Abstract
Biological nitrogen fixation is catalyzed by nitrogenase, a complex metalloenzyme composed of two separately purifiable component proteins encoded by the structural genes nifH, nifD, and nifK. Deletion of the Azotobacter vinelandii nifS gene lowers the activities of both nitrogenase component proteins. Because both nitrogenase component proteins have metallocluster prosthetic groups that are composed of iron- and sulfur-containing cores, this result indicated that the nifS gene product could be involved in the mobilization of the iron or sulfur required for metallocluster formation. In the present work, it is shown that NIFS is a pyridoxal phosphate-containing homodimer that catalyzes the formation of L-alanine and elemental sulfur by using L-cysteine as substrate. NIFS activity is extremely sensitive to thiol-specific alkylating reagents, which indicates the participation of a cysteinyl thiolate at the active site. Based on these results we propose that an enzyme-bound cysteinyl persulfide that requires the release of the sulfur from the substrate L-cysteine for its formation ultimately provides the inorganic sulfide required for nitrogenase metallocluster formation. The recent discovery of nifS-like genes in non-nitrogen-fixing organisms also raises the possibility that the reaction catalyzed by NIFS represents a universal mechanism that involves pyridoxal phosphate chemistry, in the mobilization of the sulfur required for metallocluster formation.
Full text
PDF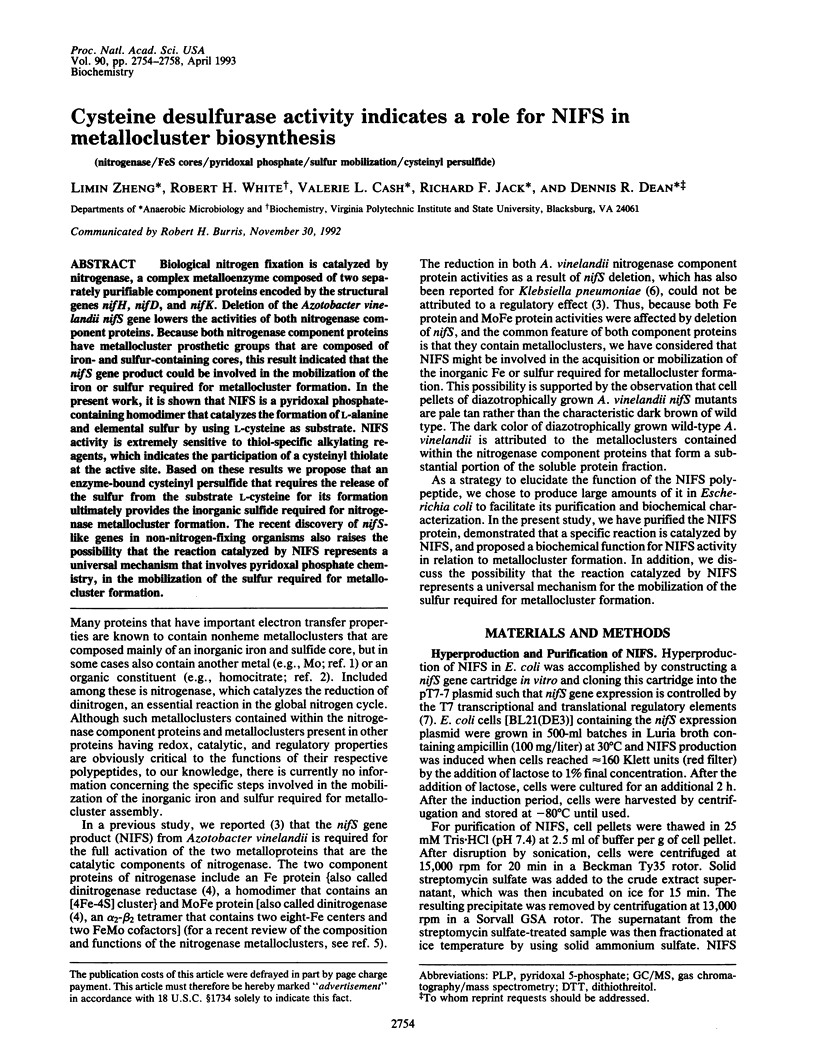
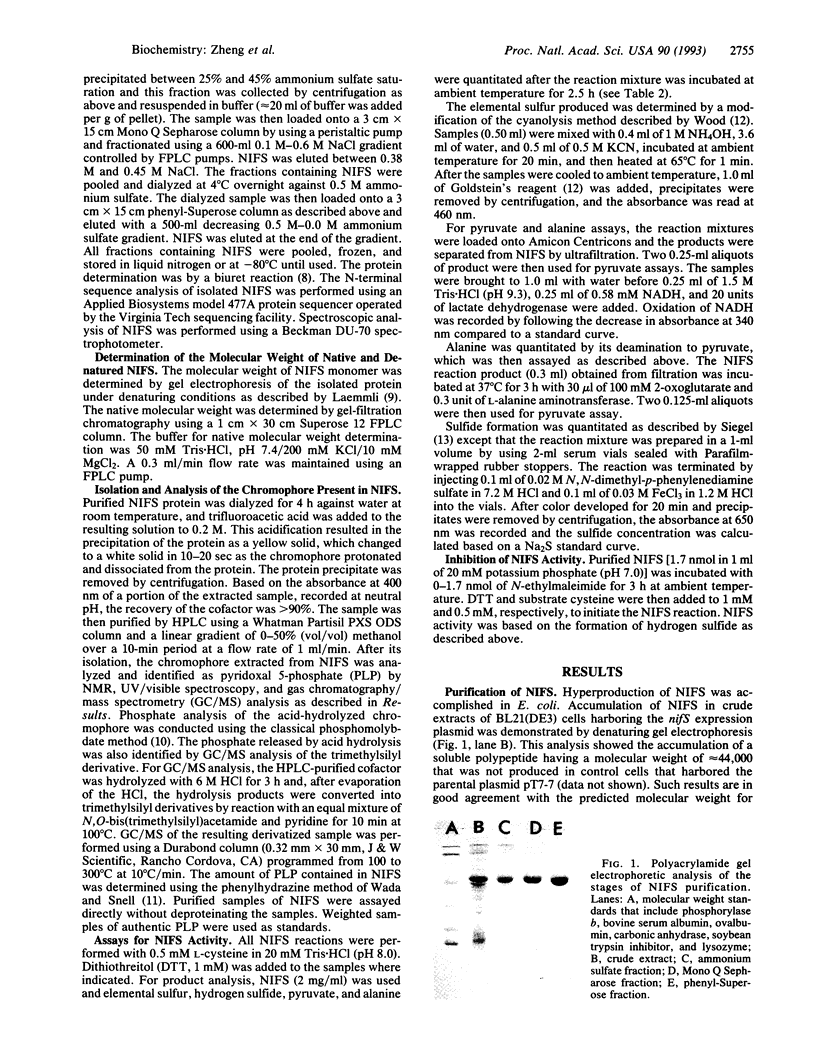
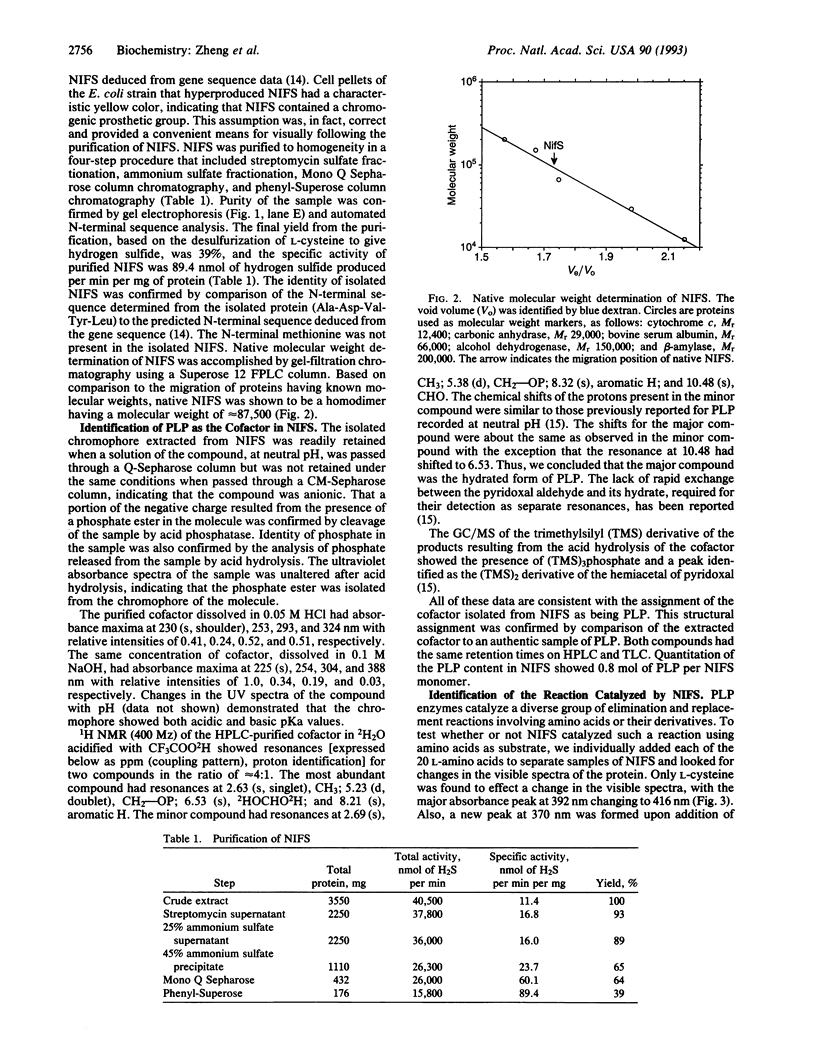
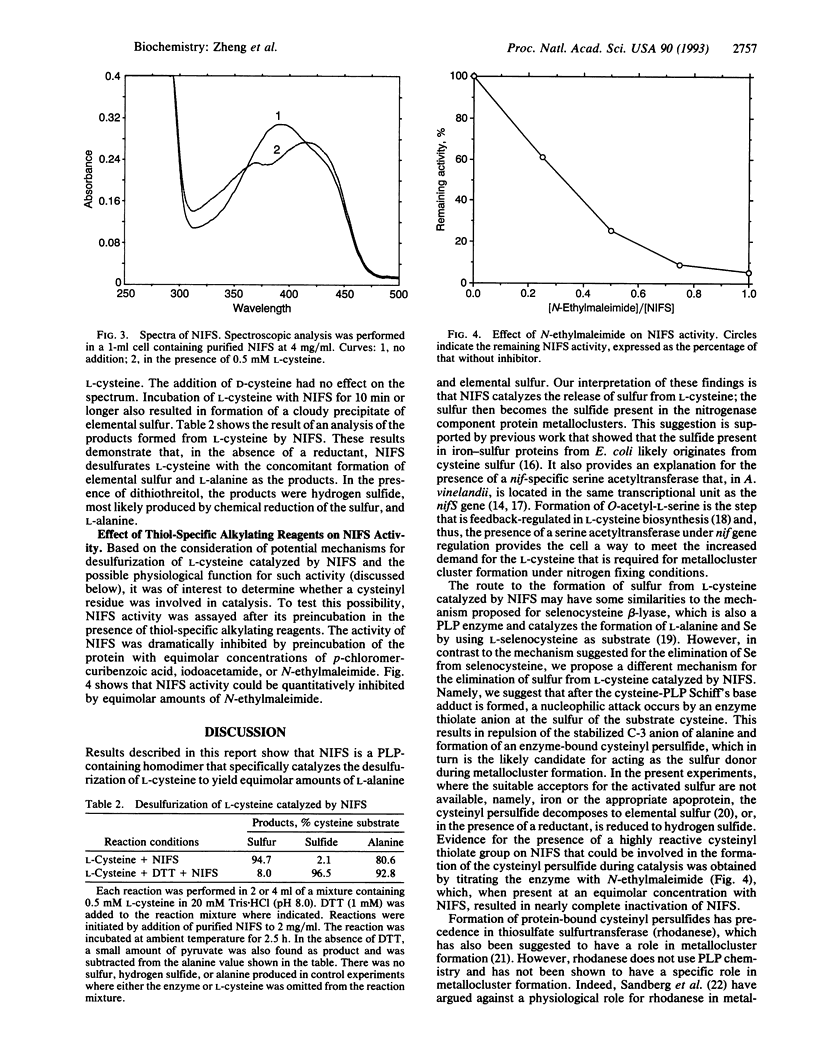
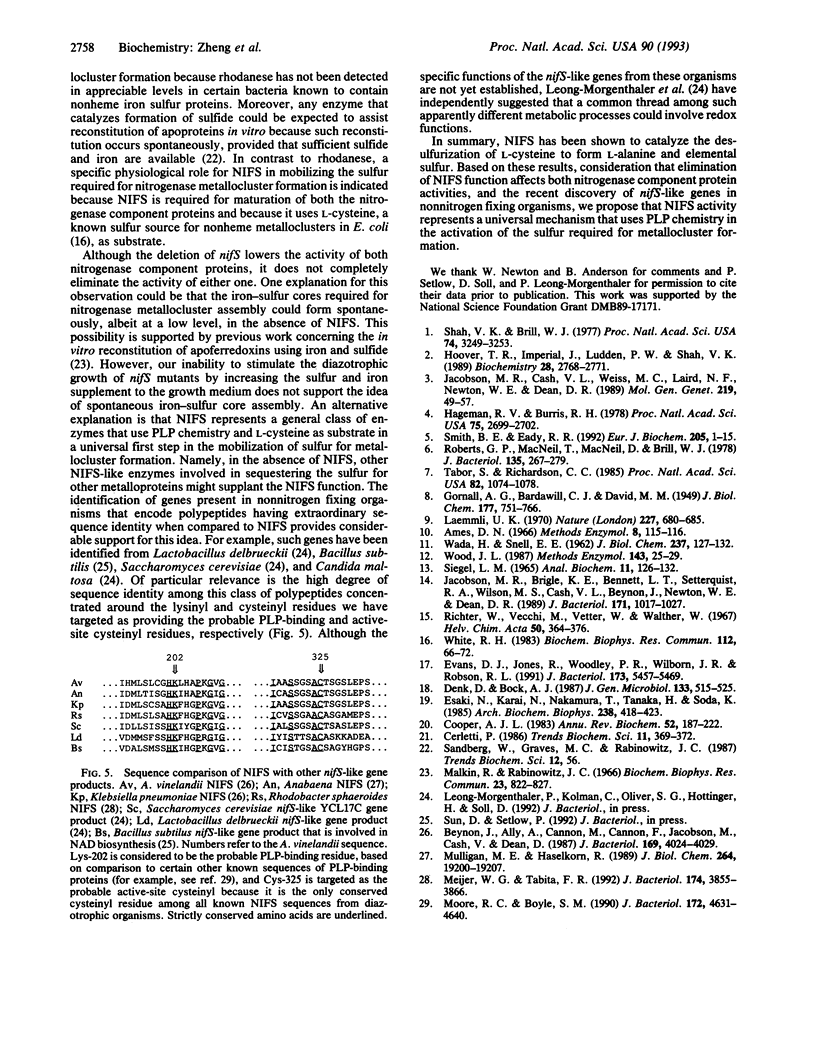
Images in this article
Selected References
These references are in PubMed. This may not be the complete list of references from this article.
- Beynon J., Ally A., Cannon M., Cannon F., Jacobson M., Cash V., Dean D. Comparative organization of nitrogen fixation-specific genes from Azotobacter vinelandii and Klebsiella pneumoniae: DNA sequence of the nifUSV genes. J Bacteriol. 1987 Sep;169(9):4024–4029. doi: 10.1128/jb.169.9.4024-4029.1987. [DOI] [PMC free article] [PubMed] [Google Scholar]
- Cooper A. J. Biochemistry of sulfur-containing amino acids. Annu Rev Biochem. 1983;52:187–222. doi: 10.1146/annurev.bi.52.070183.001155. [DOI] [PubMed] [Google Scholar]
- Denk D., Böck A. L-cysteine biosynthesis in Escherichia coli: nucleotide sequence and expression of the serine acetyltransferase (cysE) gene from the wild-type and a cysteine-excreting mutant. J Gen Microbiol. 1987 Mar;133(3):515–525. doi: 10.1099/00221287-133-3-515. [DOI] [PubMed] [Google Scholar]
- Esaki N., Karai N., Nakamura T., Tanaka H., Soda K. Mechanism of reactions catalyzed by selenocysteine beta-lyase. Arch Biochem Biophys. 1985 May 1;238(2):418–423. doi: 10.1016/0003-9861(85)90182-1. [DOI] [PubMed] [Google Scholar]
