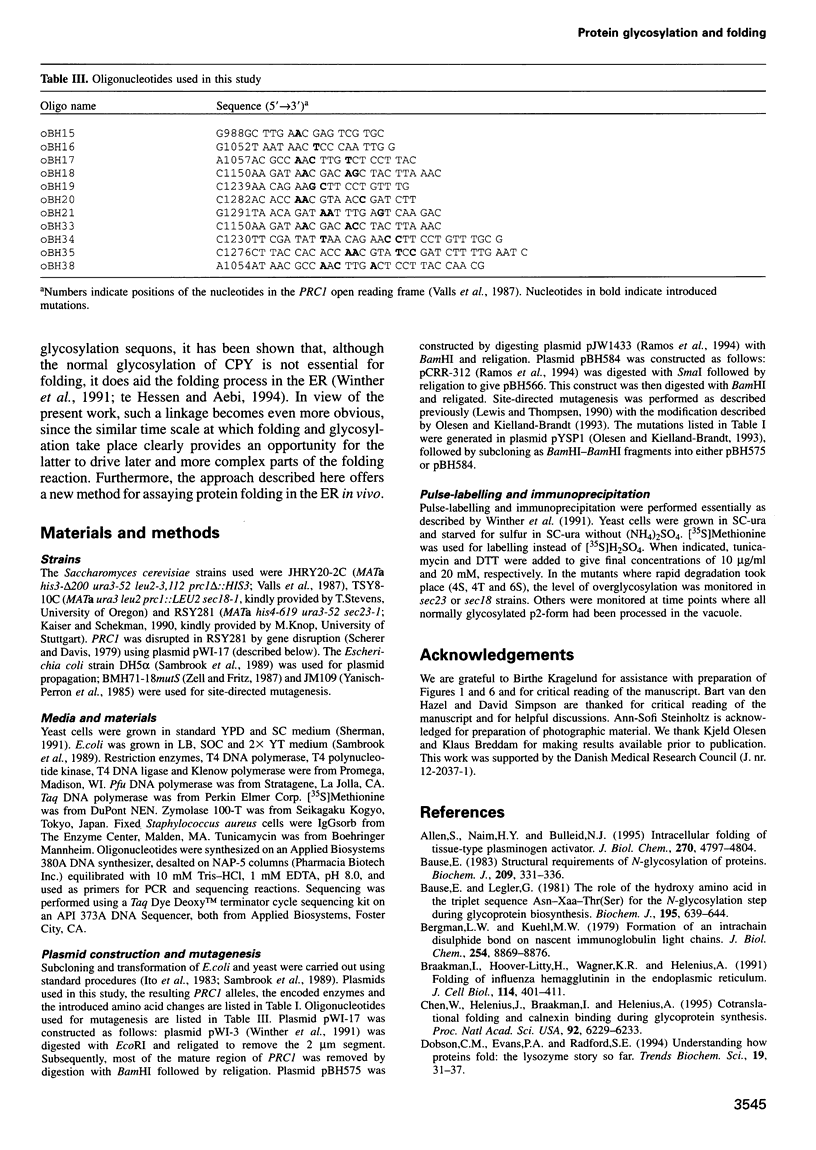
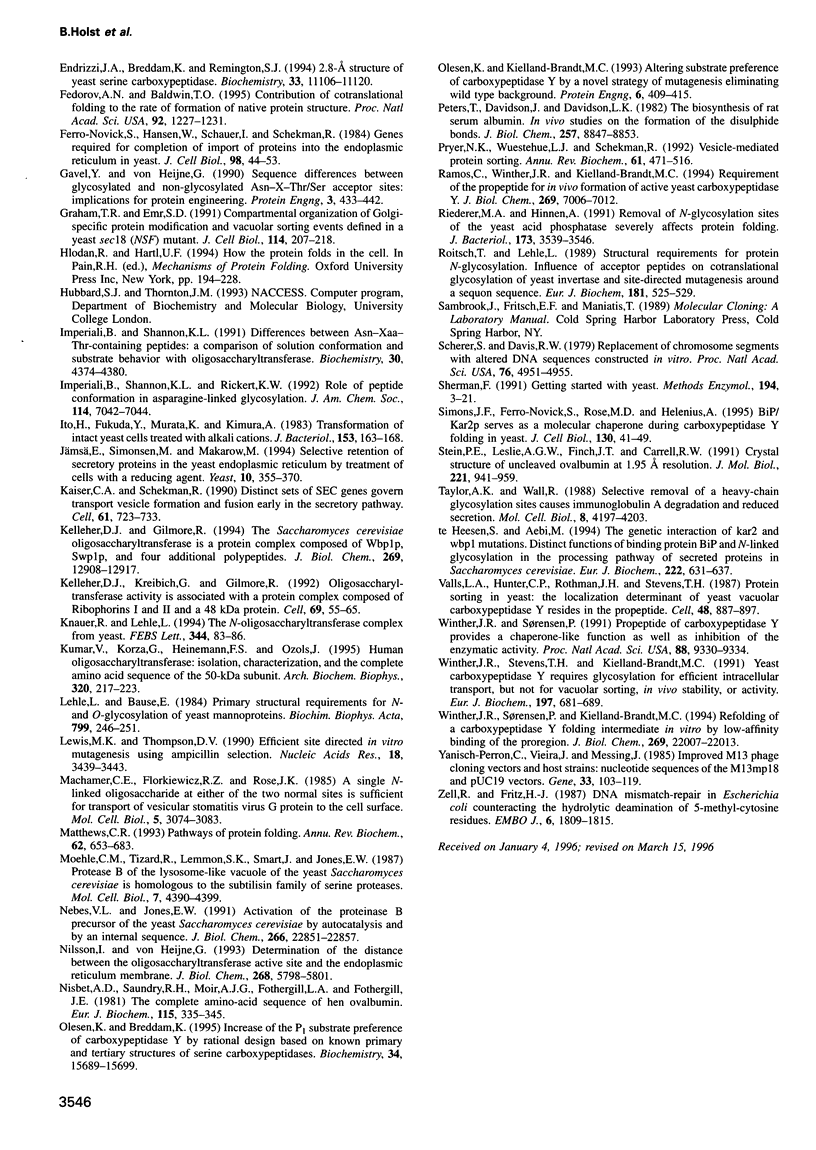
Images in this article
Selected References
These references are in PubMed. This may not be the complete list of references from this article.
- Allen S., Naim H. Y., Bulleid N. J. Intracellular folding of tissue-type plasminogen activator. Effects of disulfide bond formation on N-linked glycosylation and secretion. J Biol Chem. 1995 Mar 3;270(9):4797–4804. doi: 10.1074/jbc.270.9.4797. [DOI] [PubMed] [Google Scholar]
- Bause E., Legler G. The role of the hydroxy amino acid in the triplet sequence Asn-Xaa-Thr(Ser) for the N-glycosylation step during glycoprotein biosynthesis. Biochem J. 1981 Jun 1;195(3):639–644. doi: 10.1042/bj1950639. [DOI] [PMC free article] [PubMed] [Google Scholar]
- Bause E. Structural requirements of N-glycosylation of proteins. Studies with proline peptides as conformational probes. Biochem J. 1983 Feb 1;209(2):331–336. doi: 10.1042/bj2090331. [DOI] [PMC free article] [PubMed] [Google Scholar]
- Bergman L. W., Kuehl W. M. Formation of an intrachain disulfide bond on nascent immunoglobulin light chains. J Biol Chem. 1979 Sep 25;254(18):8869–8876. [PubMed] [Google Scholar]
- Braakman I., Hoover-Litty H., Wagner K. R., Helenius A. Folding of influenza hemagglutinin in the endoplasmic reticulum. J Cell Biol. 1991 Aug;114(3):401–411. doi: 10.1083/jcb.114.3.401. [DOI] [PMC free article] [PubMed] [Google Scholar]
- Chen W., Helenius J., Braakman I., Helenius A. Cotranslational folding and calnexin binding during glycoprotein synthesis. Proc Natl Acad Sci U S A. 1995 Jul 3;92(14):6229–6233. doi: 10.1073/pnas.92.14.6229. [DOI] [PMC free article] [PubMed] [Google Scholar]
- Dobson C. M., Evans P. A., Radford S. E. Understanding how proteins fold: the lysozyme story so far. Trends Biochem Sci. 1994 Jan;19(1):31–37. doi: 10.1016/0968-0004(94)90171-6. [DOI] [PubMed] [Google Scholar]
- Endrizzi J. A., Breddam K., Remington S. J. 2.8-A structure of yeast serine carboxypeptidase. Biochemistry. 1994 Sep 20;33(37):11106–11120. doi: 10.1021/bi00203a007. [DOI] [PubMed] [Google Scholar]
- Fedorov A. N., Baldwin T. O. Contribution of cotranslational folding to the rate of formation of native protein structure. Proc Natl Acad Sci U S A. 1995 Feb 14;92(4):1227–1231. doi: 10.1073/pnas.92.4.1227. [DOI] [PMC free article] [PubMed] [Google Scholar]
