Abstract
We introduce a methodology, fluorescence lifetime imaging (FLIM), in which the contrast depends on the fluorescence lifetime at each point in a two-dimensional image and not on the local concentration and/or intensity of the fluorophore. We used FLIM to create lifetime images of NADH when free in solution and when bound to malate dehydrogenase. This represents a challenging case for lifetime imaging because the NADH decay times are just 0.4 and 1.0 ns in the free and bound states, respectively. In the present apparatus, lifetime images are created from a series of phase-sensitive images obtained with a gain-modulated image intensifier and recorded with a charge-coupled device (CCD) camera. The intensifier gain is modulated at the light-modulation frequency or a harmonic thereof. A series of stationary phase-sensitive images each obtained with various phase shifts of the gain-modulation signal, is used to determine the phase angle or modulation of the emission at each pixel, which is in essence the lifetime image. We also describe am imaging procedure that allows specific decay times to be suppressed, allowing in this case suppression of the emission from either free or bound NADH. Since the fluorescence lifetimes of probes are known to be sensitive to numerous chemical and physical factors such as pH, oxygen, temperature, cations, polarity, and binding to macromolecules, this method allows imaging of the chemical or property of interest in macroscopic and microscopic samples. The concept of FLIM appears to have numerous potential applications in the biosciences.
