Abstract
Little is known about the signaling pathways by which motoneurons induce synapses on muscle fibers, and no receptors for synapse-inducing signals have yet been identified. Because several other inductive events in development are mediated by receptor tyrosine kinases (RTKs), and because phosphotyrosine staining within muscle fibers is concentrated at synaptic sites, one possibility is that synapse-inducing signals are transduced by a RTK within the muscle fiber. We have used PCR to search for tyrosine kinases within the electric organ of the electric ray Torpedo californica, since this tissue is homologous to muscle but is much more densely innervated and is therefore a rich source of synaptic molecules. We have isolated a RTK that is specifically expressed in electric organ and skeletal muscle. The kinase domain of this receptor is related to the trk family of neurotrophin receptors, but unlike any previously described receptor, the extracellular region of this Torpedo RTK contains a kringle domain close to the transmembrane domain.
Full text
PDF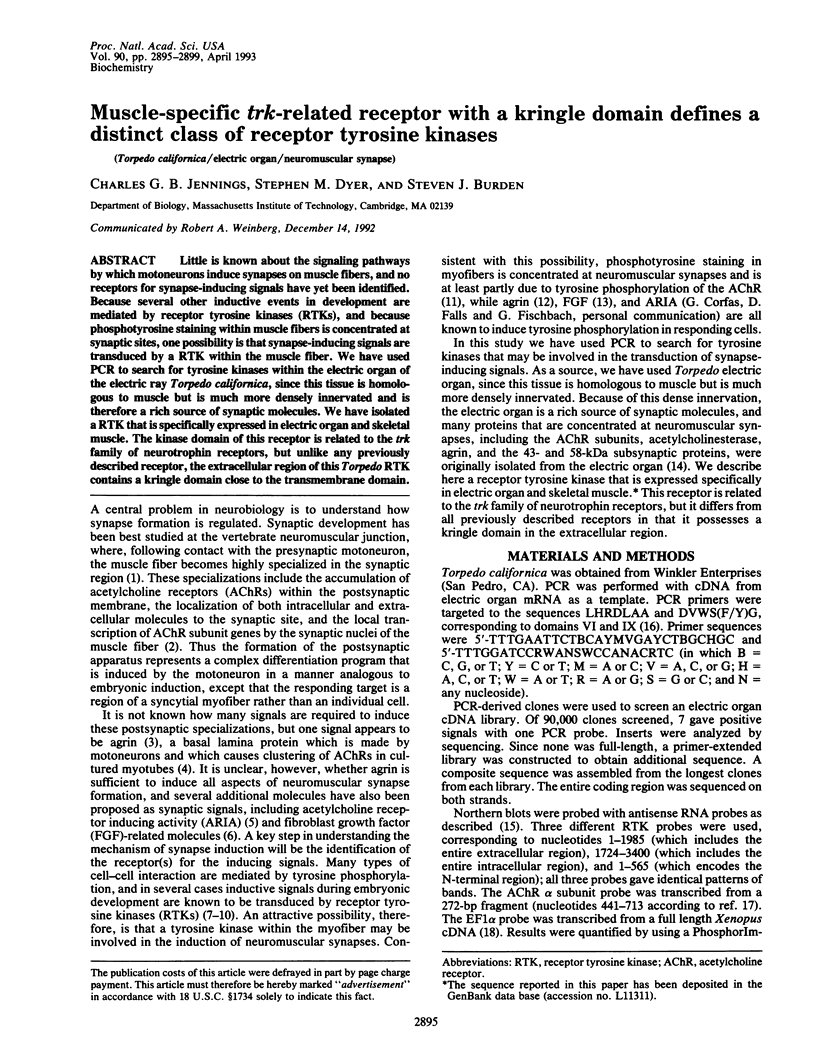
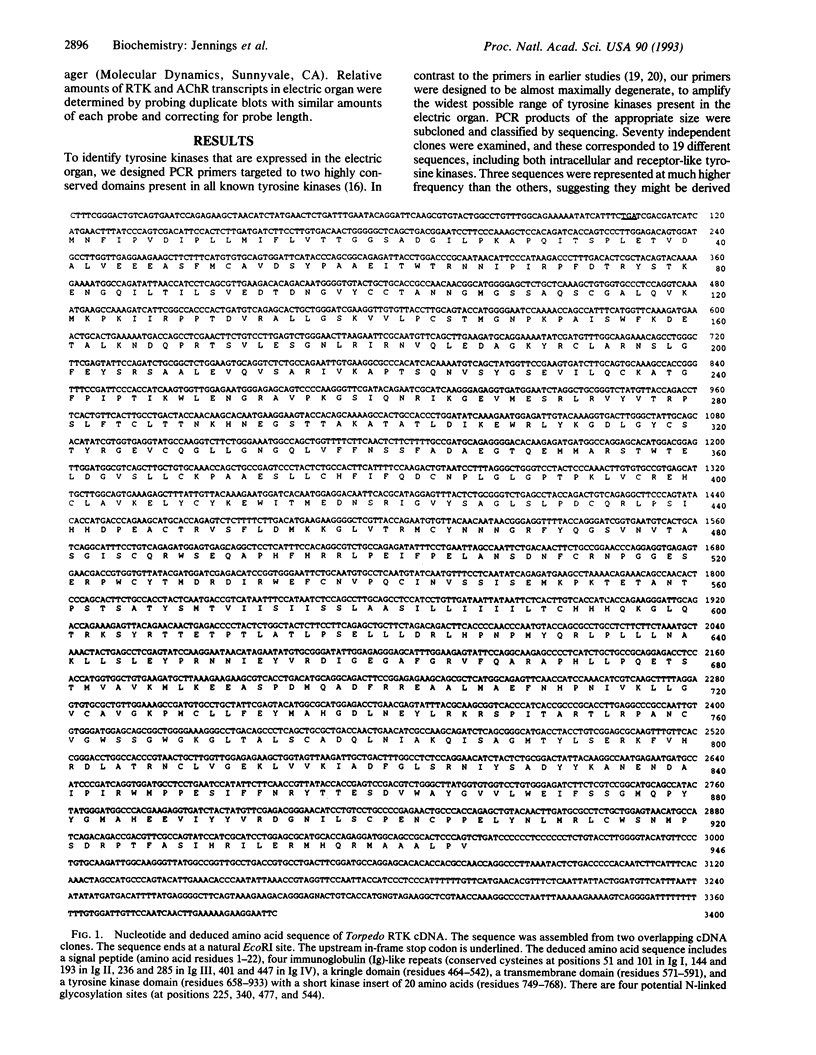
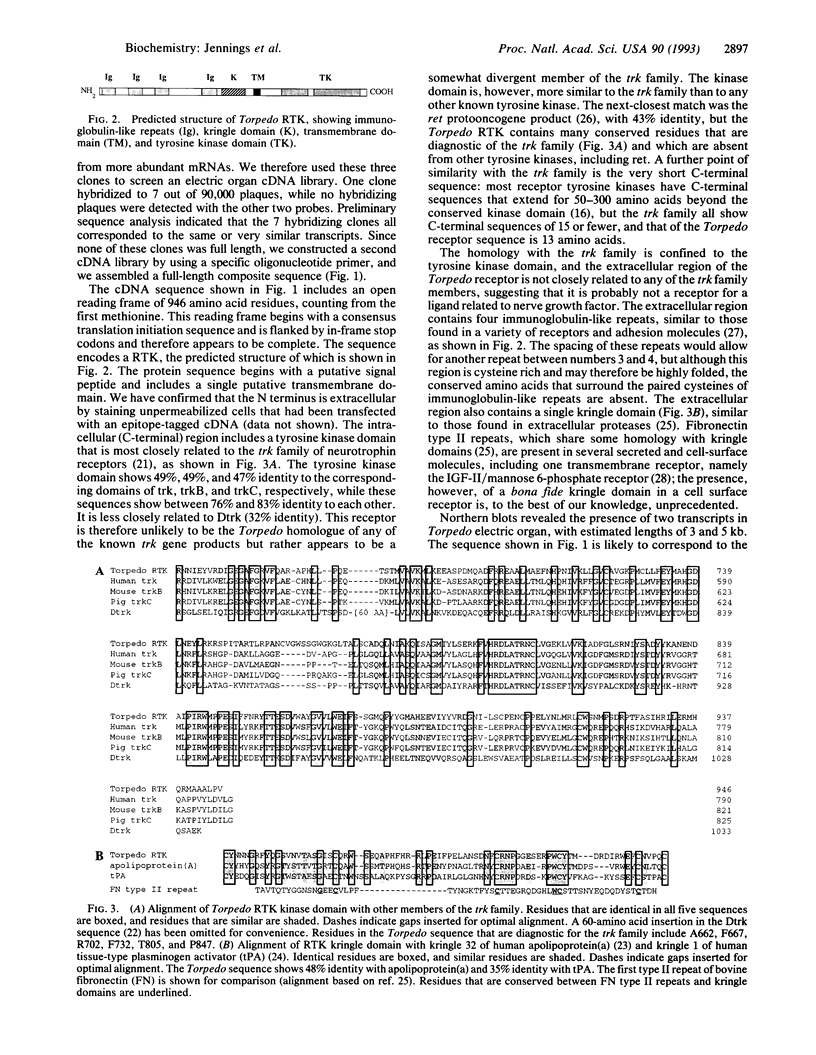
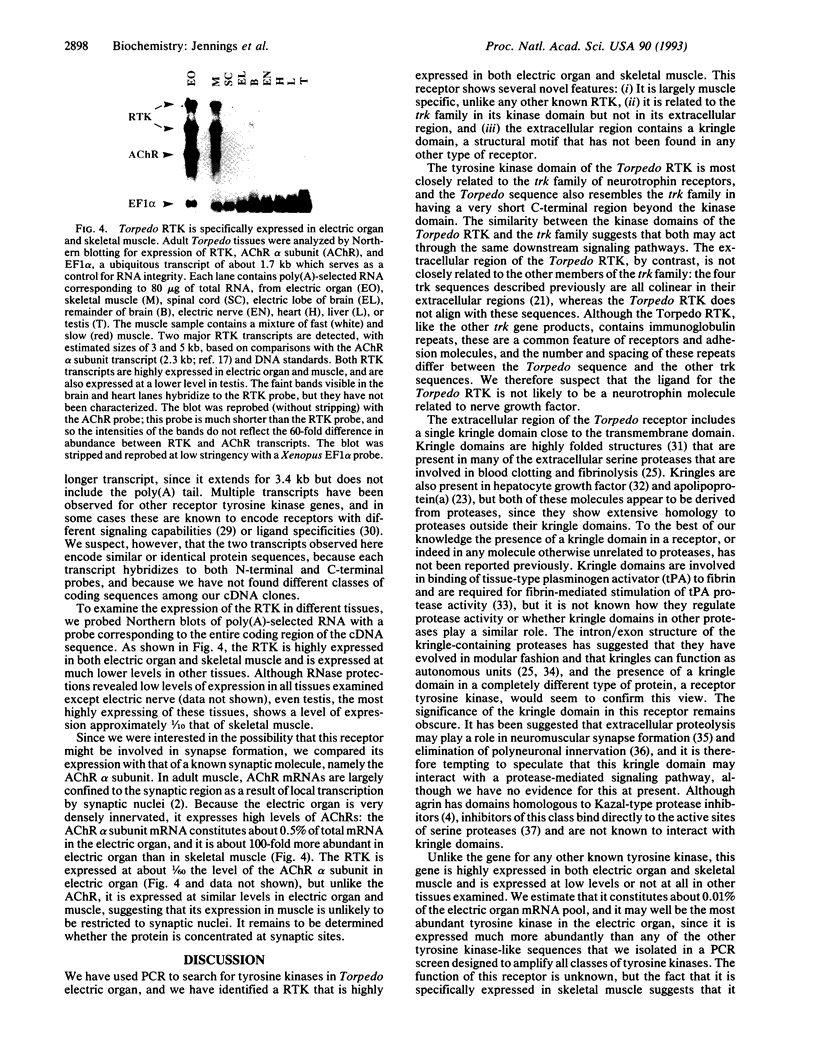
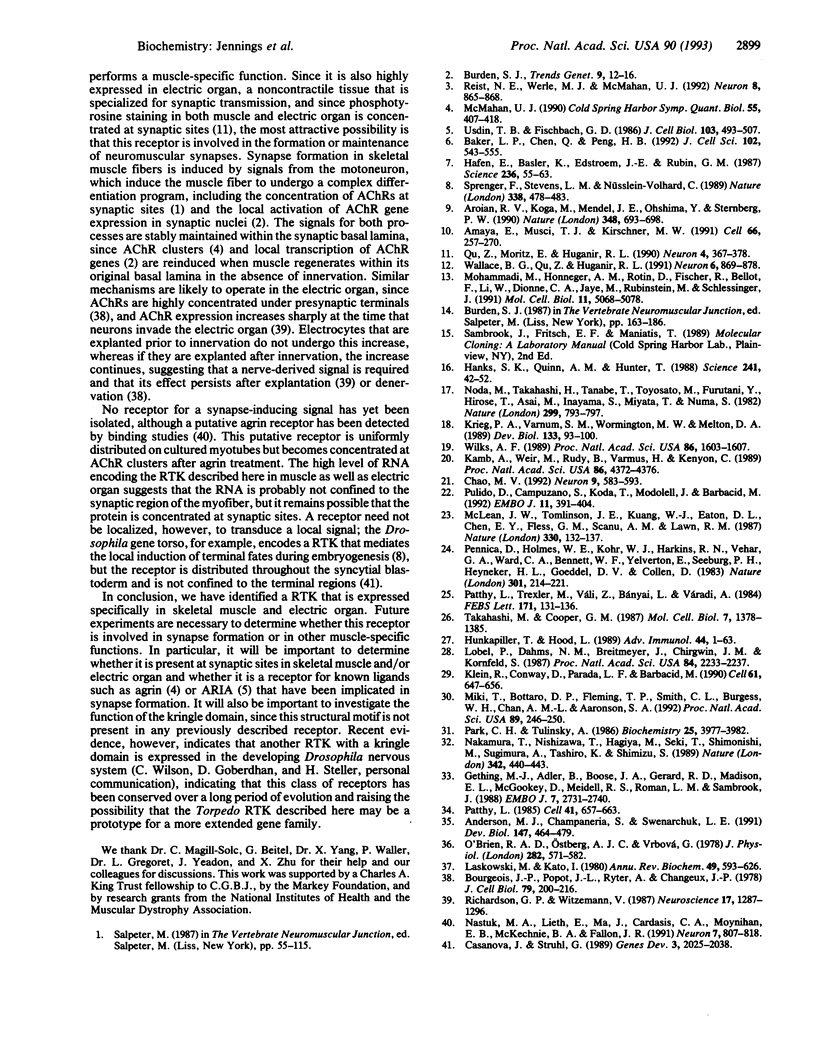
Images in this article
Selected References
These references are in PubMed. This may not be the complete list of references from this article.
