Abstract
Metal cations are known to be required for proton pumping by bacteriorhodopsin (bR). Previous studies found that bR has two high-affinity and four to six low-affinity Ca(2+)-binding sites. In our efforts to find the location of these Ca2+ sites, the effects of replacing charged (Asp-85, Asp-212, and Arg-82) and H-bonding (Tyr-185) residues in the retinal pocket on the color control and binding affinity of Ca2+ ions in Ca(2+)-regenerated bR were examined. The important results are as follows: (i) The removal of Ca2+ from recombinant bR in which charged residues were replaced by neutral ones shifted the retinal absorption to the blue, opposite to that observed in wild-type bR or in recombinant bR in which the H-bonding residue, Tyr-185, was replaced by a non-H-bonding amino acid (Phe). (ii) Similar to the observation in wild-type bR, the binding of Ca2+ to the second site gave the observed color change in the recombinant bR samples in which charged residues were replaced by neutral ones. (iii) The residue replacements had no effect on the affinity constants of the four to six weakly bound Ca2+. (iv) The two high-affinity sites exhibited reduced affinity with substitutions; while the extent of the reduction depended on the specific substitution, each site was reduced by the same factor for each of the charged residue substitutions but by different factors for the mutant where Tyr-185 was replaced with Phe(Y185F). The above results suggest that the two Ca2+ ions in the two high-affinity sites are within interaction distance with one another and with the charged residues in the retinal pocket. The results further suggest that, while the interaction between Tyr-185 and the high-affinity Ca2+ ions is relatively short range and specific (with more coupling to the Ca2+ ion in the second affinity site), between the charged residues and Ca2+ ions it seems to be of the electrostatic (e.g., ion-ion) long range, nonspecific type. Although neither Asp-85, Asp-212, nor Arg-82 is individually directly involved