Full text
PDF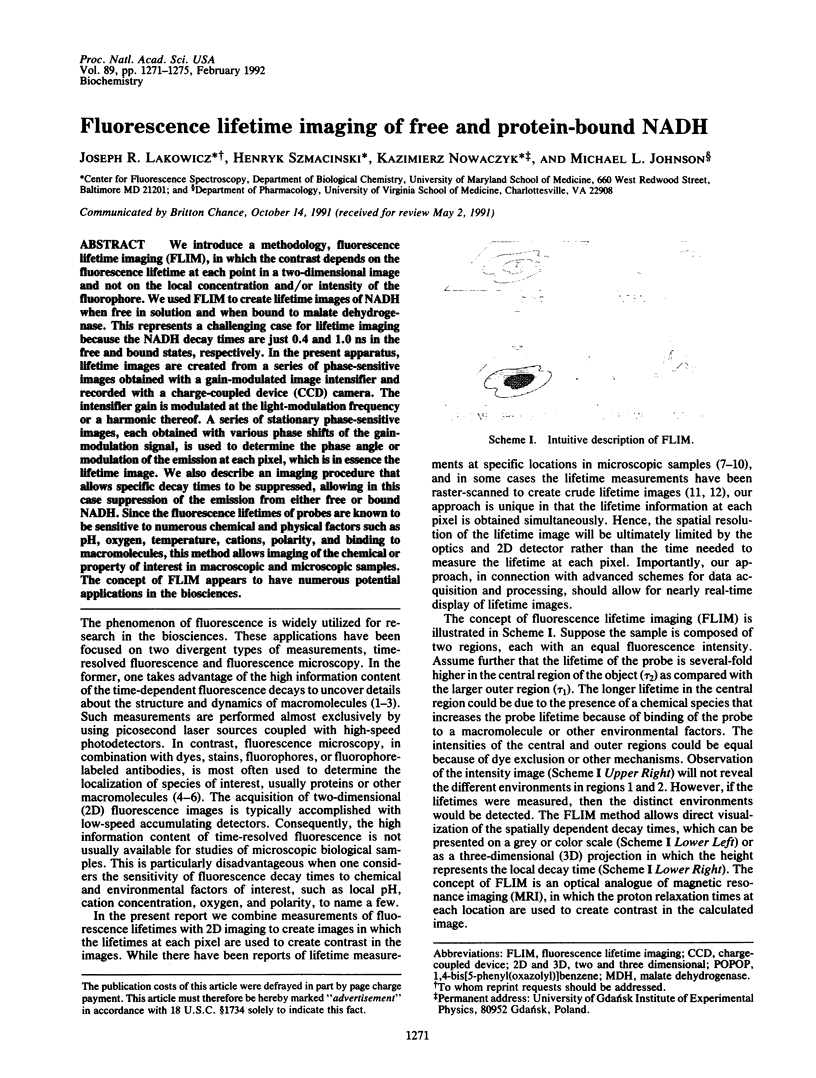
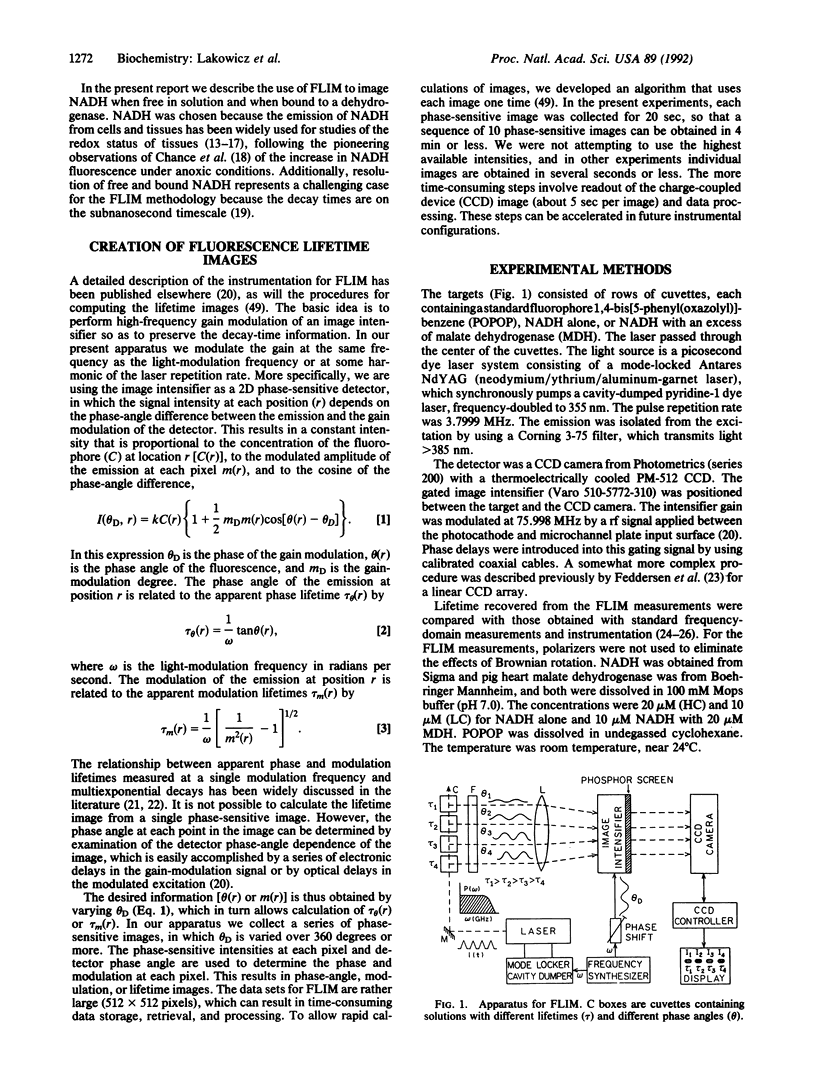
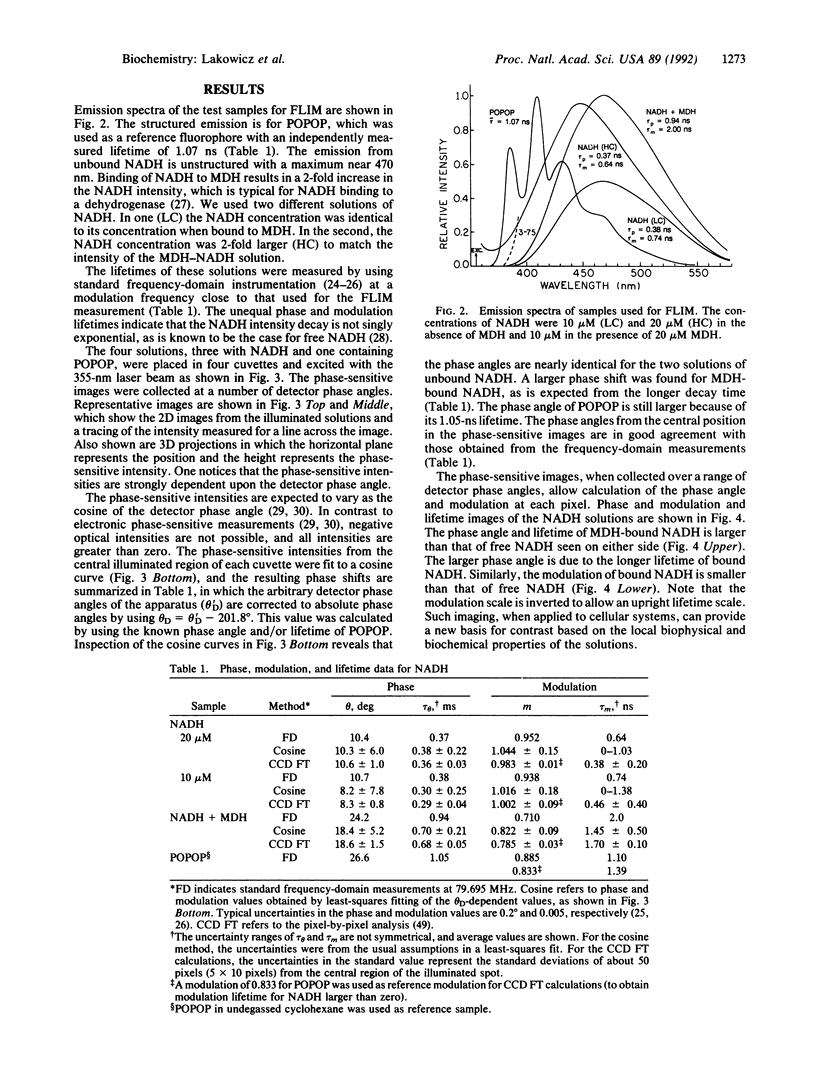
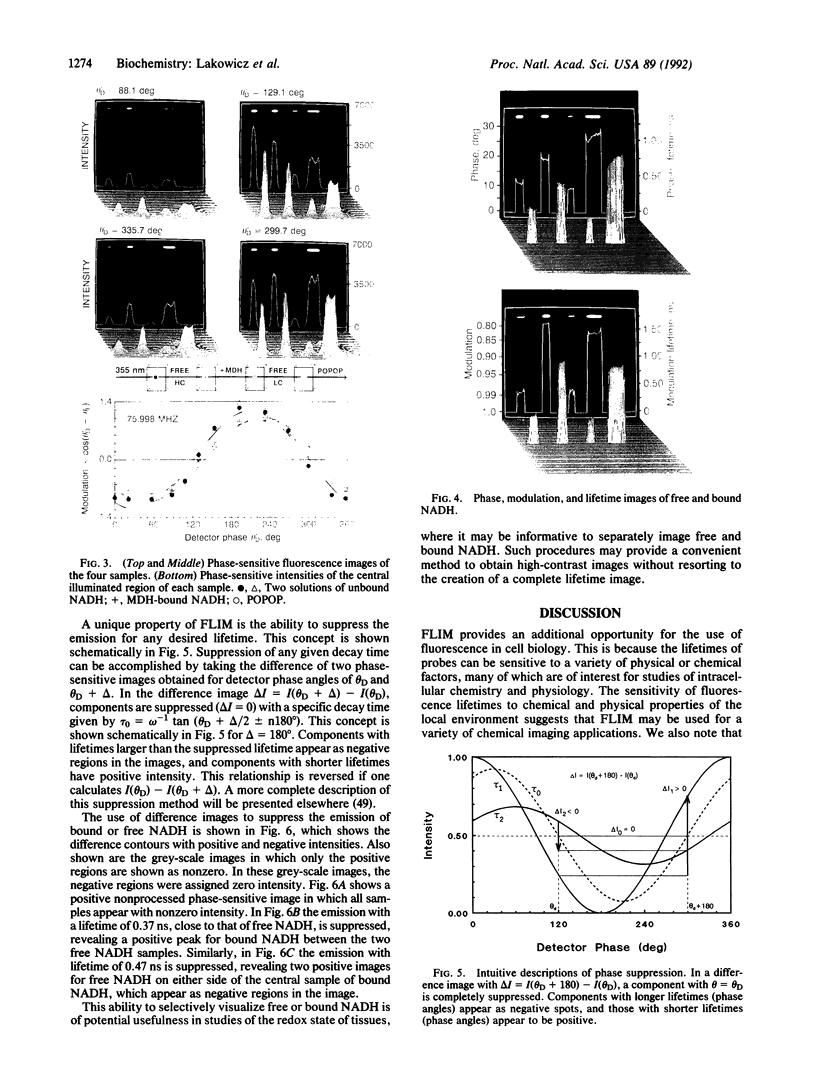
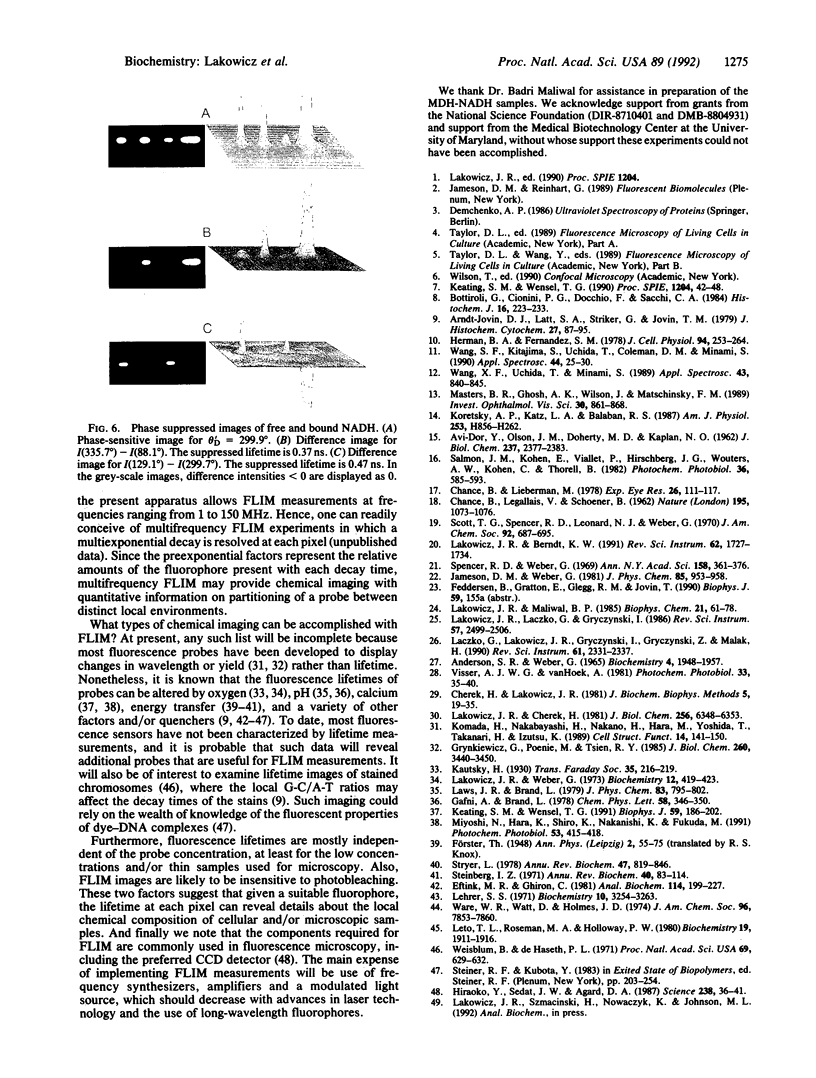
Images in this article
Selected References
These references are in PubMed. This may not be the complete list of references from this article.
- Arndt-Jovin D. J., Latt S. A., Striker G., Jovin T. M. Fluorescence decay analysis in solution and in a microscope of DNA and chromosomes stained with quinacrine. J Histochem Cytochem. 1979 Jan;27(1):87–95. doi: 10.1177/27.1.438507. [DOI] [PubMed] [Google Scholar]
- Bottiroli G., Cionini P. G., Docchio F., Sacchi C. A. In situ evaluation of the functional state of chromatin by means of Quinacrine Mustard staining and time-resolved fluorescence microscopy. Histochem J. 1984 Mar;16(3):223–233. doi: 10.1007/BF01003607. [DOI] [PubMed] [Google Scholar]
- CHANCE B., LEGALLAIS V., SCHOENER B. Metabolically linked changes in fluorescence emission spectra of cortex of rat brain, kidney and adrenal gland. Nature. 1962 Sep 15;195:1073–1075. doi: 10.1038/1951073a0. [DOI] [PubMed] [Google Scholar]
- Chance B., Lieberman M. Intrinsic fluorescence emission from the cornea at low temperatures: evidence of mitochondrial signals and their differing redox states in epithelial and endothelial sides. Exp Eye Res. 1978 Jan;26(1):111–117. doi: 10.1016/0014-4835(78)90159-8. [DOI] [PubMed] [Google Scholar]