- Amaya E., Musci T. J., Kirschner M. W. Expression of a dominant negative mutant of the FGF receptor disrupts mesoderm formation in Xenopus embryos. Cell. 1991 Jul 26;66(2):257–270. doi: 10.1016/0092-8674(91)90616-7. [DOI] [PubMed] [Google Scholar]
- Anderson M. J., Champaneria S., Swenarchuk L. E. Synaptic differentiation can be evoked by polymer microbeads that mimic localized pericellular proteolysis by removing proteins from adjacent surfaces. Dev Biol. 1991 Oct;147(2):464–479. doi: 10.1016/0012-1606(91)90305-m. [DOI] [PubMed] [Google Scholar]
- Aroian R. V., Koga M., Mendel J. E., Ohshima Y., Sternberg P. W. The let-23 gene necessary for Caenorhabditis elegans vulval induction encodes a tyrosine kinase of the EGF receptor subfamily. Nature. 1990 Dec 20;348(6303):693–699. doi: 10.1038/348693a0. [DOI] [PubMed] [Google Scholar]
- Baker L. P., Chen Q., Peng H. B. Induction of acetylcholine receptor clustering by native polystyrene beads. Implication of an endogenous muscle-derived signalling system. J Cell Sci. 1992 Jul;102(Pt 3):543–555. doi: 10.1242/jcs.102.3.543. [DOI] [PubMed] [Google Scholar]
- Bourgeois J. P., Popot J. L., Ryter A., Changeux J. P. Quantitative studies on the localization of the cholinergic receptor protein in the normal and denervated electroplaque from Electrophorus electricus. J Cell Biol. 1978 Oct;79(1):200–216. doi: 10.1083/jcb.79.1.200. [DOI] [PMC free article] [PubMed] [Google Scholar]
- Casanova J., Struhl G. Localized surface activity of torso, a receptor tyrosine kinase, specifies terminal body pattern in Drosophila. Genes Dev. 1989 Dec;3(12B):2025–2038. doi: 10.1101/gad.3.12b.2025. [DOI] [PubMed] [Google Scholar]
- Chao M. V. Neurotrophin receptors: a window into neuronal differentiation. Neuron. 1992 Oct;9(4):583–593. doi: 10.1016/0896-6273(92)90023-7. [DOI] [PubMed] [Google Scholar]
- Gething M. J., Adler B., Boose J. A., Gerard R. D., Madison E. L., McGookey D., Meidell R. S., Roman L. M., Sambrook J. Variants of human tissue-type plasminogen activator that lack specific structural domains of the heavy chain. EMBO J. 1988 Sep;7(9):2731–2740. doi: 10.1002/j.1460-2075.1988.tb03127.x. [DOI] [PMC free article] [PubMed] [Google Scholar]
- Hafen E., Basler K., Edstroem J. E., Rubin G. M. Sevenless, a cell-specific homeotic gene of Drosophila, encodes a putative transmembrane receptor with a tyrosine kinase domain. Science. 1987 Apr 3;236(4797):55–63. doi: 10.1126/science.2882603. [DOI] [PubMed] [Google Scholar]
- Hanks S. K., Quinn A. M., Hunter T. The protein kinase family: conserved features and deduced phylogeny of the catalytic domains. Science. 1988 Jul 1;241(4861):42–52. doi: 10.1126/science.3291115. [DOI] [PubMed] [Google Scholar]
- Hunkapiller T., Hood L. Diversity of the immunoglobulin gene superfamily. Adv Immunol. 1989;44:1–63. doi: 10.1016/s0065-2776(08)60639-2. [DOI] [PubMed] [Google Scholar]
- Kamb A., Weir M., Rudy B., Varmus H., Kenyon C. Identification of genes from pattern formation, tyrosine kinase, and potassium channel families by DNA amplification. Proc Natl Acad Sci U S A. 1989 Jun;86(12):4372–4376. doi: 10.1073/pnas.86.12.4372. [DOI] [PMC free article] [PubMed] [Google Scholar]