in the binding of Ca2+ in these two sites, they might all participate in it. Together with the protonated Schiff base, the charged residues along with Tyr-185 and one or two Ca2+ ions (and probably a few water molecules) seem to form an electrostatically coupled system that is part of a cavity that controls the color and function of bR.
Full text
PDF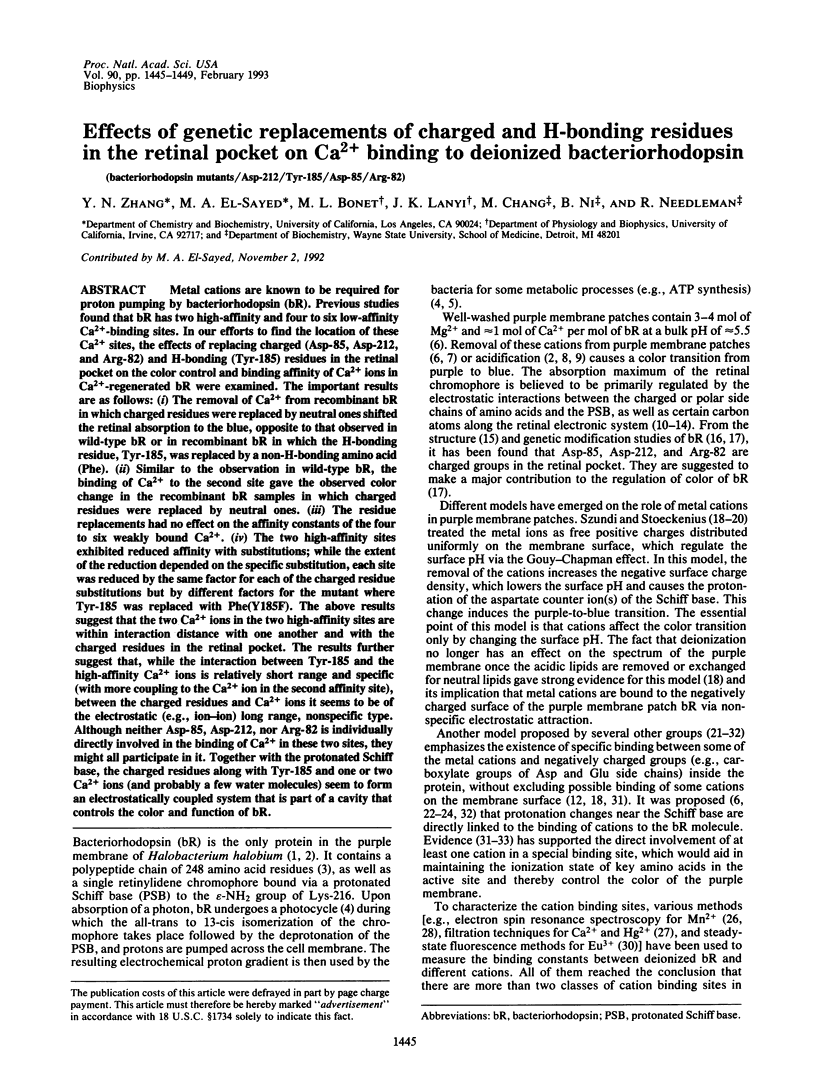
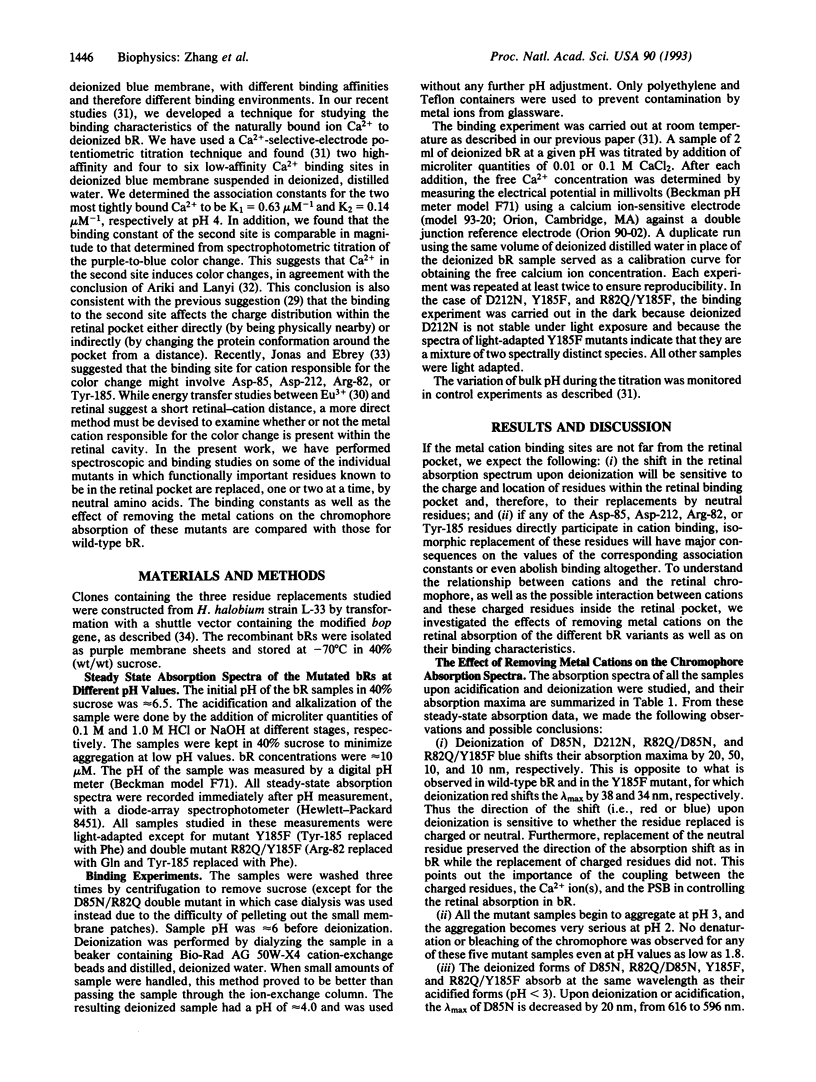
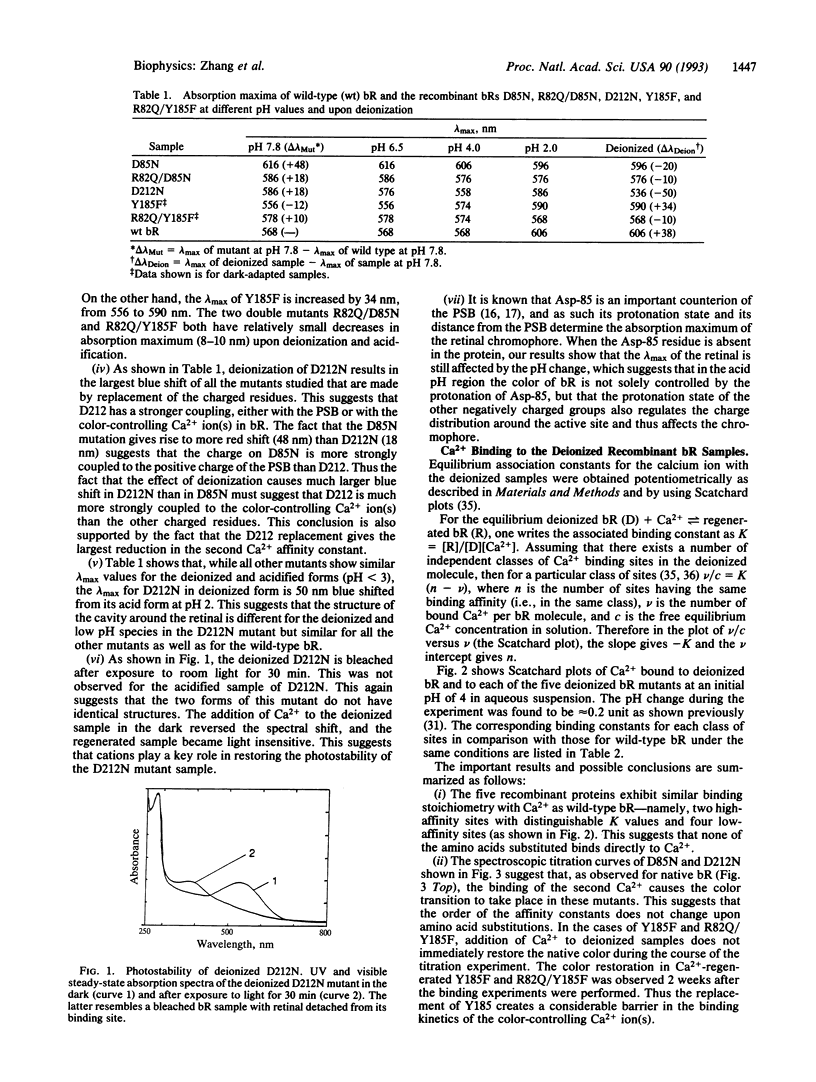
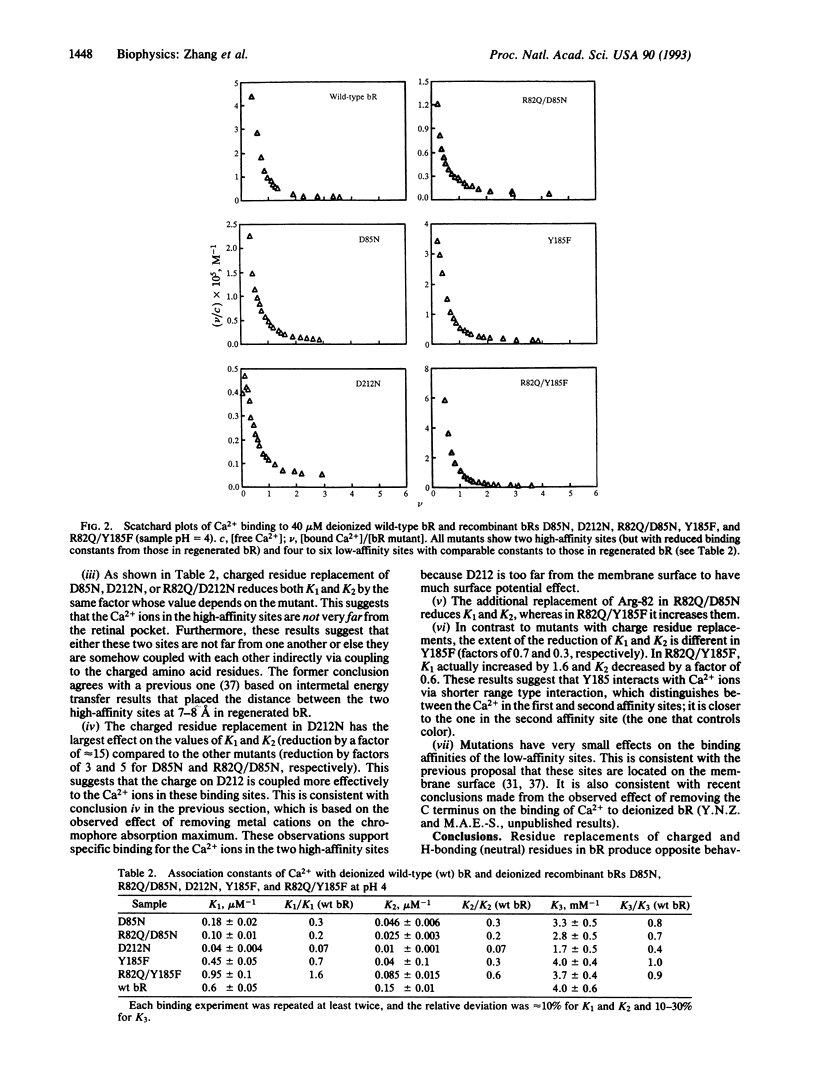
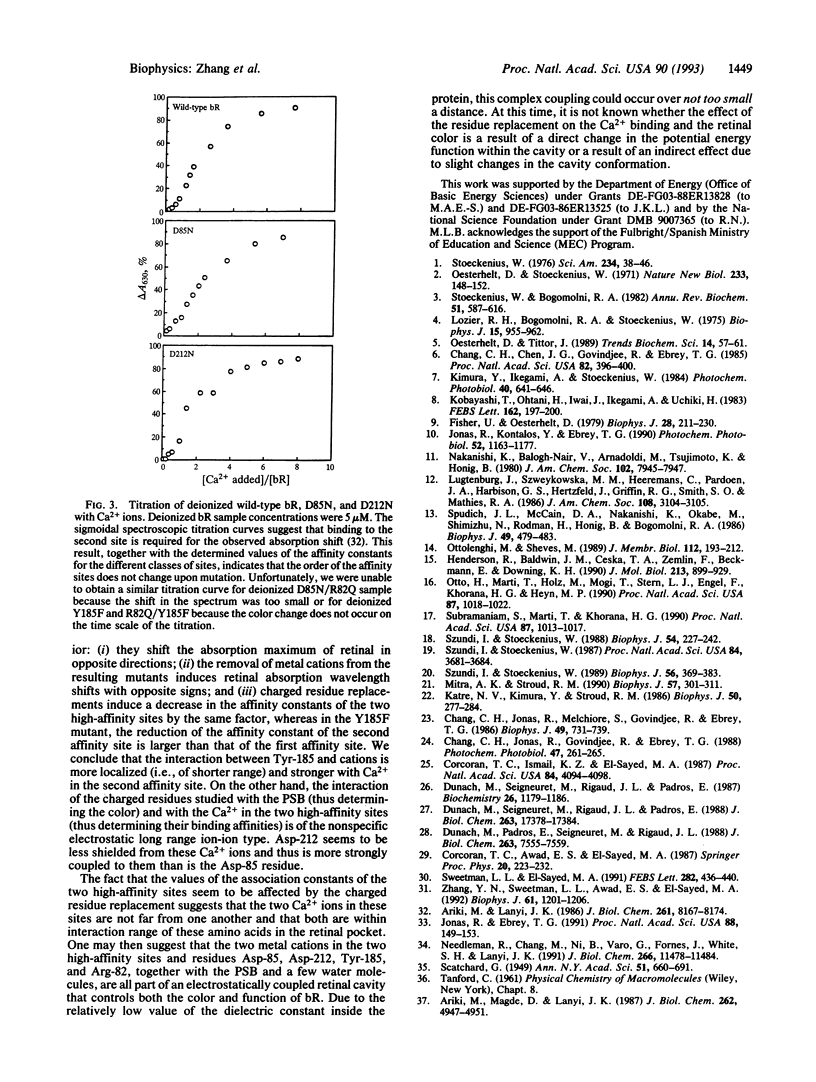
Selected References
These references are in PubMed. This may not be the complete list of references from this article.