- Ferro-Novick S., Hansen W., Schauer I., Schekman R. Genes required for completion of import of proteins into the endoplasmic reticulum in yeast. J Cell Biol. 1984 Jan;98(1):44–53. doi: 10.1083/jcb.98.1.44. [DOI] [PMC free article] [PubMed] [Google Scholar]
- Gavel Y., von Heijne G. Sequence differences between glycosylated and non-glycosylated Asn-X-Thr/Ser acceptor sites: implications for protein engineering. Protein Eng. 1990 Apr;3(5):433–442. doi: 10.1093/protein/3.5.433. [DOI] [PMC free article] [PubMed] [Google Scholar]
- Graham T. R., Emr S. D. Compartmental organization of Golgi-specific protein modification and vacuolar protein sorting events defined in a yeast sec18 (NSF) mutant. J Cell Biol. 1991 Jul;114(2):207–218. doi: 10.1083/jcb.114.2.207. [DOI] [PMC free article] [PubMed] [Google Scholar]
- Imperiali B., Shannon K. L. Differences between Asn-Xaa-Thr-containing peptides: a comparison of solution conformation and substrate behavior with oligosaccharyltransferase. Biochemistry. 1991 May 7;30(18):4374–4380. doi: 10.1021/bi00232a002. [DOI] [PubMed] [Google Scholar]
- Ito H., Fukuda Y., Murata K., Kimura A. Transformation of intact yeast cells treated with alkali cations. J Bacteriol. 1983 Jan;153(1):163–168. doi: 10.1128/jb.153.1.163-168.1983. [DOI] [PMC free article] [PubMed] [Google Scholar]
- Jämsä E., Simonen M., Makarow M. Selective retention of secretory proteins in the yeast endoplasmic reticulum by treatment of cells with a reducing agent. Yeast. 1994 Mar;10(3):355–370. doi: 10.1002/yea.320100308. [DOI] [PubMed] [Google Scholar]
- Kaiser C. A., Schekman R. Distinct sets of SEC genes govern transport vesicle formation and fusion early in the secretory pathway. Cell. 1990 May 18;61(4):723–733. doi: 10.1016/0092-8674(90)90483-u. [DOI] [PubMed] [Google Scholar]
- Kelleher D. J., Gilmore R. The Saccharomyces cerevisiae oligosaccharyltransferase is a protein complex composed of Wbp1p, Swp1p, and four additional polypeptides. J Biol Chem. 1994 Apr 29;269(17):12908–12917. [PubMed] [Google Scholar]
- Kelleher D. J., Kreibich G., Gilmore R. Oligosaccharyltransferase activity is associated with a protein complex composed of ribophorins I and II and a 48 kd protein. Cell. 1992 Apr 3;69(1):55–65. doi: 10.1016/0092-8674(92)90118-v. [DOI] [PubMed] [Google Scholar]
