Abstract
The gag genes of retroviruses encode nucleocapsid proteins that package genomic RNA and are essential for viral infectivity. These RNA binding proteins have a Cys-Xaa2-Cys-Xaa4-His-Xaa4-Cys zinc binding motif that is distinct from the typical zinc-finger motif Cys-Xaa2-Cys-Xaa12-14-His-Xaa2-His that is found in some transcriptional activators. Escherichia coli alanyl-tRNA synthetase contains a zinc-binding Cys-Xaa2-Cys-Xaa6-His-Xaa2-His motif that resembles that of retroviral nucleic acid binding proteins. We show here that, for alanyl-tRNA synthetase, the metal bound at the retroviral-like metal binding motif is important specifically for tRNA recognition and not for amino acid activation. Moreover, the enzyme-tRNA interaction is strongly dependent on the geometry of metal coordination to the protein. These and additional experiments collectively suggest a role for the retroviral-like metal binding motif in RNA recognition and, further, raise the possibility that the protein-bound metal itself participates in an RNA interaction.
Full text
PDF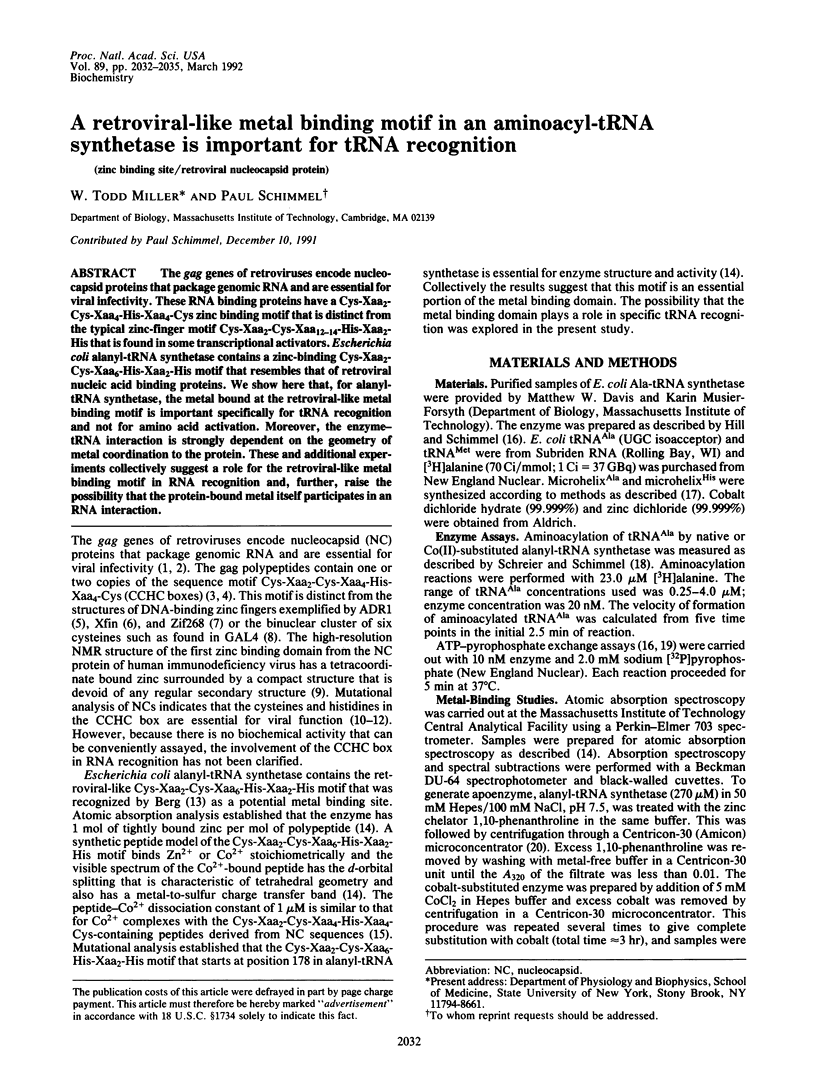
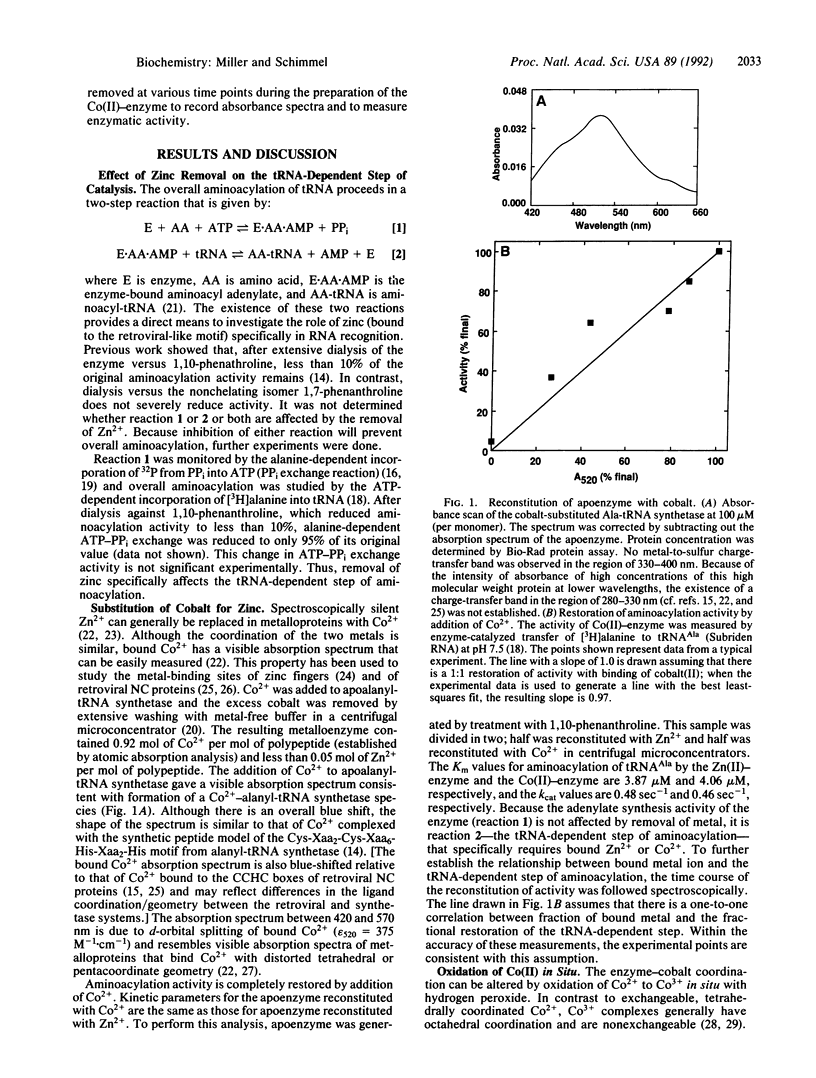
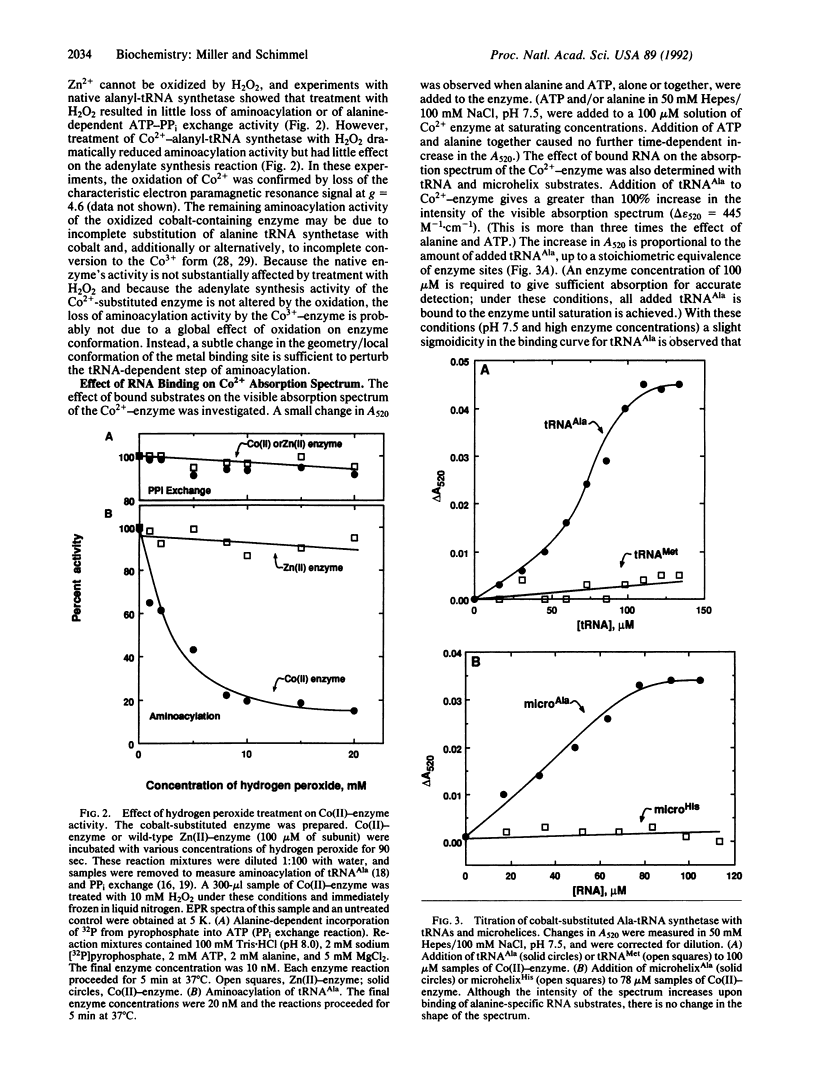
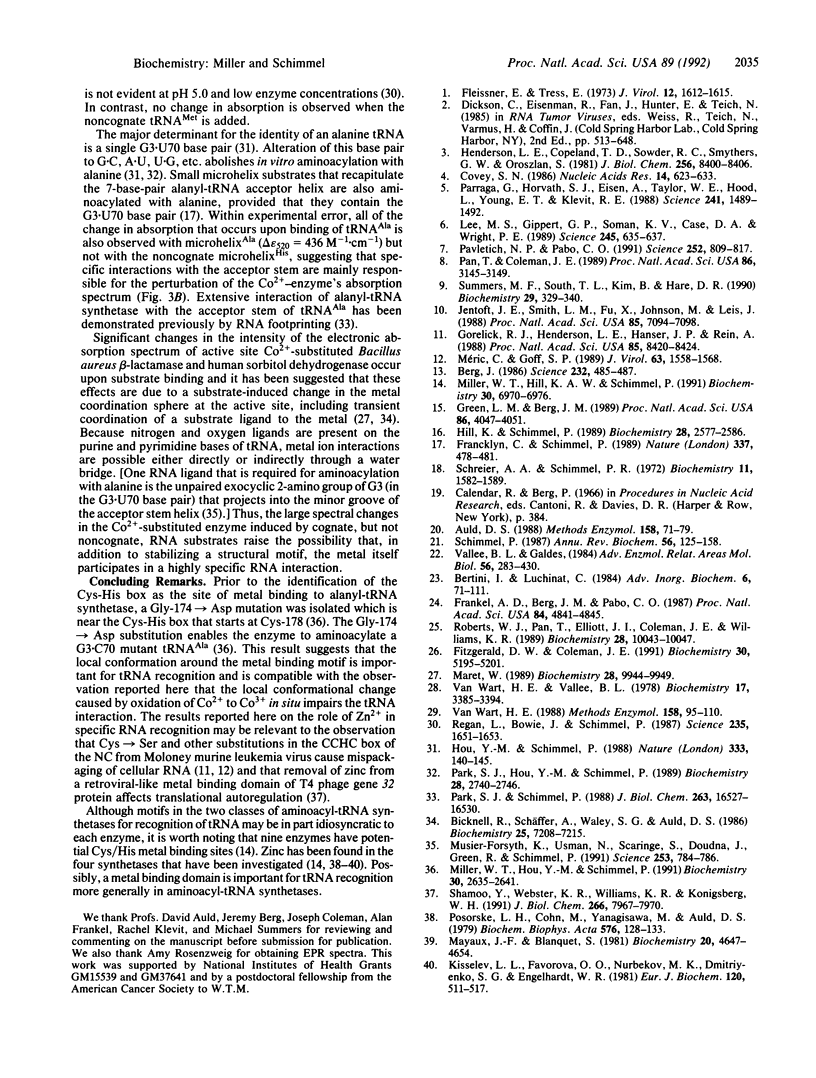
Selected References
These references are in PubMed. This may not be the complete list of references from this article.