- Eftink M. R., Ghiron C. A. Fluorescence quenching studies with proteins. Anal Biochem. 1981 Jul 1;114(2):199–227. doi: 10.1016/0003-2697(81)90474-7. [DOI] [PubMed] [Google Scholar]
- Grynkiewicz G., Poenie M., Tsien R. Y. A new generation of Ca2+ indicators with greatly improved fluorescence properties. J Biol Chem. 1985 Mar 25;260(6):3440–3450. [PubMed] [Google Scholar]
- Herman B. A., Fernandez S. M. Changes in membrane dynamics associated with myogenic cell fusion. J Cell Physiol. 1978 Mar;94(3):253–263. doi: 10.1002/jcp.1040940303. [DOI] [PubMed] [Google Scholar]
- Hiraoka Y., Sedat J. W., Agard D. A. The use of a charge-coupled device for quantitative optical microscopy of biological structures. Science. 1987 Oct 2;238(4823):36–41. doi: 10.1126/science.3116667. [DOI] [PubMed] [Google Scholar]
- Keating S. M., Wensel T. G. Nanosecond fluorescence microscopy. Emission kinetics of fura-2 in single cells. Biophys J. 1991 Jan;59(1):186–202. doi: 10.1016/S0006-3495(91)82210-X. [DOI] [PMC free article] [PubMed] [Google Scholar]
- Komada H., Nakabayashi H., Nakano H., Hara M., Yoshida T., Takanari H., Izutsu K. Measurement of the cytosolic free calcium ion concentration of individual lymphocytes by microfluorometry using quin 2 or fura-2. Cell Struct Funct. 1989 Apr;14(2):141–150. doi: 10.1247/csf.14.141. [DOI] [PubMed] [Google Scholar]