- Knauer R., Lehle L. The N-oligosaccharyltransferase complex from yeast. FEBS Lett. 1994 May 9;344(1):83–86. doi: 10.1016/0014-5793(94)00356-4. [DOI] [PubMed] [Google Scholar]
- Kumar V., Korza G., Heinemann F. S., Ozols J. Human oligosaccharyltransferase: isolation, characterization, and the complete amino acid sequence of 50-kDa subunit. Arch Biochem Biophys. 1995 Jul 10;320(2):217–223. doi: 10.1016/0003-9861(95)90003-9. [DOI] [PubMed] [Google Scholar]
- Lewis M. K., Thompson D. V. Efficient site directed in vitro mutagenesis using ampicillin selection. Nucleic Acids Res. 1990 Jun 25;18(12):3439–3443. doi: 10.1093/nar/18.12.3439. [DOI] [PMC free article] [PubMed] [Google Scholar]
- Machamer C. E., Florkiewicz R. Z., Rose J. K. A single N-linked oligosaccharide at either of the two normal sites is sufficient for transport of vesicular stomatitis virus G protein to the cell surface. Mol Cell Biol. 1985 Nov;5(11):3074–3083. doi: 10.1128/mcb.5.11.3074. [DOI] [PMC free article] [PubMed] [Google Scholar]
- Matthews C. R. Pathways of protein folding. Annu Rev Biochem. 1993;62:653–683. doi: 10.1146/annurev.bi.62.070193.003253. [DOI] [PubMed] [Google Scholar]
- Moehle C. M., Tizard R., Lemmon S. K., Smart J., Jones E. W. Protease B of the lysosomelike vacuole of the yeast Saccharomyces cerevisiae is homologous to the subtilisin family of serine proteases. Mol Cell Biol. 1987 Dec;7(12):4390–4399. doi: 10.1128/mcb.7.12.4390. [DOI] [PMC free article] [PubMed] [Google Scholar]
- Nebes V. L., Jones E. W. Activation of the proteinase B precursor of the yeast Saccharomyces cerevisiae by autocatalysis and by an internal sequence. J Biol Chem. 1991 Dec 5;266(34):22851–22857. [PubMed] [Google Scholar]
- Nilsson I. M., von Heijne G. Determination of the distance between the oligosaccharyltransferase active site and the endoplasmic reticulum membrane. J Biol Chem. 1993 Mar 15;268(8):5798–5801. [PubMed] [Google Scholar]
- Nisbet A. D., Saundry R. H., Moir A. J., Fothergill L. A., Fothergill J. E. The complete amino-acid sequence of hen ovalbumin. Eur J Biochem. 1981 Apr;115(2):335–345. doi: 10.1111/j.1432-1033.1981.tb05243.x. [DOI] [PubMed] [Google Scholar]