- Evans D. J., Jones R., Woodley P. R., Wilborn J. R., Robson R. L. Nucleotide sequence and genetic analysis of the Azotobacter chroococcum nifUSVWZM gene cluster, including a new gene (nifP) which encodes a serine acetyltransferase. J Bacteriol. 1991 Sep;173(17):5457–5469. doi: 10.1128/jb.173.17.5457-5469.1991. [DOI] [PMC free article] [PubMed] [Google Scholar]
- Hageman R. V., Burris R. H. Nitrogenase and nitrogenase reductase associate and dissociate with each catalytic cycle. Proc Natl Acad Sci U S A. 1978 Jun;75(6):2699–2702. doi: 10.1073/pnas.75.6.2699. [DOI] [PMC free article] [PubMed] [Google Scholar]
- Hoover T. R., Imperial J., Ludden P. W., Shah V. K. Homocitrate is a component of the iron-molybdenum cofactor of nitrogenase. Biochemistry. 1989 Apr 4;28(7):2768–2771. doi: 10.1021/bi00433a004. [DOI] [PubMed] [Google Scholar]
- Jacobson M. R., Brigle K. E., Bennett L. T., Setterquist R. A., Wilson M. S., Cash V. L., Beynon J., Newton W. E., Dean D. R. Physical and genetic map of the major nif gene cluster from Azotobacter vinelandii. J Bacteriol. 1989 Feb;171(2):1017–1027. doi: 10.1128/jb.171.2.1017-1027.1989. [DOI] [PMC free article] [PubMed] [Google Scholar]
- Jacobson M. R., Cash V. L., Weiss M. C., Laird N. F., Newton W. E., Dean D. R. Biochemical and genetic analysis of the nifUSVWZM cluster from Azotobacter vinelandii. Mol Gen Genet. 1989 Oct;219(1-2):49–57. doi: 10.1007/BF00261156. [DOI] [PubMed] [Google Scholar]