- Auld D. S. Methods for metal substitution. Methods Enzymol. 1988;158:71–79. doi: 10.1016/0076-6879(88)58048-5. [DOI] [PubMed] [Google Scholar]
- Berg J. M. Potential metal-binding domains in nucleic acid binding proteins. Science. 1986 Apr 25;232(4749):485–487. doi: 10.1126/science.2421409. [DOI] [PubMed] [Google Scholar]
- Bertini I., Luchinat C. High spin cobalt(II) as a probe for the investigation of metalloproteins. Adv Inorg Biochem. 1984;6:71–111. [PubMed] [Google Scholar]
- Bicknell R., Schäffer A., Waley S. G., Auld D. S. Changes in the coordination geometry of the active-site metal during catalysis of benzylpenicillin hydrolysis by Bacillus cereus beta-lactamase II. Biochemistry. 1986 Nov 4;25(22):7208–7215. doi: 10.1021/bi00370a066. [DOI] [PubMed] [Google Scholar]
- Covey S. N. Amino acid sequence homology in gag region of reverse transcribing elements and the coat protein gene of cauliflower mosaic virus. Nucleic Acids Res. 1986 Jan 24;14(2):623–633. doi: 10.1093/nar/14.2.623. [DOI] [PMC free article] [PubMed] [Google Scholar]
- Fitzgerald D. W., Coleman J. E. Physicochemical properties of cloned nucleocapsid protein from HIV. Interactions with metal ions. Biochemistry. 1991 May 28;30(21):5195–5201. doi: 10.1021/bi00235a012. [DOI] [PubMed] [Google Scholar]
- Fleissner E., Tress E. Isolation of a ribonucleoprotein structure from oncornaviruses. J Virol. 1973 Dec;12(6):1612–1615. doi: 10.1128/jvi.12.6.1612-1615.1973. [DOI] [PMC free article] [PubMed] [Google Scholar]
- Francklyn C., Schimmel P. Aminoacylation of RNA minihelices with alanine. Nature. 1989 Feb 2;337(6206):478–481. doi: 10.1038/337478a0. [DOI] [PubMed] [Google Scholar]
- Frankel A. D., Berg J. M., Pabo C. O. Metal-dependent folding of a single zinc finger from transcription factor IIIA. Proc Natl Acad Sci U S A. 1987 Jul;84(14):4841–4845. doi: 10.1073/pnas.84.14.4841. [DOI] [PMC free article] [PubMed] [Google Scholar]
- Gorelick R. J., Henderson L. E., Hanser J. P., Rein A. Point mutants of Moloney murine leukemia virus that fail to package viral RNA: evidence for specific RNA recognition by a "zinc finger-like" protein sequence. Proc Natl Acad Sci U S A. 1988 Nov;85(22):8420–8424. doi: 10.1073/pnas.85.22.8420. [DOI] [PMC free article] [PubMed] [Google Scholar]
- Green L. M., Berg J. M. A retroviral Cys-Xaa2-Cys-Xaa4-His-Xaa4-Cys peptide binds metal ions: spectroscopic studies and a proposed three-dimensional structure. Proc Natl Acad Sci U S A. 1989 Jun;86(11):4047–4051. doi: 10.1073/pnas.86.11.4047. [DOI] [PMC free article] [PubMed] [Google Scholar]
- Henderson L. E., Copeland T. D., Sowder R. C., Smythers G. W., Oroszlan S. Primary structure of the low molecular weight nucleic acid-binding proteins of murine leukemia viruses. J Biol Chem. 1981 Aug 25;256(16):8400–8406. [PubMed] [Google Scholar]
- Hill K., Schimmel P. Evidence that the 3' end of a tRNA binds to a site in the adenylate synthesis domain of an aminoacyl-tRNA synthetase. Biochemistry. 1989 Mar 21;28(6):2577–2586. doi: 10.1021/bi00432a035. [DOI] [PubMed] [Google Scholar]
