Abstract
We have used a combination of spectroscopic, calorimetric, and computational techniques to characterize the properties of nucleic acid complexes with 2',5''- and 3',5''-phosphodiester linkages. Specifically, we have compared the properties of complexes formed by the association of 3',5'' single-stranded 16-mers of adenylic acid (A16) and thymidylic acid (T16) with the complexes formed by the corresponding single-stranded 16-mers with 2',5''-phosphodiester linkages (A*16 and T*16). Our results reveal the following differential features: (i) the 3',5'' strands form either a duplex or a triplex, depending on the sodium ion concentration, whereas the 2',5'' strands form either a triplex or no complex at all; (ii) the 2',5'' and 3',5'' triplexes exhibit significantly different CD spectra, suggesting that the two triplex states are conformationally nonequivalent; (iii) the 2',5'' triplex has a lower charge density than the 3',5'' triplex; (iv) the thermal stability of the 3',5'' triplex, as expected, is concentration dependent, whereas the thermal stability of the 2',5'' triplex is concentration independent; (v) relative to their component single strands, the 2',5'' triplex is thermodynamically much less stable than the 3',5'' triplex, despite being thermally more stable; (vi) the reduced thermodynamic stability of the 2',5'' triplex relative to the 3',5'' triplex is overwhelmingly enthalpic in origin. In the aggregate, our results reveal and characterize significant differences in the properties of complexes formed by the association of strands with identical base sequences but different phosphodiester linkages. We describe a structural model that is consistent with many of the differential properties