- Olesen K., Breddam K. Increase of the P1 Lys/Leu substrate preference of carboxypeptidase Y by rational design based on known primary and tertiary structures of serine carboxypeptidases. Biochemistry. 1995 Dec 5;34(48):15689–15699. doi: 10.1021/bi00048a013. [DOI] [PubMed] [Google Scholar]
- Olesen K., Kielland-Brandt M. C. Altering substrate preference of carboxypeptidase Y by a novel strategy of mutagenesis eliminating wild type background. Protein Eng. 1993 Jun;6(4):409–415. doi: 10.1093/protein/6.4.409. [DOI] [PubMed] [Google Scholar]
- Peters T., Jr, Davidson L. K. The biosynthesis of rat serum albumin. In vivo studies on the formation of the disulfide bonds. J Biol Chem. 1982 Aug 10;257(15):8847–8853. [PubMed] [Google Scholar]
- Pryer N. K., Wuestehube L. J., Schekman R. Vesicle-mediated protein sorting. Annu Rev Biochem. 1992;61:471–516. doi: 10.1146/annurev.bi.61.070192.002351. [DOI] [PubMed] [Google Scholar]
- Ramos C., Winther J. R., Kielland-Brandt M. C. Requirement of the propeptide for in vivo formation of active yeast carboxypeptidase Y. J Biol Chem. 1994 Mar 4;269(9):7006–7012. [PubMed] [Google Scholar]
- Riederer M. A., Hinnen A. Removal of N-glycosylation sites of the yeast acid phosphatase severely affects protein folding. J Bacteriol. 1991 Jun;173(11):3539–3546. doi: 10.1128/jb.173.11.3539-3546.1991. [DOI] [PMC free article] [PubMed] [Google Scholar]
- Roitsch T., Lehle L. Structural requirements for protein N-glycosylation. Influence of acceptor peptides on cotranslational glycosylation of yeast invertase and site-directed mutagenesis around a sequon sequence. Eur J Biochem. 1989 May 1;181(2):525–529. doi: 10.1111/j.1432-1033.1989.tb14755.x. [DOI] [PubMed] [Google Scholar]
- Scherer S., Davis R. W. Replacement of chromosome segments with altered DNA sequences constructed in vitro. Proc Natl Acad Sci U S A. 1979 Oct;76(10):4951–4955. doi: 10.1073/pnas.76.10.4951. [DOI] [PMC free article] [PubMed] [Google Scholar]
- Sherman F. Getting started with yeast. Methods Enzymol. 1991;194:3–21. doi: 10.1016/0076-6879(91)94004-v. [DOI] [PubMed] [Google Scholar]
- Simons J. F., Ferro-Novick S., Rose M. D., Helenius A. BiP/Kar2p serves as a molecular chaperone during carboxypeptidase Y folding in yeast. J Cell Biol. 1995 Jul;130(1):41–49. doi: 10.1083/jcb.130.1.41. [DOI] [PMC free article] [PubMed] [Google Scholar]