- Koretsky A. P., Katz L. A., Balaban R. S. Determination of pyridine nucleotide fluorescence from the perfused heart using an internal standard. Am J Physiol. 1987 Oct;253(4 Pt 2):H856–H862. doi: 10.1152/ajpheart.1987.253.4.H856. [DOI] [PubMed] [Google Scholar]
- Lakowicz J. R., Cherek H. Phase-sensitive fluorescence spectroscopy: a new method to resolve fluorescence lifetimes or emission spectra of components in a mixture of fluorophores. J Biochem Biophys Methods. 1981 Jul;5(1):19–35. doi: 10.1016/0165-022x(81)90030-0. [DOI] [PubMed] [Google Scholar]
- Lakowicz J. R., Cherek H. Resolution of heterogeneous fluorescence from proteins and aromatic amino acids by phase-sensitive detection of fluorescence. J Biol Chem. 1981 Jun 25;256(12):6348–6353. [PubMed] [Google Scholar]
- Lakowicz J. R., Maliwal B. P. Construction and performance of a variable-frequency phase-modulation fluorometer. Biophys Chem. 1985 Jan;21(1):61–78. doi: 10.1016/0301-4622(85)85007-9. [DOI] [PubMed] [Google Scholar]
- Lehrer S. S. Solute perturbation of protein fluorescence. The quenching of the tryptophyl fluorescence of model compounds and of lysozyme by iodide ion. Biochemistry. 1971 Aug 17;10(17):3254–3263. doi: 10.1021/bi00793a015. [DOI] [PubMed] [Google Scholar]
- Leto T. L., Roseman M. A., Holloway P. W. Mechanism of exchange of cytochrome b5 between phosphatidylcholine vesicles. Biochemistry. 1980 Apr 29;19(9):1911–1916. doi: 10.1021/bi00550a028. [DOI] [PubMed] [Google Scholar]