observed. We also speculate on how these differential properties may have provided an evolutionary advantage for 3',5'' linkages and how the properties of 2',5'' complexes might be exploited in antisense strategies.
Full text
PDF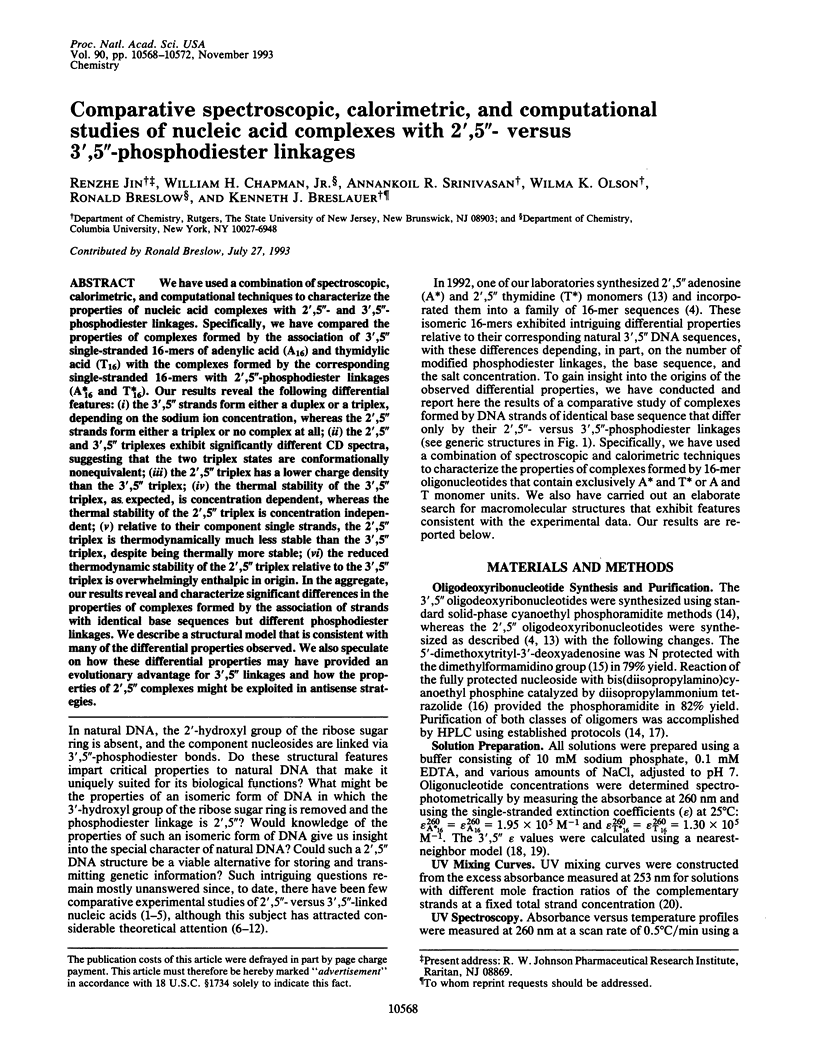
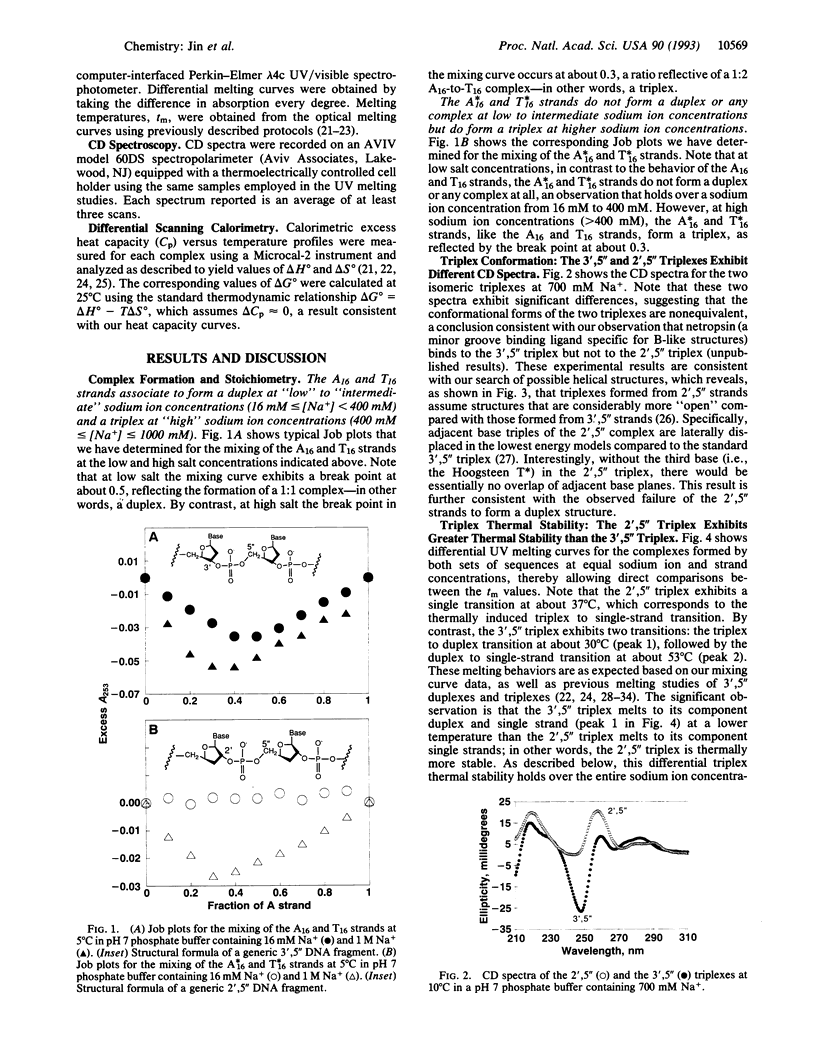
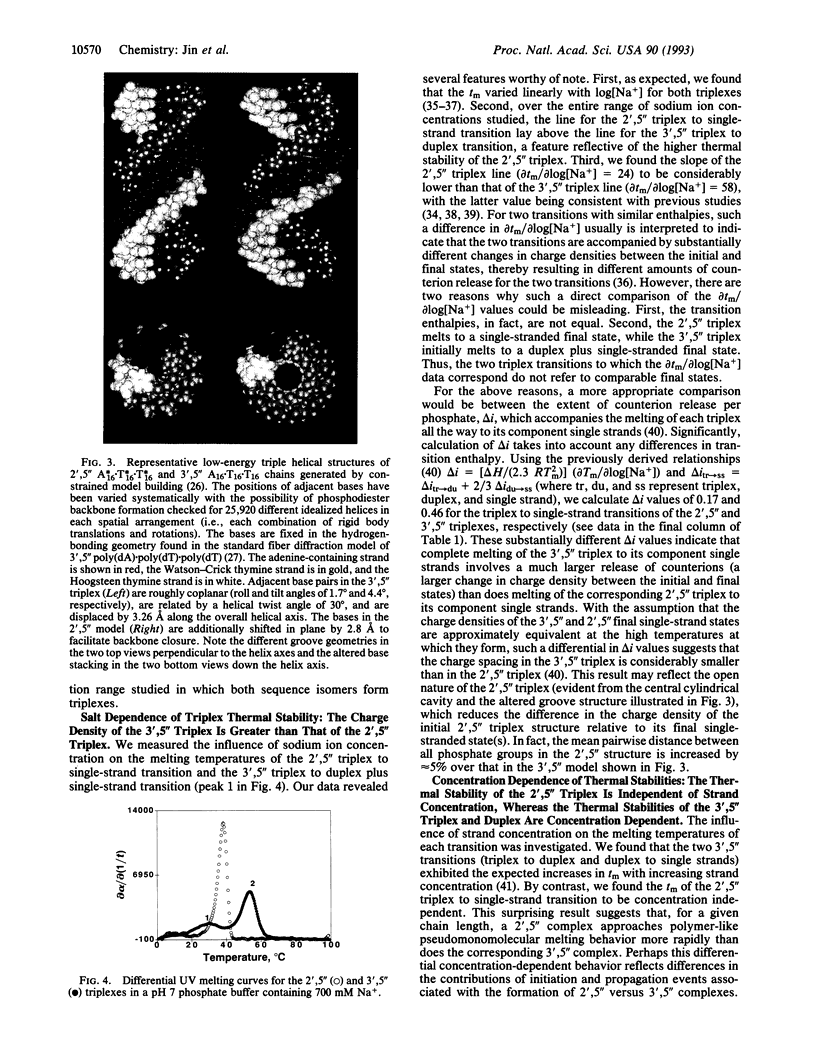
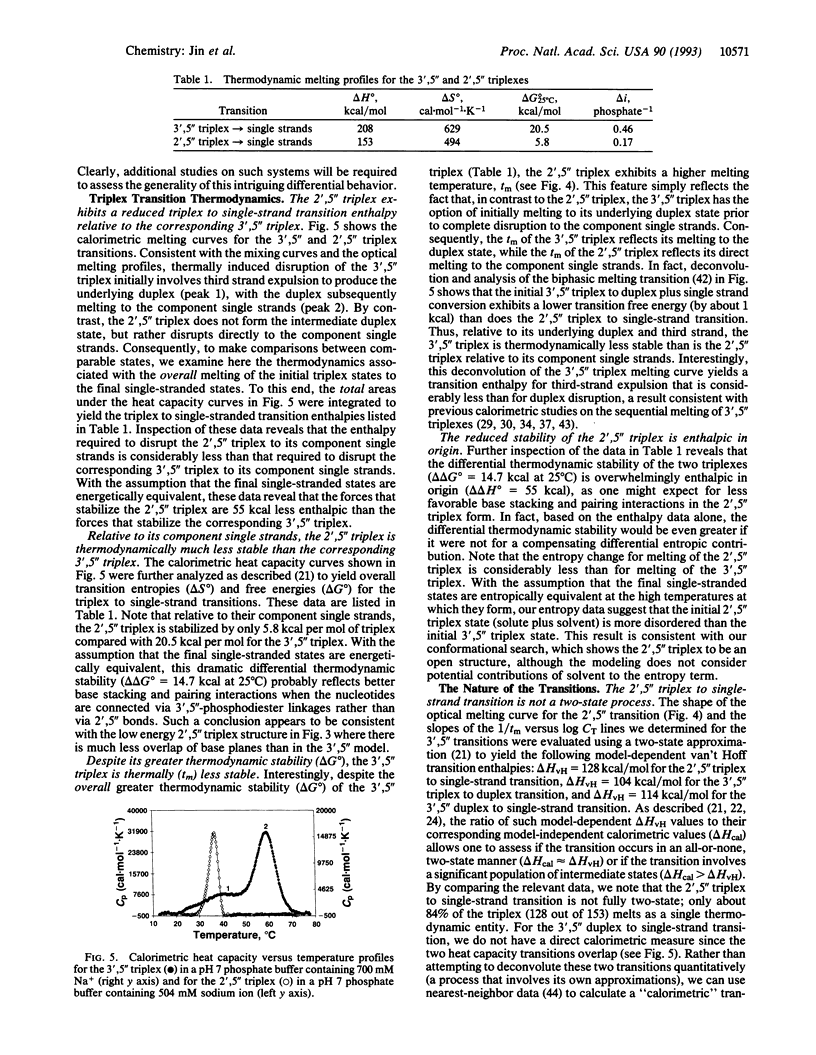
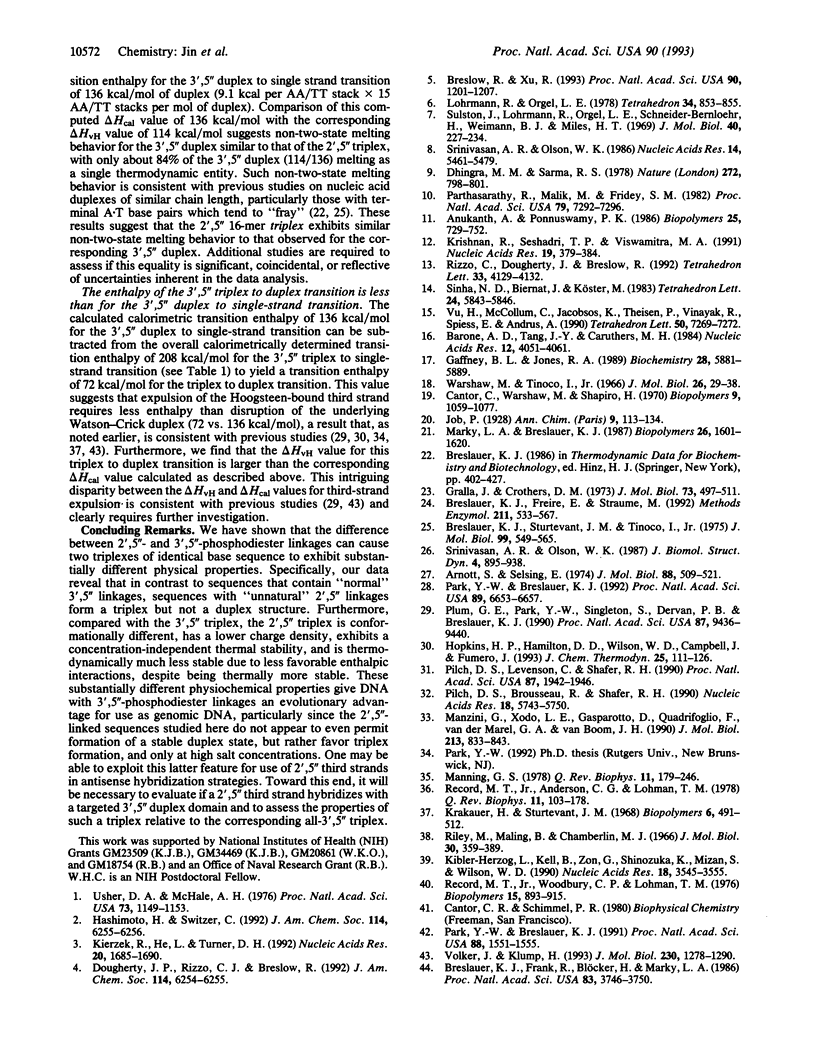
Images in this article
Selected References
These references are in PubMed. This may not be the complete list of references from this article.