- Stein P. E., Leslie A. G., Finch J. T., Carrell R. W. Crystal structure of uncleaved ovalbumin at 1.95 A resolution. J Mol Biol. 1991 Oct 5;221(3):941–959. doi: 10.1016/0022-2836(91)80185-w. [DOI] [PubMed] [Google Scholar]
- Taylor A. K., Wall R. Selective removal of alpha heavy-chain glycosylation sites causes immunoglobulin A degradation and reduced secretion. Mol Cell Biol. 1988 Oct;8(10):4197–4203. doi: 10.1128/mcb.8.10.4197. [DOI] [PMC free article] [PubMed] [Google Scholar]
- Valls L. A., Hunter C. P., Rothman J. H., Stevens T. H. Protein sorting in yeast: the localization determinant of yeast vacuolar carboxypeptidase Y resides in the propeptide. Cell. 1987 Mar 13;48(5):887–897. doi: 10.1016/0092-8674(87)90085-7. [DOI] [PubMed] [Google Scholar]
- Winther J. R., Stevens T. H., Kielland-Brandt M. C. Yeast carboxypeptidase Y requires glycosylation for efficient intracellular transport, but not for vacuolar sorting, in vivo stability, or activity. Eur J Biochem. 1991 May 8;197(3):681–689. doi: 10.1111/j.1432-1033.1991.tb15959.x. [DOI] [PubMed] [Google Scholar]
- Winther J. R., Sørensen P., Kielland-Brandt M. C. Refolding of a carboxypeptidase Y folding intermediate in vitro by low-affinity binding of the proregion. J Biol Chem. 1994 Sep 2;269(35):22007–22013. [PubMed] [Google Scholar]
- Winther J. R., Sørensen P. Propeptide of carboxypeptidase Y provides a chaperone-like function as well as inhibition of the enzymatic activity. Proc Natl Acad Sci U S A. 1991 Oct 15;88(20):9330–9334. doi: 10.1073/pnas.88.20.9330. [DOI] [PMC free article] [PubMed] [Google Scholar]
- Yanisch-Perron C., Vieira J., Messing J. Improved M13 phage cloning vectors and host strains: nucleotide sequences of the M13mp18 and pUC19 vectors. Gene. 1985;33(1):103–119. doi: 10.1016/0378-1119(85)90120-9. [DOI] [PubMed] [Google Scholar]
- Zell R., Fritz H. J. DNA mismatch-repair in Escherichia coli counteracting the hydrolytic deamination of 5-methyl-cytosine residues. EMBO J. 1987 Jun;6(6):1809–1815. doi: 10.1002/j.1460-2075.1987.tb02435.x. [DOI] [PMC free article] [PubMed] [Google Scholar]
- te Heesen S., Aebi M. The genetic interaction of kar2 and wbp1 mutations. Distinct functions of binding protein BiP and N-linked glycosylation in the processing pathway of secreted proteins in Saccharomyces cerevisiae. Eur J Biochem. 1994 Jun 1;222(2):631–637. doi: 10.1111/j.1432-1033.1994.tb18906.x. [DOI] [PubMed] [Google Scholar]