Abstract
We have done a systematic study on the contribution of the single-stranded NCCA end (where N is any nucleotide) to the stability of the aminoacyl stem of tRNA. A 7-bp RNA duplex with the single-strand ACCA 3' terminus derived from the aminoacyl stem of Escherichia coli tRNA(Ala) and several chemically synthesized sequence variants are characterized by proton NMR and thermodynamic parameters. The single-stranded 3' terminus noticeably stabilizes the duplex in a sequence-dependent manner. Though the largest contribution to the stability gain due to the ACCA end is provided by the first dangling 3' nucleotide, the influence of even the fourth nucleotide is measurable. The nature of the N73 discriminator base influences the stem structure and stability, which may be important for the recognition of tRNA by aminoacyl-tRNA synthetase. The stepwise attachment of the nucleotides to the 3' tail improves the stacking of the unpaired bases over the helix stem. Hence, the ACCA end appears to be structured. Replacing Mg2+ with Mn2+ causes broadening of certain imino proton peaks in the NMR spectrum, indicating a specific divalent metal ion binding site in the vicinity of the major identity element of the duplex (G3-U70) that is required for its recognition by the Ala-tRNA synthetase.
Full text
PDF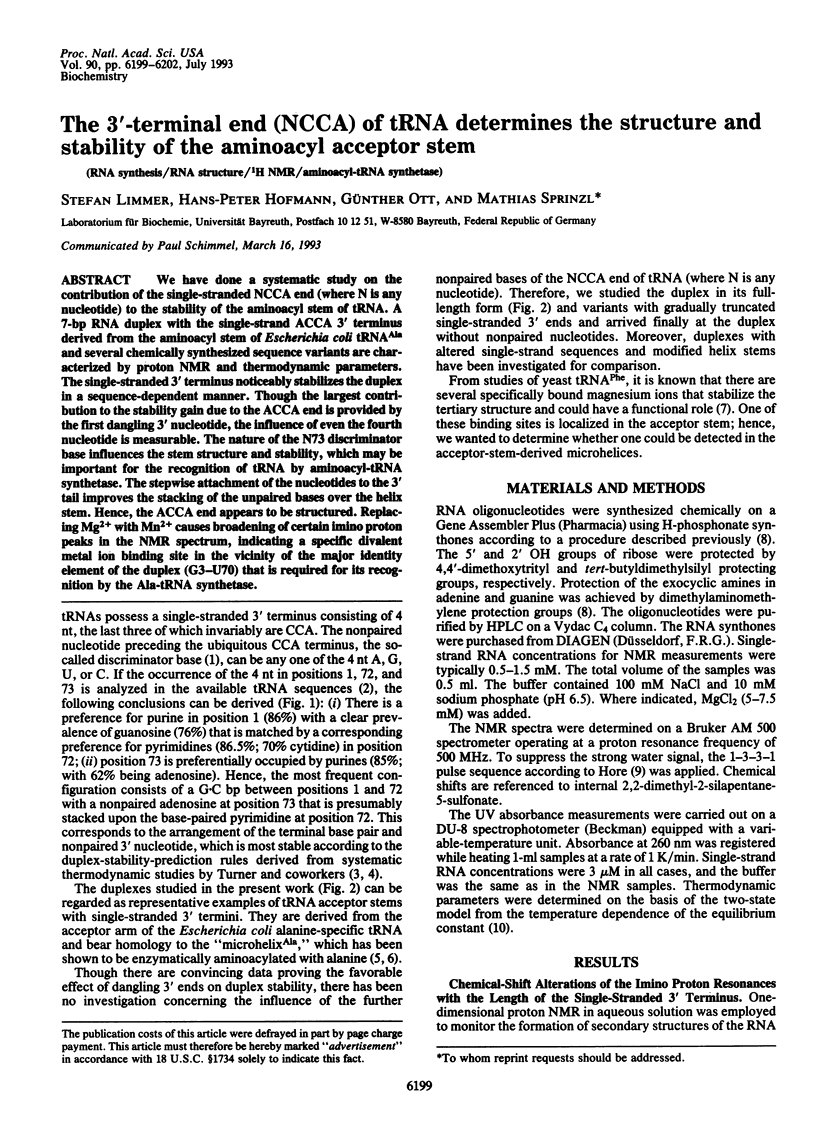
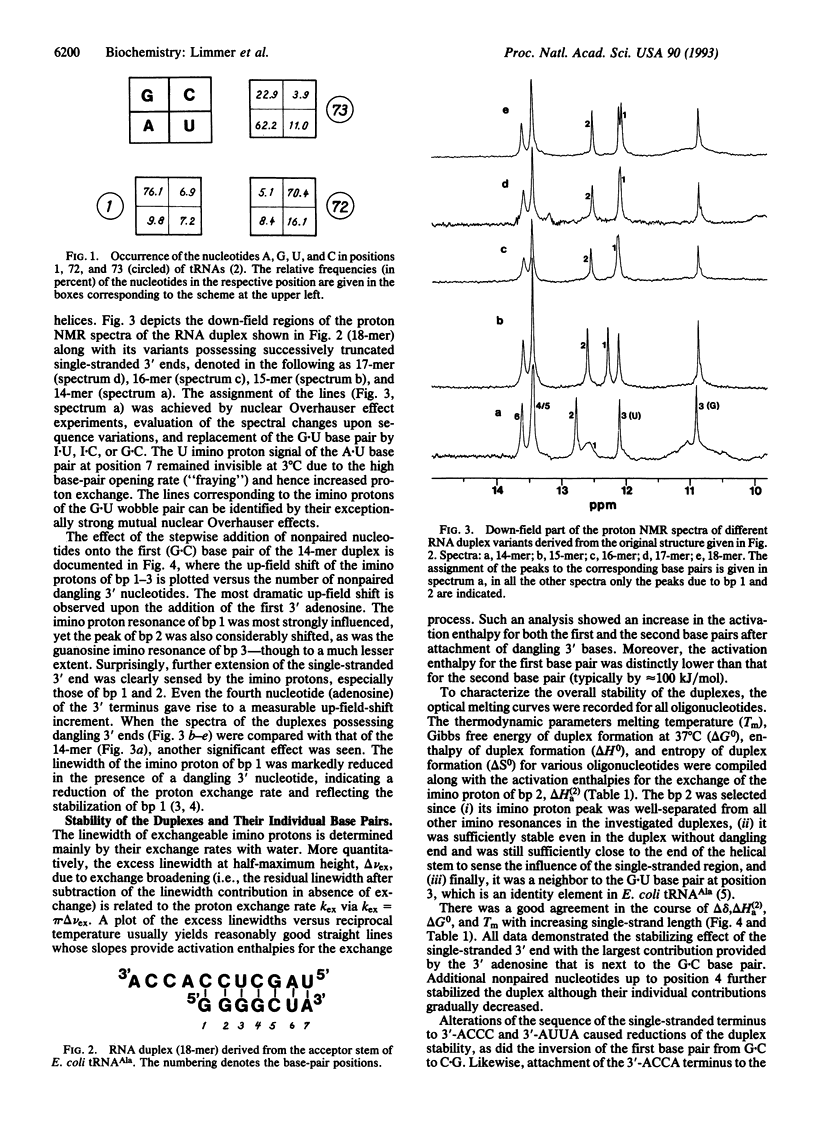
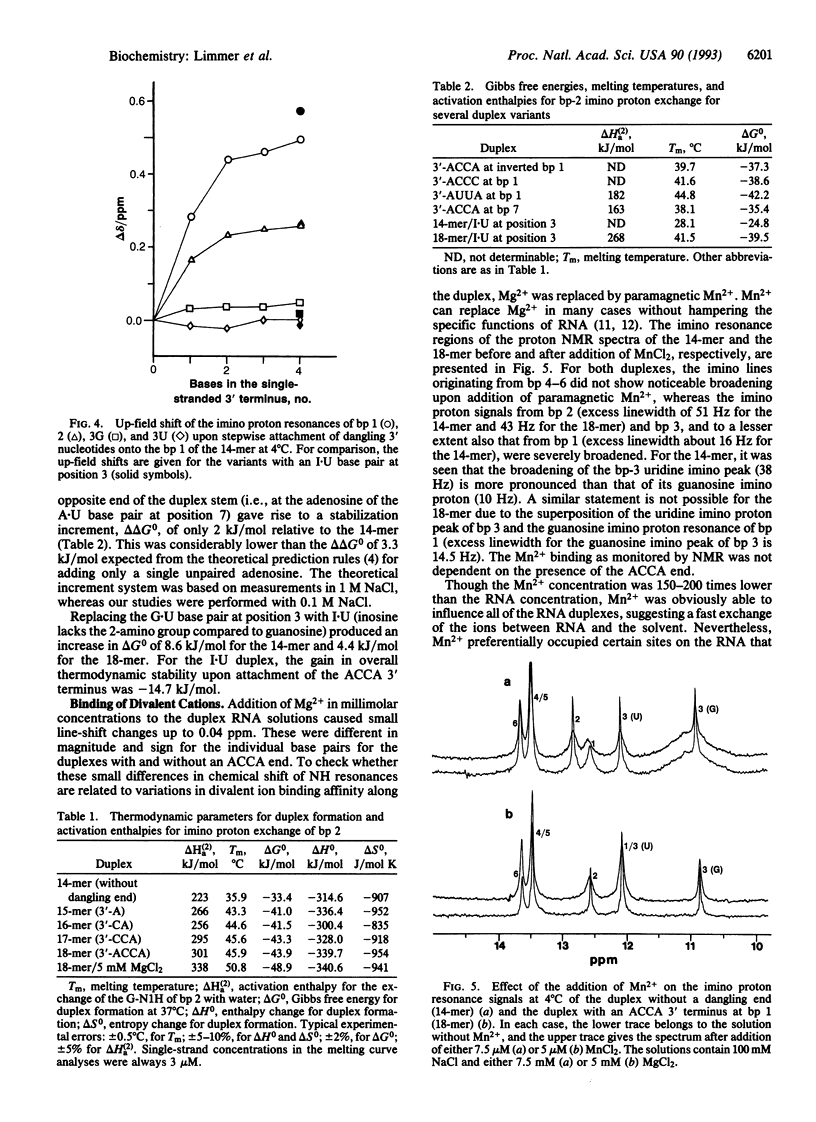
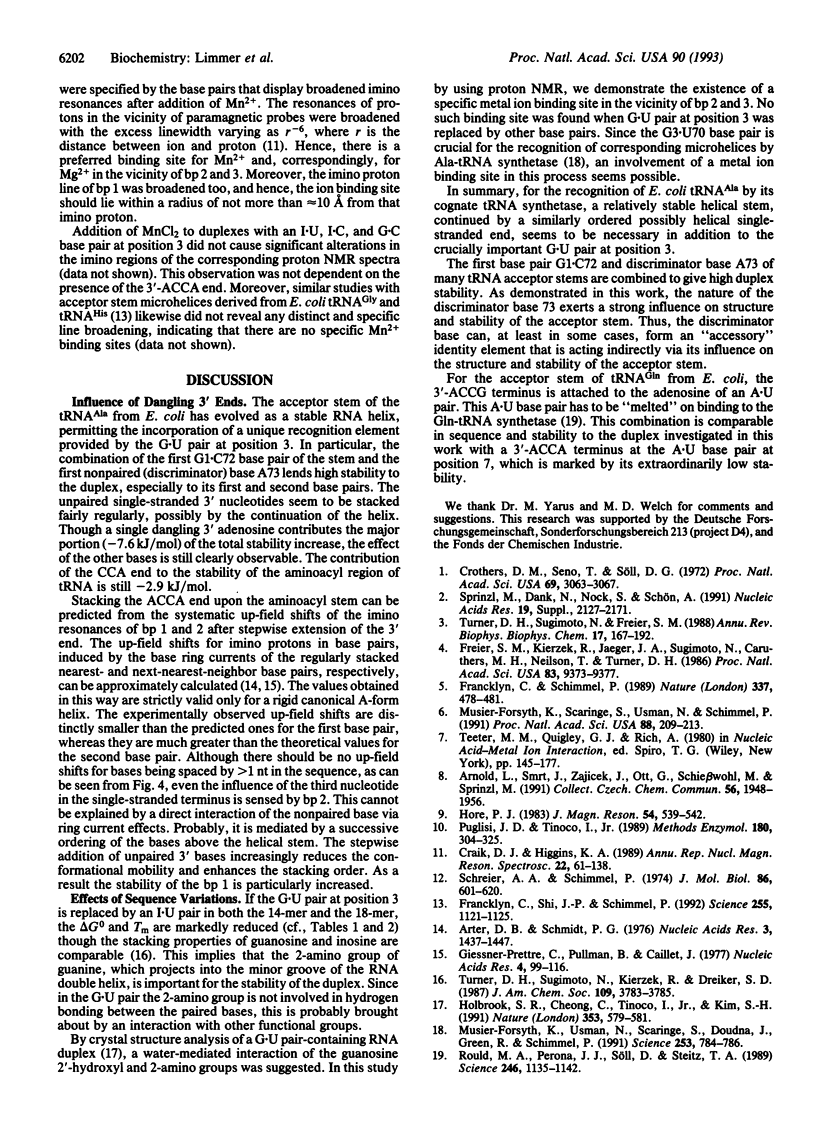
Selected References
These references are in PubMed. This may not be the complete list of references from this article.
