Abstract
We used tryptophan auxotrophs of the dicot Arabidopsis thaliana (wall cress) to determine whether tryptophan has the capacity to serve as a precursor to the auxin, indole-3-acetic acid (IAA). Quantitative gas chromatography-selected ion monitoring-mass spectrometry (GC-SIM-MS) revealed that the trp2-1 mutant, which is defective in the conversion of indole to tryptophan, accumulated amide- and ester-linked IAA at levels 38-fold and 19-fold, respectively, above those of the wild type. Tryptophan and free IAA were isolated from the trp2-1 mutant grown in the presence of [15N]anthranilate and [2H5]tryptophan, and the relative 15N and 2H5 enrichments of tryptophan and IAA were determined via GC-SIM-MS. The 15N enrichment of tryptophan, 13% +/- 4%, was less than the 15N enrichment of the IAA pool, 39% +/- 4%; therefore, IAA biosynthesis occurs via a tryptophan-independent pathway. The amount of 2H5 incorporated by the plant into IAA from tryptophan (9% +/- 4%) was low and only slightly above the level of spontaneous, nonenzymatic conversion of [2H5]tryptophan to [2H5]IAA. These results show that the dicot Arabidopsis is similar to the monocot Zea mays in that the major route of IAA biosynthesis does not occur through tryptophan.
Full text
PDF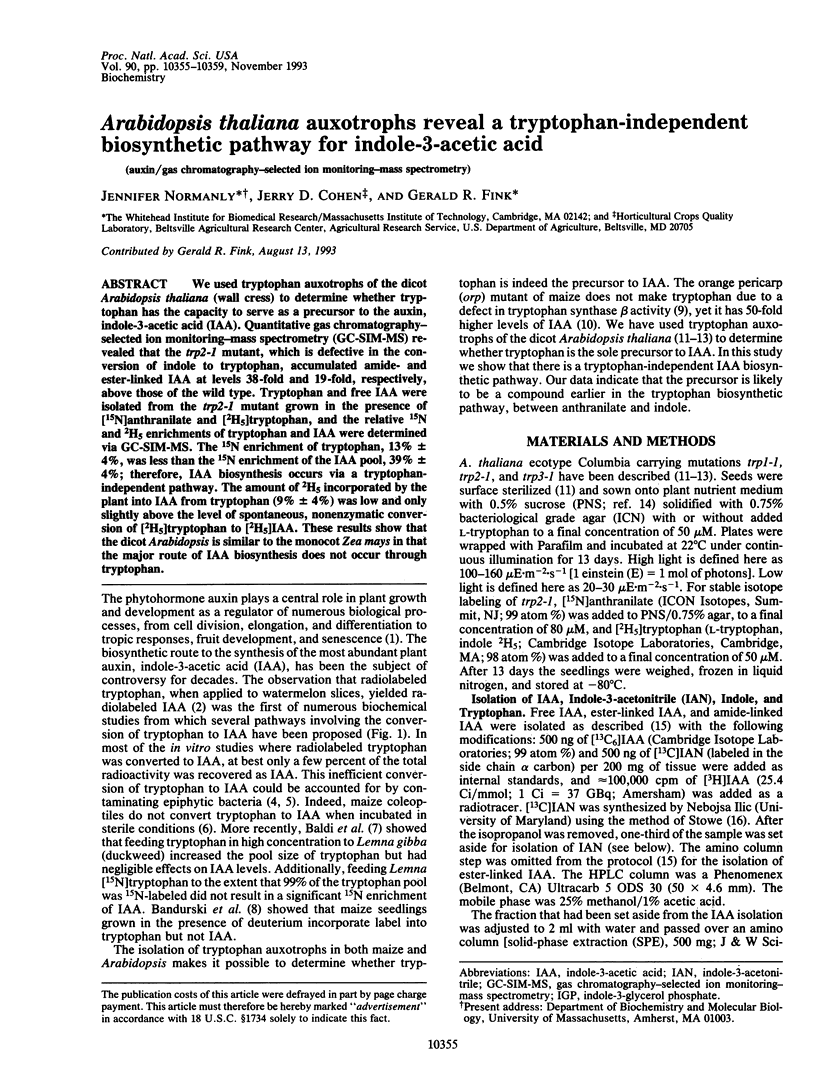
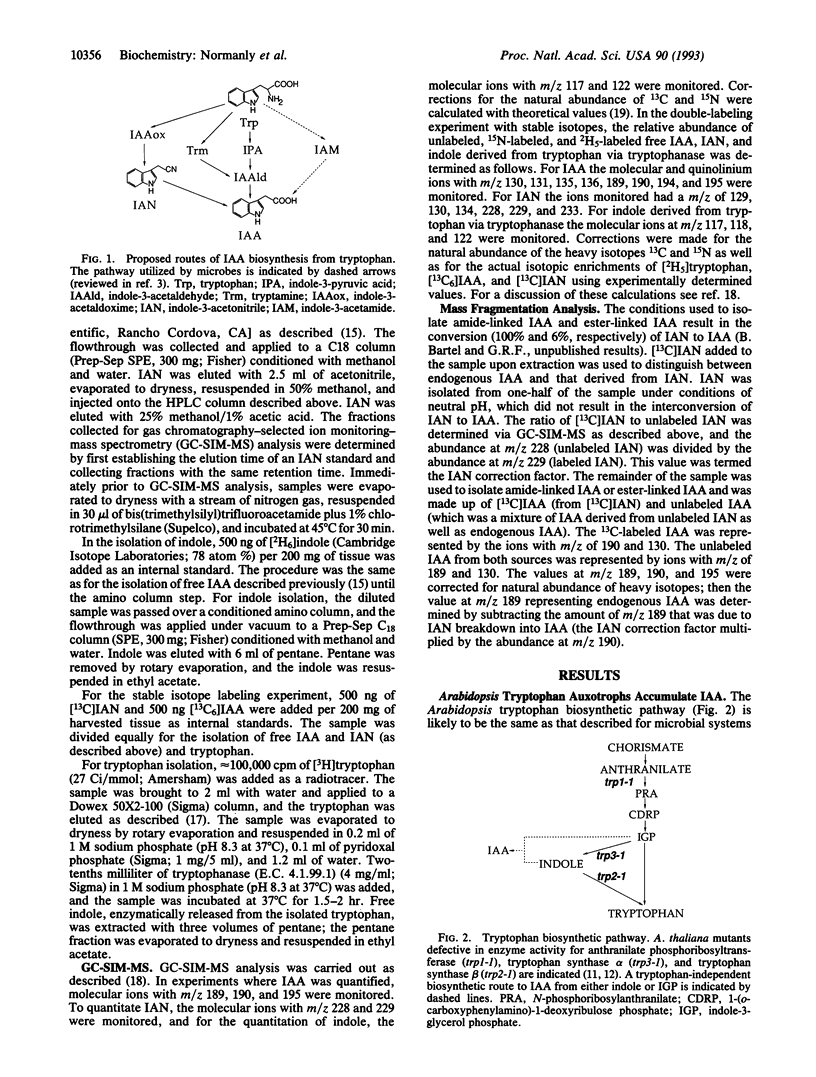
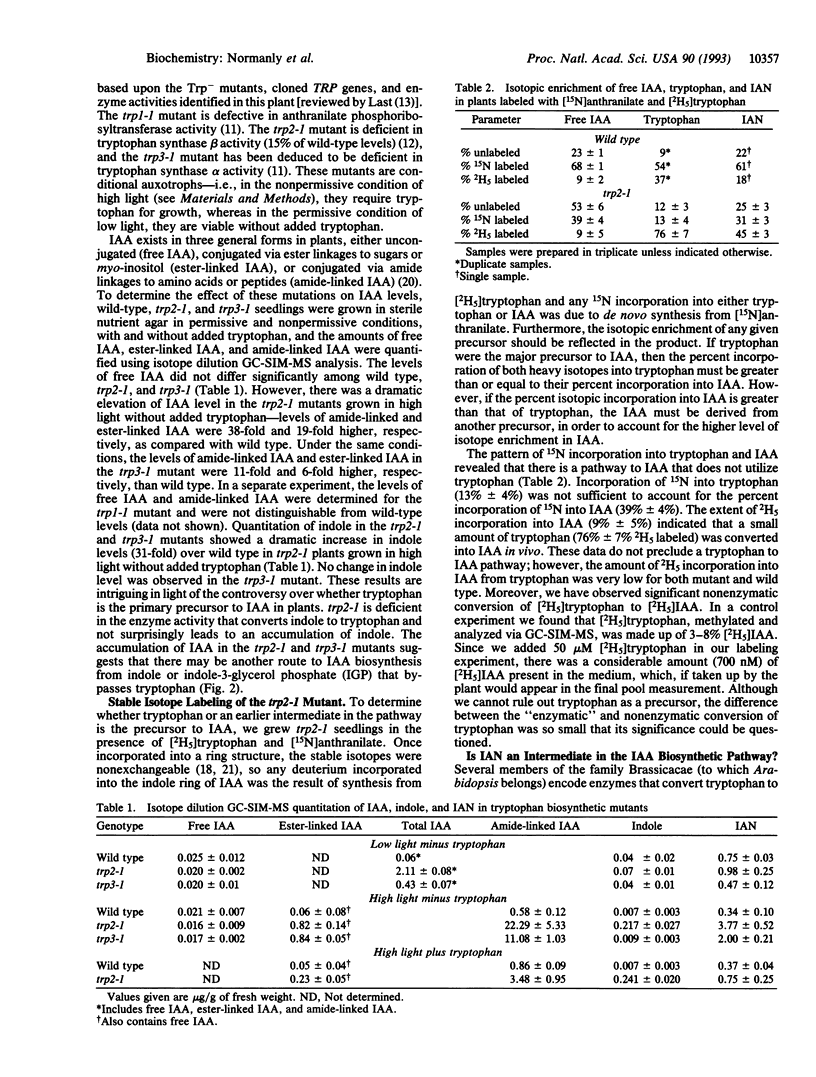
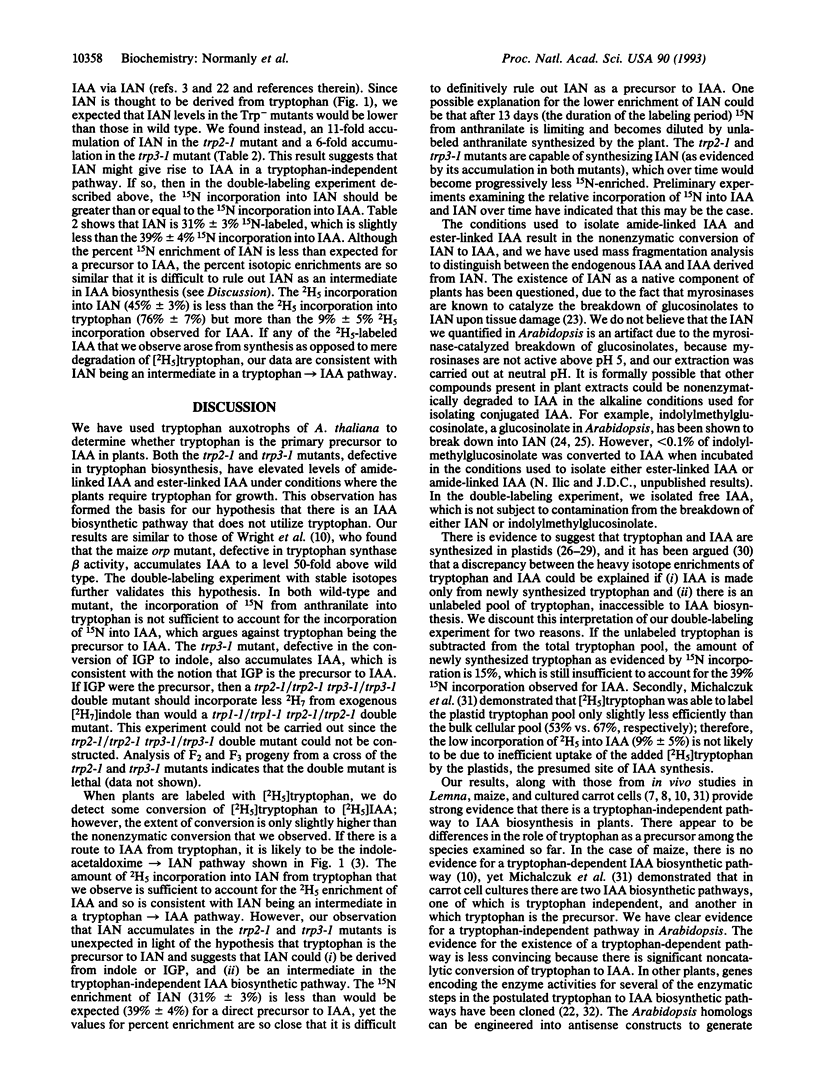
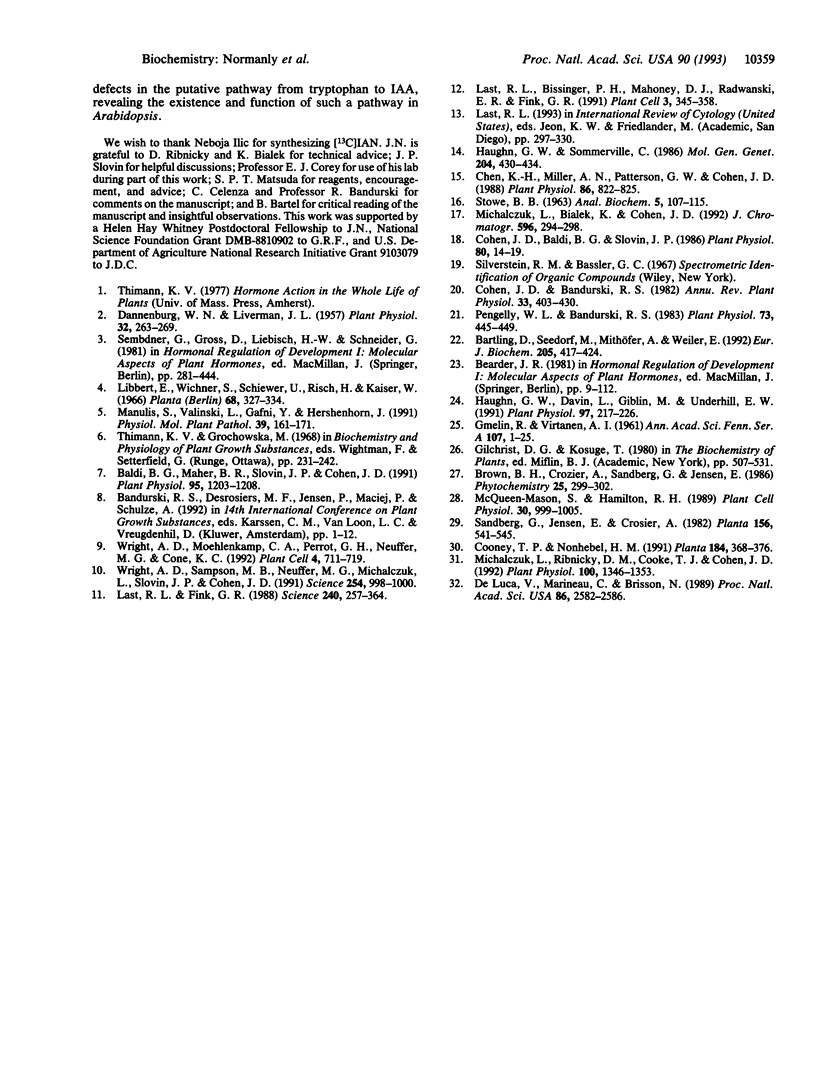
Selected References
These references are in PubMed. This may not be the complete list of references from this article.