- Klein R., Conway D., Parada L. F., Barbacid M. The trkB tyrosine protein kinase gene codes for a second neurogenic receptor that lacks the catalytic kinase domain. Cell. 1990 May 18;61(4):647–656. doi: 10.1016/0092-8674(90)90476-u. [DOI] [PubMed] [Google Scholar]
- Krieg P. A., Varnum S. M., Wormington W. M., Melton D. A. The mRNA encoding elongation factor 1-alpha (EF-1 alpha) is a major transcript at the midblastula transition in Xenopus. Dev Biol. 1989 May;133(1):93–100. doi: 10.1016/0012-1606(89)90300-x. [DOI] [PubMed] [Google Scholar]
- Laskowski M., Jr, Kato I. Protein inhibitors of proteinases. Annu Rev Biochem. 1980;49:593–626. doi: 10.1146/annurev.bi.49.070180.003113. [DOI] [PubMed] [Google Scholar]
- Lobel P., Dahms N. M., Breitmeyer J., Chirgwin J. M., Kornfeld S. Cloning of the bovine 215-kDa cation-independent mannose 6-phosphate receptor. Proc Natl Acad Sci U S A. 1987 Apr;84(8):2233–2237. doi: 10.1073/pnas.84.8.2233. [DOI] [PMC free article] [PubMed] [Google Scholar]
- McLean J. W., Tomlinson J. E., Kuang W. J., Eaton D. L., Chen E. Y., Fless G. M., Scanu A. M., Lawn R. M. cDNA sequence of human apolipoprotein(a) is homologous to plasminogen. Nature. 1987 Nov 12;330(6144):132–137. doi: 10.1038/330132a0. [DOI] [PubMed] [Google Scholar]
- McMahan U. J. The agrin hypothesis. Cold Spring Harb Symp Quant Biol. 1990;55:407–418. doi: 10.1101/sqb.1990.055.01.041. [DOI] [PubMed] [Google Scholar]
- Miki T., Bottaro D. P., Fleming T. P., Smith C. L., Burgess W. H., Chan A. M., Aaronson S. A. Determination of ligand-binding specificity by alternative splicing: two distinct growth factor receptors encoded by a single gene. Proc Natl Acad Sci U S A. 1992 Jan 1;89(1):246–250. doi: 10.1073/pnas.89.1.246. [DOI] [PMC free article] [PubMed] [Google Scholar]
- Mohammadi M., Honegger A. M., Rotin D., Fischer R., Bellot F., Li W., Dionne C. A., Jaye M., Rubinstein M., Schlessinger J. A tyrosine-phosphorylated carboxy-terminal peptide of the fibroblast growth factor receptor (Flg) is a binding site for the SH2 domain of phospholipase C-gamma 1. Mol Cell Biol. 1991 Oct;11(10):5068–5078. doi: 10.1128/mcb.11.10.5068. [DOI] [PMC free article] [PubMed] [Google Scholar]
- Nakamura T., Nishizawa T., Hagiya M., Seki T., Shimonishi M., Sugimura A., Tashiro K., Shimizu S. Molecular cloning and expression of human hepatocyte growth factor. Nature. 1989 Nov 23;342(6248):440–443. doi: 10.1038/342440a0. [DOI] [PubMed] [Google Scholar]
- Nastuk M. A., Lieth E., Ma J. Y., Cardasis C. A., Moynihan E. B., McKechnie B. A., Fallon J. R. The putative agrin receptor binds ligand in a calcium-dependent manner and aggregates during agrin-induced acetylcholine receptor clustering. Neuron. 1991 Nov;7(5):807–818. doi: 10.1016/0896-6273(91)90283-6. [DOI] [PubMed] [Google Scholar]
- Noda M., Takahashi H., Tanabe T., Toyosato M., Furutani Y., Hirose T., Asai M., Inayama S., Miyata T., Numa S. Primary structure of alpha-subunit precursor of Torpedo californica acetylcholine receptor deduced from cDNA sequence. Nature. 1982 Oct 28;299(5886):793–797. doi: 10.1038/299793a0. [DOI] [PubMed] [Google Scholar]
- O'Brien R. A., Ostberg A. J., Vrbová G. Observations on the elimination of polyneuronal innervation in developing mammalian skeletal muscle. J Physiol. 1978 Sep;282:571–582. doi: 10.1113/jphysiol.1978.sp012482. [DOI] [PMC free article] [PubMed] [Google Scholar]