- Laemmli U. K. Cleavage of structural proteins during the assembly of the head of bacteriophage T4. Nature. 1970 Aug 15;227(5259):680–685. doi: 10.1038/227680a0. [DOI] [PubMed] [Google Scholar]
- Malkin R., Rabinowitz J. C. The reconstitution of clostridial ferredoxin. Biochem Biophys Res Commun. 1966 Jun 21;23(6):822–827. doi: 10.1016/0006-291x(66)90561-4. [DOI] [PubMed] [Google Scholar]
- Meijer W. G., Tabita F. R. Isolation and characterization of the nifUSVW-rpoN gene cluster from Rhodobacter sphaeroides. J Bacteriol. 1992 Jun;174(12):3855–3866. doi: 10.1128/jb.174.12.3855-3866.1992. [DOI] [PMC free article] [PubMed] [Google Scholar]
- Moore R. C., Boyle S. M. Nucleotide sequence and analysis of the speA gene encoding biosynthetic arginine decarboxylase in Escherichia coli. J Bacteriol. 1990 Aug;172(8):4631–4640. doi: 10.1128/jb.172.8.4631-4640.1990. [DOI] [PMC free article] [PubMed] [Google Scholar]
- Mulligan M. E., Haselkorn R. Nitrogen fixation (nif) genes of the cyanobacterium Anabaena species strain PCC 7120. The nifB-fdxN-nifS-nifU operon. J Biol Chem. 1989 Nov 15;264(32):19200–19207. [PubMed] [Google Scholar]
- Roberts G. P., MacNeil T., MacNeil D., Brill W. J. Regulation and characterization of protein products coded by the nif (nitrogen fixation) genes of Klebsiella pneumoniae. J Bacteriol. 1978 Oct;136(1):267–279. doi: 10.1128/jb.136.1.267-279.1978. [DOI] [PMC free article] [PubMed] [Google Scholar]