- Baldi B. G., Maher B. R., Slovin J. P., Cohen J. D. Stable Isotope Labeling, in Vivo, of d- and l-Tryptophan Pools in Lemna gibba and the Low Incorporation of Label into Indole-3-Acetic Acid. Plant Physiol. 1991 Apr;95(4):1203–1208. doi: 10.1104/pp.95.4.1203. [DOI] [PMC free article] [PubMed] [Google Scholar]
- Bartling D., Seedorf M., Mithöfer A., Weiler E. W. Cloning and expression of an Arabidopsis nitrilase which can convert indole-3-acetonitrile to the plant hormone, indole-3-acetic acid. Eur J Biochem. 1992 Apr 1;205(1):417–424. doi: 10.1111/j.1432-1033.1992.tb16795.x. [DOI] [PubMed] [Google Scholar]
- Chen K. H., Miller A. N., Patterson G. W., Cohen J. D. A Rapid and Simple Procedure for Purification of Indole-3-Acetic Acid Prior to GC-SIM-MS Analysis. Plant Physiol. 1988 Mar;86(3):822–825. doi: 10.1104/pp.86.3.822. [DOI] [PMC free article] [PubMed] [Google Scholar]
- Cohen J. D., Baldi B. G., Slovin J. P. C(6)-[benzene ring]-indole-3-acetic Acid: a new internal standard for quantitative mass spectral analysis of indole-3-acetic Acid in plants. Plant Physiol. 1986 Jan;80(1):14–19. doi: 10.1104/pp.80.1.14. [DOI] [PMC free article] [PubMed] [Google Scholar]
- Dannenburg W. N., Liverman J. L. Conversion of Tryptophan-2-C to Indoleacetic Acid by Watermelon Tissue Slices. Plant Physiol. 1957 Jul;32(4):263–269. doi: 10.1104/pp.32.4.263. [DOI] [PMC free article] [PubMed] [Google Scholar]
- De Luca V., Marineau C., Brisson N. Molecular cloning and analysis of cDNA encoding a plant tryptophan decarboxylase: comparison with animal dopa decarboxylases. Proc Natl Acad Sci U S A. 1989 Apr;86(8):2582–2586. doi: 10.1073/pnas.86.8.2582. [DOI] [PMC free article] [PubMed] [Google Scholar]
- Haughn G. W., Davin L., Giblin M., Underhill E. W. Biochemical Genetics of Plant Secondary Metabolites in Arabidopsis thaliana: The Glucosinolates. Plant Physiol. 1991 Sep;97(1):217–226. doi: 10.1104/pp.97.1.217. [DOI] [PMC free article] [PubMed] [Google Scholar]
- Last R. L., Bissinger P. H., Mahoney D. J., Radwanski E. R., Fink G. R. Tryptophan mutants in Arabidopsis: the consequences of duplicated tryptophan synthase beta genes. Plant Cell. 1991 Apr;3(4):345–358. doi: 10.1105/tpc.3.4.345. [DOI] [PMC free article] [PubMed] [Google Scholar]
- Michalczuk L., Ribnicky D. M., Cooke T. J., Cohen J. D. Regulation of indole-3-acetic Acid biosynthetic pathways in carrot cell cultures. Plant Physiol. 1992 Nov;100(3):1346–1353. doi: 10.1104/pp.100.3.1346. [DOI] [PMC free article] [PubMed] [Google Scholar]
- Pengelly W. L., Bandurski R. S. Analysis of Indole-3-acetic Acid Metabolism in Zea mays Using Deuterium Oxide as a Tracer. Plant Physiol. 1983 Oct;73(2):445–449. doi: 10.1104/pp.73.2.445. [DOI] [PMC free article] [PubMed] [Google Scholar]
- STOWE B. B. Synthesis of high specific activity C14-carboxyl indoleacetic acid and of C14-nitrile indoleacetonitrile. Anal Biochem. 1963 Feb;5:107–115. doi: 10.1016/0003-2697(63)90017-4. [DOI] [PubMed] [Google Scholar]
- Wright A. D., Moehlenkamp C. A., Perrot G. H., Neuffer M. G., Cone K. C. The maize auxotrophic mutant orange pericarp is defective in duplicate genes for tryptophan synthase beta. Plant Cell. 1992 Jun;4(6):711–719. doi: 10.1105/tpc.4.6.711. [DOI] [PMC free article] [PubMed] [Google Scholar]
- Wright A. D., Sampson M. B., Neuffer M. G., Michalczuk L., Slovin J. P., Cohen J. D. Indole-3-Acetic Acid Biosynthesis in the Mutant Maize orange pericarp, a Tryptophan Auxotroph. Science. 1991 Nov 15;254(5034):998–1000. doi: 10.1126/science.254.5034.998. [DOI] [PubMed] [Google Scholar]