- Hou Y. M., Schimmel P. A simple structural feature is a major determinant of the identity of a transfer RNA. Nature. 1988 May 12;333(6169):140–145. doi: 10.1038/333140a0. [DOI] [PubMed] [Google Scholar]
- Jentoft J. E., Smith L. M., Fu X. D., Johnson M., Leis J. Conserved cysteine and histidine residues of the avian myeloblastosis virus nucleocapsid protein are essential for viral replication but are not "zinc-binding fingers". Proc Natl Acad Sci U S A. 1988 Oct;85(19):7094–7098. doi: 10.1073/pnas.85.19.7094. [DOI] [PMC free article] [PubMed] [Google Scholar]
- Kisselev L. L., Favorova O. O., Nurbekov M. K., Dmitriyenko S. G., Engelhardt W. A. Bovine tryptophanyl-tRNA synthetase. A zinc metalloenzyme. Eur J Biochem. 1981 Dec;120(3):511–517. doi: 10.1111/j.1432-1033.1981.tb05729.x. [DOI] [PubMed] [Google Scholar]
- Lee M. S., Gippert G. P., Soman K. V., Case D. A., Wright P. E. Three-dimensional solution structure of a single zinc finger DNA-binding domain. Science. 1989 Aug 11;245(4918):635–637. doi: 10.1126/science.2503871. [DOI] [PubMed] [Google Scholar]
- Maret W. Cobalt(II)-substituted class III alcohol and sorbitol dehydrogenases from human liver. Biochemistry. 1989 Dec 26;28(26):9944–9949. doi: 10.1021/bi00452a011. [DOI] [PubMed] [Google Scholar]
- Mayaux J. F., Blanquet S. Binding of zinc to Escherichia coli phenylalanyl transfer ribonucleic acid synthetase. Comparison with other aminoacyl transfer ribonucleic acid synthetases. Biochemistry. 1981 Aug 4;20(16):4647–4654. doi: 10.1021/bi00519a020. [DOI] [PubMed] [Google Scholar]
- Miller W. T., Hill K. A., Schimmel P. Evidence for a "cysteine-histidine box" metal-binding site in an Escherichia coli aminoacyl-tRNA synthetase. Biochemistry. 1991 Jul 16;30(28):6970–6976. doi: 10.1021/bi00242a023. [DOI] [PubMed] [Google Scholar]
- Miller W. T., Hou Y. M., Schimmel P. Mutant aminoacyl-tRNA synthetase that compensates for a mutation in the major identity determinant of its tRNA. Biochemistry. 1991 Mar 12;30(10):2635–2641. doi: 10.1021/bi00224a011. [DOI] [PubMed] [Google Scholar]
- Musier-Forsyth K., Usman N., Scaringe S., Doudna J., Green R., Schimmel P. Specificity for aminoacylation of an RNA helix: an unpaired, exocyclic amino group in the minor groove. Science. 1991 Aug 16;253(5021):784–786. doi: 10.1126/science.1876835. [DOI] [PubMed] [Google Scholar]
- Méric C., Goff S. P. Characterization of Moloney murine leukemia virus mutants with single-amino-acid substitutions in the Cys-His box of the nucleocapsid protein. J Virol. 1989 Apr;63(4):1558–1568. doi: 10.1128/jvi.63.4.1558-1568.1989. [DOI] [PMC free article] [PubMed] [Google Scholar]
- Pan T., Coleman J. E. Structure and function of the Zn(II) binding site within the DNA-binding domain of the GAL4 transcription factor. Proc Natl Acad Sci U S A. 1989 May;86(9):3145–3149. doi: 10.1073/pnas.86.9.3145. [DOI] [PMC free article] [PubMed] [Google Scholar]
- Park S. J., Hou Y. M., Schimmel P. A single base pair affects binding and catalytic parameters in the molecular recognition of a transfer RNA. Biochemistry. 1989 Mar 21;28(6):2740–2746. doi: 10.1021/bi00432a056. [DOI] [PubMed] [Google Scholar]