- Ariki M., Lanyi J. K. Characterization of metal ion-binding sites in bacteriorhodopsin. J Biol Chem. 1986 Jun 25;261(18):8167–8174. [PubMed] [Google Scholar]
- Ariki M., Magde D., Lanyi J. K. Metal ion binding sites of bacteriorhodopsin. Laser-induced lanthanide luminescence study. J Biol Chem. 1987 Apr 15;262(11):4947–4951. [PubMed] [Google Scholar]
- Chang C. H., Chen J. G., Govindjee R., Ebrey T. Cation binding by bacteriorhodopsin. Proc Natl Acad Sci U S A. 1985 Jan;82(2):396–400. doi: 10.1073/pnas.82.2.396. [DOI] [PMC free article] [PubMed] [Google Scholar]
- Chang C. H., Jonas R., Melchiore S., Govindjee R., Ebrey T. G. Mechanism and role of divalent cation binding of bacteriorhodopsin. Biophys J. 1986 Mar;49(3):731–739. doi: 10.1016/S0006-3495(86)83699-2. [DOI] [PMC free article] [PubMed] [Google Scholar]
- Corcoran T. C., Ismail K. Z., El-Sayed M. A. Evidence for the involvement of more than one metal cation in the Schiff base deprotonation process during the photocycle of bacteriorhodopsin. Proc Natl Acad Sci U S A. 1987 Jun;84(12):4094–4098. doi: 10.1073/pnas.84.12.4094. [DOI] [PMC free article] [PubMed] [Google Scholar]
- Duñach M., Padrós E., Seigneuret M., Rigaud J. L. On the molecular mechanism of the blue to purple transition of bacteriorhodopsin. UV-difference spectroscopy and electron spin resonance studies. J Biol Chem. 1988 Jun 5;263(16):7555–7559. [PubMed] [Google Scholar]
- Duñach M., Seigneuret M., Rigaud J. L., Padrós E. Influence of cations on the blue to purple transition of bacteriorhodopsin. Comparison of Ca2+ and Hg2+ binding and their effect on the surface potential. J Biol Chem. 1988 Nov 25;263(33):17378–17384. [PubMed] [Google Scholar]
- Fischer U., Oesterhelt D. Chromophore equilibria in bacteriorhodopsin. Biophys J. 1979 Nov;28(2):211–230. doi: 10.1016/S0006-3495(79)85172-3. [DOI] [PMC free article] [PubMed] [Google Scholar]
- Henderson R., Baldwin J. M., Ceska T. A., Zemlin F., Beckmann E., Downing K. H. Model for the structure of bacteriorhodopsin based on high-resolution electron cryo-microscopy. J Mol Biol. 1990 Jun 20;213(4):899–929. doi: 10.1016/S0022-2836(05)80271-2. [DOI] [PubMed] [Google Scholar]
- Jonas R., Ebrey T. G. Binding of a single divalent cation directly correlates with the blue-to-purple transition in bacteriorhodopsin. Proc Natl Acad Sci U S A. 1991 Jan 1;88(1):149–153. doi: 10.1073/pnas.88.1.149. [DOI] [PMC free article] [PubMed] [Google Scholar]
- Jonas R., Koutalos Y., Ebrey T. G. Purple membrane: surface charge density and the multiple effect of pH and cations. Photochem Photobiol. 1990 Dec;52(6):1163–1177. doi: 10.1111/j.1751-1097.1990.tb08455.x. [DOI] [PubMed] [Google Scholar]
- Katre N. V., Kimura Y., Stroud R. M. Cation binding sites on the projected structure of bacteriorhodopsin. Biophys J. 1986 Aug;50(2):277–284. doi: 10.1016/S0006-3495(86)83461-0. [DOI] [PMC free article] [PubMed] [Google Scholar]
- Kimura Y., Ikegami A., Stoeckenius W. Salt and pH-dependent changes of the purple membrane absorption spectrum. Photochem Photobiol. 1984 Nov;40(5):641–646. doi: 10.1111/j.1751-1097.1984.tb05353.x. [DOI] [PubMed] [Google Scholar]
- Lozier R. H., Bogomolni R. A., Stoeckenius W. Bacteriorhodopsin: a light-driven proton pump in Halobacterium Halobium. Biophys J. 1975 Sep;15(9):955–962. doi: 10.1016/S0006-3495(75)85875-9. [DOI] [PMC free article] [PubMed] [Google Scholar]
- Mitra A. K., Stroud R. M. High sensitivity electron diffraction analysis. A study of divalent cation binding to purple membrane. Biophys J. 1990 Feb;57(2):301–311. doi: 10.1016/S0006-3495(90)82532-7. [DOI] [PMC free article] [PubMed] [Google Scholar]