- Anukanth A., Ponnuswamy P. K. 2'5'-linked polynucleotides do form a double-stranded helical structure: a result from the energy minimization study of A2'p5'A. Biopolymers. 1986 Apr;25(4):729–752. doi: 10.1002/bip.360250414. [DOI] [PubMed] [Google Scholar]
- Arnott S., Selsing E. Structures for the polynucleotide complexes poly(dA) with poly (dT) and poly(dT) with poly(dA) with poly (dT). J Mol Biol. 1974 Sep 15;88(2):509–521. doi: 10.1016/0022-2836(74)90498-7. [DOI] [PubMed] [Google Scholar]
- Barone A. D., Tang J. Y., Caruthers M. H. In situ activation of bis-dialkylaminophosphines--a new method for synthesizing deoxyoligonucleotides on polymer supports. Nucleic Acids Res. 1984 May 25;12(10):4051–4061. doi: 10.1093/nar/12.10.4051. [DOI] [PMC free article] [PubMed] [Google Scholar]
- Breslauer K. J., Frank R., Blöcker H., Marky L. A. Predicting DNA duplex stability from the base sequence. Proc Natl Acad Sci U S A. 1986 Jun;83(11):3746–3750. doi: 10.1073/pnas.83.11.3746. [DOI] [PMC free article] [PubMed] [Google Scholar]
- Breslauer K. J., Freire E., Straume M. Calorimetry: a tool for DNA and ligand-DNA studies. Methods Enzymol. 1992;211:533–567. doi: 10.1016/0076-6879(92)11030-m. [DOI] [PubMed] [Google Scholar]
- Breslauer K. J., Sturtevant J. M., Tinoco I., Jr Calorimetric and spectroscopic investigation of the helix-to-coil transition of a ribo-oligonucleotide: rA7U7. J Mol Biol. 1975 Dec 25;99(4):549–565. doi: 10.1016/s0022-2836(75)80171-9. [DOI] [PubMed] [Google Scholar]
- Breslow R., Xu R. Recognition and catalysis in nucleic acid chemistry. Proc Natl Acad Sci U S A. 1993 Feb 15;90(4):1201–1207. doi: 10.1073/pnas.90.4.1201. [DOI] [PMC free article] [PubMed] [Google Scholar]
- Cantor C. R., Warshaw M. M., Shapiro H. Oligonucleotide interactions. 3. Circular dichroism studies of the conformation of deoxyoligonucleotides. Biopolymers. 1970;9(9):1059–1077. doi: 10.1002/bip.1970.360090909. [DOI] [PubMed] [Google Scholar]
- Dhingra M. M., Sarma R. H. Why do nucleic acids have 3'5' phosphodiester bonds? Nature. 1978 Apr 27;272(5656):798–801. doi: 10.1038/272798a0. [DOI] [PubMed] [Google Scholar]
- Gaffney B. L., Jones R. A. Thermodynamic comparison of the base pairs formed by the carcinogenic lesion O6-methylguanine with reference both to Watson-Crick pairs and to mismatched pairs. Biochemistry. 1989 Jul 11;28(14):5881–5889. doi: 10.1021/bi00440a026. [DOI] [PubMed] [Google Scholar]
- Gralla J., Crothers D. M. Free energy of imperfect nucleic acid helices. II. Small hairpin loops. J Mol Biol. 1973 Feb 5;73(4):497–511. doi: 10.1016/0022-2836(73)90096-x. [DOI] [PubMed] [Google Scholar]
- Kibler-Herzog L., Kell B., Zon G., Shinozuka K., Mizan S., Wilson W. D. Sequence dependent effects in methylphosphonate deoxyribonucleotide double and triple helical complexes. Nucleic Acids Res. 1990 Jun 25;18(12):3545–3555. doi: 10.1093/nar/18.12.3545. [DOI] [PMC free article] [PubMed] [Google Scholar]