- Park S. J., Schimmel P. Evidence for interaction of an aminoacyl transfer RNA synthetase with a region important for the identity of its cognate transfer RNA. J Biol Chem. 1988 Nov 15;263(32):16527–16530. [PubMed] [Google Scholar]
- Pavletich N. P., Pabo C. O. Zinc finger-DNA recognition: crystal structure of a Zif268-DNA complex at 2.1 A. Science. 1991 May 10;252(5007):809–817. doi: 10.1126/science.2028256. [DOI] [PubMed] [Google Scholar]
- Posorske L. H., Cohn M., Yanagisawa N., Auld D. S. Methionyl-tRNA synthetase of Escherichia coli. A zinc metalloprotein. Biochim Biophys Acta. 1979 Jan 25;576(1):128–133. doi: 10.1016/0005-2795(79)90491-4. [DOI] [PubMed] [Google Scholar]
- Párraga G., Horvath S. J., Eisen A., Taylor W. E., Hood L., Young E. T., Klevit R. E. Zinc-dependent structure of a single-finger domain of yeast ADR1. Science. 1988 Sep 16;241(4872):1489–1492. doi: 10.1126/science.3047872. [DOI] [PubMed] [Google Scholar]
- Regan L., Bowie J., Schimmel P. Polypeptide sequences essential for RNA recognition by an enzyme. Science. 1987 Mar 27;235(4796):1651–1653. doi: 10.1126/science.2435005. [DOI] [PubMed] [Google Scholar]
- Roberts W. J., Pan T., Elliott J. I., Coleman J. E., Williams K. R. p10 single-stranded nucleic acid binding protein from murine leukemia virus binds metal ions via the peptide sequence Cys26-X2-Cys29-X4-His34-X4-Cys39. Biochemistry. 1989 Dec 26;28(26):10043–10047. doi: 10.1021/bi00452a024. [DOI] [PubMed] [Google Scholar]
- Schimmel P. Aminoacyl tRNA synthetases: general scheme of structure-function relationships in the polypeptides and recognition of transfer RNAs. Annu Rev Biochem. 1987;56:125–158. doi: 10.1146/annurev.bi.56.070187.001013. [DOI] [PubMed] [Google Scholar]
- Schreier A. A., Schimmel P. R. Transfer ribonucleic acid synthetase catalyzed deacylation of aminoacyl transfer ribonucleic acid in the absence of adenosine monophosphate and pyrophosphate. Biochemistry. 1972 Apr 25;11(9):1582–1589. doi: 10.1021/bi00759a006. [DOI] [PubMed] [Google Scholar]
- Shamoo Y., Webster K. R., Williams K. R., Konigsberg W. H. A retrovirus-like zinc domain is essential for translational repression of bacteriophage T4 gene 32. J Biol Chem. 1991 May 5;266(13):7967–7970. [PubMed] [Google Scholar]
- Summers M. F., South T. L., Kim B., Hare D. R. High-resolution structure of an HIV zinc fingerlike domain via a new NMR-based distance geometry approach. Biochemistry. 1990 Jan 16;29(2):329–340. doi: 10.1021/bi00454a005. [DOI] [PubMed] [Google Scholar]
- Vallee B. L., Galdes A. The metallobiochemistry of zinc enzymes. Adv Enzymol Relat Areas Mol Biol. 1984;56:283–430. doi: 10.1002/9780470123027.ch5. [DOI] [PubMed] [Google Scholar]
- Van Wart H. E. Introduction of exchange-inert metal ions into enzymes. Methods Enzymol. 1988;158:95–110. doi: 10.1016/0076-6879(88)58050-3. [DOI] [PubMed] [Google Scholar]
- van Wart H. E., Vallee B. L. Enzymatically inactive, exchange-inert Co(III)-carboxypeptidase A: role of inner sphere coordination in peptide and ester catalysis. Biochemistry. 1978 Aug 8;17(16):3385–3394. doi: 10.1021/bi00609a032. [DOI] [PubMed] [Google Scholar]