- Needleman R., Chang M., Ni B., Váró G., Fornés J., White S. H., Lanyi J. K. Properties of Asp212----Asn bacteriorhodopsin suggest that Asp212 and Asp85 both participate in a counterion and proton acceptor complex near the Schiff base. J Biol Chem. 1991 Jun 25;266(18):11478–11484. [PubMed] [Google Scholar]
- Oesterhelt D., Stoeckenius W. Rhodopsin-like protein from the purple membrane of Halobacterium halobium. Nat New Biol. 1971 Sep 29;233(39):149–152. doi: 10.1038/newbio233149a0. [DOI] [PubMed] [Google Scholar]
- Oesterhelt D., Tittor J. Two pumps, one principle: light-driven ion transport in halobacteria. Trends Biochem Sci. 1989 Feb;14(2):57–61. doi: 10.1016/0968-0004(89)90044-3. [DOI] [PubMed] [Google Scholar]
- Otto H., Marti T., Holz M., Mogi T., Stern L. J., Engel F., Khorana H. G., Heyn M. P. Substitution of amino acids Asp-85, Asp-212, and Arg-82 in bacteriorhodopsin affects the proton release phase of the pump and the pK of the Schiff base. Proc Natl Acad Sci U S A. 1990 Feb;87(3):1018–1022. doi: 10.1073/pnas.87.3.1018. [DOI] [PMC free article] [PubMed] [Google Scholar]
- Ottolenghi M., Sheves M. Synthetic retinals as probes for the binding site and photoreactions in rhodopsins. J Membr Biol. 1989 Dec;112(3):193–212. doi: 10.1007/BF01870951. [DOI] [PubMed] [Google Scholar]
- Spudich J. L., McCain D. A., Nakanishi K., Okabe M., Shimizu N., Rodman H., Honig B., Bogomolni R. A. Chromophore/protein interaction in bacterial sensory rhodopsin and bacteriorhodopsin. Biophys J. 1986 Feb;49(2):479–483. doi: 10.1016/S0006-3495(86)83657-8. [DOI] [PMC free article] [PubMed] [Google Scholar]
- Stoeckenius W., Bogomolni R. A. Bacteriorhodopsin and related pigments of halobacteria. Annu Rev Biochem. 1982;51:587–616. doi: 10.1146/annurev.bi.51.070182.003103. [DOI] [PubMed] [Google Scholar]
- Stoeckenius W. The purple membrane of salt-loving bacteria. Sci Am. 1976 Jun;234(6):38–46. doi: 10.1038/scientificamerican0676-38. [DOI] [PubMed] [Google Scholar]
- Subramaniam S., Marti T., Khorana H. G. Protonation state of Asp (Glu)-85 regulates the purple-to-blue transition in bacteriorhodopsin mutants Arg-82----Ala and Asp-85----Glu: the blue form is inactive in proton translocation. Proc Natl Acad Sci U S A. 1990 Feb;87(3):1013–1017. doi: 10.1073/pnas.87.3.1013. [DOI] [PMC free article] [PubMed] [Google Scholar]
- Sweetman L. L., el-Sayed M. A. The binding site of the strongly bound Eu3+ in Eu(3+)-regenerated bacteriorhodopsin. FEBS Lett. 1991 May 6;282(2):436–440. doi: 10.1016/0014-5793(91)80531-7. [DOI] [PubMed] [Google Scholar]
- Szundi I., Stoeckenius W. Effect of lipid surface charges on the purple-to-blue transition of bacteriorhodopsin. Proc Natl Acad Sci U S A. 1987 Jun;84(11):3681–3684. doi: 10.1073/pnas.84.11.3681. [DOI] [PMC free article] [PubMed] [Google Scholar]
- Szundi I., Stoeckenius W. Purple-to-blue transition of bacteriorhodopsin in a neutral lipid environment. Biophys J. 1988 Aug;54(2):227–232. doi: 10.1016/S0006-3495(88)82951-5. [DOI] [PMC free article] [PubMed] [Google Scholar]
- Szundi I., Stoeckenius W. Surface pH controls purple-to-blue transition of bacteriorhodopsin. A theoretical model of purple membrane surface. Biophys J. 1989 Aug;56(2):369–383. doi: 10.1016/S0006-3495(89)82683-9. [DOI] [PMC free article] [PubMed] [Google Scholar]
- Zhang Y. N., Sweetman L. L., Awad E. S., El-Sayed M. A. Nature of the individual Ca binding sites in Ca-regenerated bacteriorhodopsin. Biophys J. 1992 May;61(5):1201–1206. doi: 10.1016/S0006-3495(92)81929-X. [DOI] [PMC free article] [PubMed] [Google Scholar]