- Kierzek R., He L., Turner D. H. Association of 2'-5' oligoribonucleotides. Nucleic Acids Res. 1992 Apr 11;20(7):1685–1690. doi: 10.1093/nar/20.7.1685. [DOI] [PMC free article] [PubMed] [Google Scholar]
- Kirshnan R., Seshadri T. P., Viswamitra M. A. Visualisation of a 2'-5' parallel stranded double helix at atomic resolution: crystal structure of cytidylyl-2',5'-adenosine. Nucleic Acids Res. 1991 Jan 25;19(2):379–384. doi: 10.1093/nar/19.2.379. [DOI] [PMC free article] [PubMed] [Google Scholar]
- Krakauer H., Sturtevant J. M. Heats of the helix-coil transitions of the poly A-poly U complexes. Biopolymers. 1968 Apr;6(4):491–512. doi: 10.1002/bip.1968.360060406. [DOI] [PubMed] [Google Scholar]
- Manning G. S. The molecular theory of polyelectrolyte solutions with applications to the electrostatic properties of polynucleotides. Q Rev Biophys. 1978 May;11(2):179–246. doi: 10.1017/s0033583500002031. [DOI] [PubMed] [Google Scholar]
- Manzini G., Xodo L. E., Gasparotto D., Quadrifoglio F., van der Marel G. A., van Boom J. H. Triple helix formation by oligopurine-oligopyrimidine DNA fragments. Electrophoretic and thermodynamic behavior. J Mol Biol. 1990 Jun 20;213(4):833–843. doi: 10.1016/S0022-2836(05)80267-0. [DOI] [PubMed] [Google Scholar]
- Marky L. A., Breslauer K. J. Calculating thermodynamic data for transitions of any molecularity from equilibrium melting curves. Biopolymers. 1987 Sep;26(9):1601–1620. doi: 10.1002/bip.360260911. [DOI] [PubMed] [Google Scholar]
- Park Y. W., Breslauer K. J. A spectroscopic and calorimetric study of the melting behaviors of a "bent" and a "normal" DNA duplex: [d(GA4T4C)]2 versus [d(GT4A4C)]2. Proc Natl Acad Sci U S A. 1991 Feb 15;88(4):1551–1555. doi: 10.1073/pnas.88.4.1551. [DOI] [PMC free article] [PubMed] [Google Scholar]
- Park Y. W., Breslauer K. J. Drug binding to higher ordered DNA structures: netropsin complexation with a nucleic acid triple helix. Proc Natl Acad Sci U S A. 1992 Jul 15;89(14):6653–6657. doi: 10.1073/pnas.89.14.6653. [DOI] [PMC free article] [PubMed] [Google Scholar]
- Parthasarathy R., Malik M., Fridey S. M. X-ray structure of a dinucleoside monophosphate A2'p5'C that contains a 2'-5' link found in (2'-5')oligo(A)s induced by interferons: single-stranded helical conformation of 2'-5'-linked oligonucleotides. Proc Natl Acad Sci U S A. 1982 Dec;79(23):7292–7296. doi: 10.1073/pnas.79.23.7292. [DOI] [PMC free article] [PubMed] [Google Scholar]
- Pilch D. S., Brousseau R., Shafer R. H. Thermodynamics of triple helix formation: spectrophotometric studies on the d(A)10.2d(T)10 and d(C+3T4C+3).d(G3A4G3).d(C3T4C3) triple helices. Nucleic Acids Res. 1990 Oct 11;18(19):5743–5750. doi: 10.1093/nar/18.19.5743. [DOI] [PMC free article] [PubMed] [Google Scholar]