- Masters B. R., Ghosh A. K., Wilson J., Matschinsky F. M. Pyridine nucleotides and phosphorylation potential of rabbit corneal epithelium and endothelium. Invest Ophthalmol Vis Sci. 1989 May;30(5):861–868. [PubMed] [Google Scholar]
- Miyoshi N., Hara K., Kimura S., Nakanishi K., Fukuda M. A new method of determining intracellular free Ca2+ concentration using Quin2-fluorescence. Photochem Photobiol. 1991 Mar;53(3):415–418. doi: 10.1111/j.1751-1097.1991.tb03650.x. [DOI] [PubMed] [Google Scholar]
- Salmon J. M., Kohen E., Viallet P., Hirschberg J. G., Wouters A. W., Kohen C., Thorell B. Microspectrofluorometric approach to the study of free/bound NAD(P)H ratio as metabolic indicator in various cell types. Photochem Photobiol. 1982 Nov;36(5):585–593. doi: 10.1111/j.1751-1097.1982.tb04420.x. [DOI] [PubMed] [Google Scholar]
- Steinberg I. Z. Long-range nonradiative transfer of electronic excitation energy in proteins and polypeptides. Annu Rev Biochem. 1971;40:83–114. doi: 10.1146/annurev.bi.40.070171.000503. [DOI] [PubMed] [Google Scholar]
- Stryer L. Fluorescence energy transfer as a spectroscopic ruler. Annu Rev Biochem. 1978;47:819–846. doi: 10.1146/annurev.bi.47.070178.004131. [DOI] [PubMed] [Google Scholar]
- Weisblum B., De Haseth P. L. Quinacrine, a chromosome stain specific for deoxyadenylate-deoxythymidylaterich regions in DNA. Proc Natl Acad Sci U S A. 1972 Mar;69(3):629–632. doi: 10.1073/pnas.69.3.629. [DOI] [PMC free article] [PubMed] [Google Scholar]