- Park C. H., Tulinsky A. Three-dimensional structure of the kringle sequence: structure of prothrombin fragment 1. Biochemistry. 1986 Jul 15;25(14):3977–3982. doi: 10.1021/bi00362a001. [DOI] [PubMed] [Google Scholar]
- Patthy L. Evolution of the proteases of blood coagulation and fibrinolysis by assembly from modules. Cell. 1985 Jul;41(3):657–663. doi: 10.1016/s0092-8674(85)80046-5. [DOI] [PubMed] [Google Scholar]
- Patthy L., Trexler M., Váli Z., Bányai L., Váradi A. Kringles: modules specialized for protein binding. Homology of the gelatin-binding region of fibronectin with the kringle structures of proteases. FEBS Lett. 1984 Jun 4;171(1):131–136. doi: 10.1016/0014-5793(84)80473-1. [DOI] [PubMed] [Google Scholar]
- Pennica D., Holmes W. E., Kohr W. J., Harkins R. N., Vehar G. A., Ward C. A., Bennett W. F., Yelverton E., Seeburg P. H., Heyneker H. L. Cloning and expression of human tissue-type plasminogen activator cDNA in E. coli. Nature. 1983 Jan 20;301(5897):214–221. doi: 10.1038/301214a0. [DOI] [PubMed] [Google Scholar]
- Pulido D., Campuzano S., Koda T., Modolell J., Barbacid M. Dtrk, a Drosophila gene related to the trk family of neurotrophin receptors, encodes a novel class of neural cell adhesion molecule. EMBO J. 1992 Feb;11(2):391–404. doi: 10.1002/j.1460-2075.1992.tb05067.x. [DOI] [PMC free article] [PubMed] [Google Scholar]
- Qu Z. C., Moritz E., Huganir R. L. Regulation of tyrosine phosphorylation of the nicotinic acetylcholine receptor at the rat neuromuscular junction. Neuron. 1990 Mar;4(3):367–378. doi: 10.1016/0896-6273(90)90049-l. [DOI] [PubMed] [Google Scholar]
- Reist N. E., Werle M. J., McMahan U. J. Agrin released by motor neurons induces the aggregation of acetylcholine receptors at neuromuscular junctions. Neuron. 1992 May;8(5):865–868. doi: 10.1016/0896-6273(92)90200-w. [DOI] [PubMed] [Google Scholar]
- Richardson G. P., Witzemann V. Torpedo electromotor system development: biochemical differentiation of Torpedo electrocytes in vitro. Neuroscience. 1986 Apr;17(4):1287–1296. doi: 10.1016/0306-4522(86)90095-3. [DOI] [PubMed] [Google Scholar]
- Sprenger F., Stevens L. M., Nüsslein-Volhard C. The Drosophila gene torso encodes a putative receptor tyrosine kinase. Nature. 1989 Apr 6;338(6215):478–483. doi: 10.1038/338478a0. [DOI] [PubMed] [Google Scholar]
- Takahashi M., Cooper G. M. ret transforming gene encodes a fusion protein homologous to tyrosine kinases. Mol Cell Biol. 1987 Apr;7(4):1378–1385. doi: 10.1128/mcb.7.4.1378. [DOI] [PMC free article] [PubMed] [Google Scholar]
- Usdin T. B., Fischbach G. D. Purification and characterization of a polypeptide from chick brain that promotes the accumulation of acetylcholine receptors in chick myotubes. J Cell Biol. 1986 Aug;103(2):493–507. doi: 10.1083/jcb.103.2.493. [DOI] [PMC free article] [PubMed] [Google Scholar]
- Wallace B. G., Qu Z., Huganir R. L. Agrin induces phosphorylation of the nicotinic acetylcholine receptor. Neuron. 1991 Jun;6(6):869–878. doi: 10.1016/0896-6273(91)90227-q. [DOI] [PubMed] [Google Scholar]
- Wilks A. F. Two putative protein-tyrosine kinases identified by application of the polymerase chain reaction. Proc Natl Acad Sci U S A. 1989 Mar;86(5):1603–1607. doi: 10.1073/pnas.86.5.1603. [DOI] [PMC free article] [PubMed] [Google Scholar]