- Pilch D. S., Levenson C., Shafer R. H. Structural analysis of the (dA)10.2(dT)10 triple helix. Proc Natl Acad Sci U S A. 1990 Mar;87(5):1942–1946. doi: 10.1073/pnas.87.5.1942. [DOI] [PMC free article] [PubMed] [Google Scholar]
- Plum G. E., Park Y. W., Singleton S. F., Dervan P. B., Breslauer K. J. Thermodynamic characterization of the stability and the melting behavior of a DNA triplex: a spectroscopic and calorimetric study. Proc Natl Acad Sci U S A. 1990 Dec;87(23):9436–9440. doi: 10.1073/pnas.87.23.9436. [DOI] [PMC free article] [PubMed] [Google Scholar]
- Record M. T., Jr, Anderson C. F., Lohman T. M. Thermodynamic analysis of ion effects on the binding and conformational equilibria of proteins and nucleic acids: the roles of ion association or release, screening, and ion effects on water activity. Q Rev Biophys. 1978 May;11(2):103–178. doi: 10.1017/s003358350000202x. [DOI] [PubMed] [Google Scholar]
- Record M. T., Jr, Woodbury C. P., Lohman T. M. Na+ effects on transition of DNA and polynucleotides of variable linear charge density. Biopolymers. 1976 May;15(5):893–915. doi: 10.1002/bip.1976.360150507. [DOI] [PubMed] [Google Scholar]
- Riley M., Maling B. Physical and chemical characterization of two- and three-stranded adenine-thymine and adenine-uracil homopolymer complexes. J Mol Biol. 1966 Sep;20(2):359–389. doi: 10.1016/0022-2836(66)90069-6. [DOI] [PubMed] [Google Scholar]
- Srinivasan A. R., Olson W. K. Conformational studies of (2'-5') polynucleotides: theoretical computations of energy, base morphology, helical structure, and duplex formation. Nucleic Acids Res. 1986 Jul 11;14(13):5461–5479. doi: 10.1093/nar/14.13.5461. [DOI] [PMC free article] [PubMed] [Google Scholar]
- Srinivasan A. R., Olson W. K. Nucleic acid model building: the multiple backbone solutions associated with a given base morphology. J Biomol Struct Dyn. 1987 Jun;4(6):895–938. doi: 10.1080/07391102.1987.10507690. [DOI] [PubMed] [Google Scholar]
- Sulston J., Lohrmann R., Orgel L. E., Schneider-Bernloehr H., Weimann B. J., Miles H. T. Non-enzymic oligonucleotide synthesis on a polycytidylate template. J Mol Biol. 1969 Mar 14;40(2):227–234. doi: 10.1016/0022-2836(69)90471-9. [DOI] [PubMed] [Google Scholar]
- Usher D. A., McHale A. H. Hydrolytic stability of helical RNA: a selective advantage for the natural 3',5'-bond. Proc Natl Acad Sci U S A. 1976 Apr;73(4):1149–1153. doi: 10.1073/pnas.73.4.1149. [DOI] [PMC free article] [PubMed] [Google Scholar]
- Völker J., Botes D. P., Lindsey G. G., Klump H. H. Energetics of a stable intramolecular DNA triple helix formation. J Mol Biol. 1993 Apr 20;230(4):1278–1290. doi: 10.1006/jmbi.1993.1241. [DOI] [PubMed] [Google Scholar]
- Warshaw M. M., Tinoco I., Jr Optical properties of sixteen dinucleoside phosphates. J Mol Biol. 1966 Sep;20(1):29–38. doi: 10.1016/0022-2836(66)90115-x. [DOI] [PubMed] [Google Scholar]