- Arter D. B., Schmidt P. G. Ring current shielding effects in nucleic acid double helices. Nucleic Acids Res. 1976 Jun;3(6):1437–1447. doi: 10.1093/nar/3.6.1437. [DOI] [PMC free article] [PubMed] [Google Scholar]
- Crothers D. M., Seno T., Söll G. Is there a discriminator site in transfer RNA? Proc Natl Acad Sci U S A. 1972 Oct;69(10):3063–3067. doi: 10.1073/pnas.69.10.3063. [DOI] [PMC free article] [PubMed] [Google Scholar]
- Francklyn C., Schimmel P. Aminoacylation of RNA minihelices with alanine. Nature. 1989 Feb 2;337(6206):478–481. doi: 10.1038/337478a0. [DOI] [PubMed] [Google Scholar]
- Francklyn C., Shi J. P., Schimmel P. Overlapping nucleotide determinants for specific aminoacylation of RNA microhelices. Science. 1992 Feb 28;255(5048):1121–1125. doi: 10.1126/science.1546312. [DOI] [PubMed] [Google Scholar]
- Freier S. M., Kierzek R., Jaeger J. A., Sugimoto N., Caruthers M. H., Neilson T., Turner D. H. Improved free-energy parameters for predictions of RNA duplex stability. Proc Natl Acad Sci U S A. 1986 Dec;83(24):9373–9377. doi: 10.1073/pnas.83.24.9373. [DOI] [PMC free article] [PubMed] [Google Scholar]
- Giessner-Prettre C., Pullman B., Caillet J. Theoretical study on the proton chemical shifts of hydrogen bonded nucleic acid bases. Nucleic Acids Res. 1977 Jan;4(1):99–116. doi: 10.1093/nar/4.1.99. [DOI] [PMC free article] [PubMed] [Google Scholar]
- Holbrook S. R., Cheong C., Tinoco I., Jr, Kim S. H. Crystal structure of an RNA double helix incorporating a track of non-Watson-Crick base pairs. Nature. 1991 Oct 10;353(6344):579–581. doi: 10.1038/353579a0. [DOI] [PubMed] [Google Scholar]
- Musier-Forsyth K., Scaringe S., Usman N., Schimmel P. Enzymatic aminoacylation of single-stranded RNA with an RNA cofactor. Proc Natl Acad Sci U S A. 1991 Jan 1;88(1):209–213. doi: 10.1073/pnas.88.1.209. [DOI] [PMC free article] [PubMed] [Google Scholar]
- Musier-Forsyth K., Usman N., Scaringe S., Doudna J., Green R., Schimmel P. Specificity for aminoacylation of an RNA helix: an unpaired, exocyclic amino group in the minor groove. Science. 1991 Aug 16;253(5021):784–786. doi: 10.1126/science.1876835. [DOI] [PubMed] [Google Scholar]
- Puglisi J. D., Tinoco I., Jr Absorbance melting curves of RNA. Methods Enzymol. 1989;180:304–325. doi: 10.1016/0076-6879(89)80108-9. [DOI] [PubMed] [Google Scholar]
- Rould M. A., Perona J. J., Söll D., Steitz T. A. Structure of E. coli glutaminyl-tRNA synthetase complexed with tRNA(Gln) and ATP at 2.8 A resolution. Science. 1989 Dec 1;246(4934):1135–1142. doi: 10.1126/science.2479982. [DOI] [PubMed] [Google Scholar]
- Schreier A. A., Schimmel P. R. Interaction of manganese with fragments, complementary fragment recombinations, and whole molecules of yeast phenylalanine specific transfer RNA. J Mol Biol. 1974 Jul 5;86(3):601–620. doi: 10.1016/0022-2836(74)90183-1. [DOI] [PubMed] [Google Scholar]
- Sprinzl M., Dank N., Nock S., Schön A. Compilation of tRNA sequences and sequences of tRNA genes. Nucleic Acids Res. 1991 Apr 25;19 (Suppl):2127–2171. doi: 10.1093/nar/19.suppl.2127. [DOI] [PMC free article] [PubMed] [Google Scholar]
- Turner D. H., Sugimoto N., Freier S. M. RNA structure prediction. Annu Rev Biophys Biophys Chem. 1988;17:167–192. doi: 10.1146/annurev.bb.17.060188.001123. [DOI] [PubMed] [Google Scholar]