- SIEGEL L. M. A DIRECT MICRODETERMINATION FOR SULFIDE. Anal Biochem. 1965 Apr;11:126–132. doi: 10.1016/0003-2697(65)90051-5. [DOI] [PubMed] [Google Scholar]
- Shah V. K., Brill W. J. Isolation of an iron-molybdenum cofactor from nitrogenase. Proc Natl Acad Sci U S A. 1977 Aug;74(8):3249–3253. doi: 10.1073/pnas.74.8.3249. [DOI] [PMC free article] [PubMed] [Google Scholar]
- Smith B. E., Eady R. R. Metalloclusters of the nitrogenases. Eur J Biochem. 1992 Apr 1;205(1):1–15. doi: 10.1111/j.1432-1033.1992.tb16746.x. [DOI] [PubMed] [Google Scholar]
- Tabor S., Richardson C. C. A bacteriophage T7 RNA polymerase/promoter system for controlled exclusive expression of specific genes. Proc Natl Acad Sci U S A. 1985 Feb;82(4):1074–1078. doi: 10.1073/pnas.82.4.1074. [DOI] [PMC free article] [PubMed] [Google Scholar]
- WADA H., SNELL E. E. Enzymatic transamination of pyridoxamine. I. With oxaloacetate and alpha-ketoglutarate. J Biol Chem. 1962 Jan;237:127–132. [PubMed] [Google Scholar]
- White R. H. Origin of the labile sulfide in the iron-sulfur proteins of Escherichia coli. Biochem Biophys Res Commun. 1983 Apr 15;112(1):66–72. doi: 10.1016/0006-291x(83)91798-9. [DOI] [PubMed] [Google Scholar]
- Wood J. L. Sulfane sulfur. Methods Enzymol. 1987;143:25–29. doi: 10.1016/0076-6879(87)43009-7. [DOI] [PubMed] [Google Scholar]