Abstract
Cell cycle checkpoints appear to contribute to an increase in cell survival and a decrease in abnormal heritable genetic changes following exposure to DNA damaging agents. Though several radiation-sensitive yeast mutants have been identified, little is known about the genes that control these responses in mammalian cells. Recent studies from our laboratory have demonstrated a close correlation between expression of wild-type p53 genes in human hematopoietic cells and their ability to arrest in G1 phase after certain types of DNA damage. In the present study, this correlation was first generalized to nonhematopoietic mammalian cells as well. A cause and effect relationship between expression of wild-type p53 and the G1 arrest that occurs after gamma irradiation was then established by demonstrating (i) acquisition of the G1 arrest after gamma irradiation following transfection of wild-type p53 genes into cells lacking endogenous p53 genes and (ii) loss of the G1 arrest after irradiation following transfection of mutant p53 genes into cells with wild-type endogenous p53 genes. A defined role for p53 (the most commonly mutated gene in human cancers) in a physiologic pathway has, to our knowledge, not been reported previously. Furthermore, these experiments illustrate one way in which a mutant p53 gene product can function in a "dominant negative" manner. Participation of p53 in this pathway suggests a mechanism for the contribution of abnormalities in p53 to tumorigenesis and genetic instability and provides a useful model for studies of the molecular mechanisms of p53 involvement in controlling the cell cycle.
Full text
PDF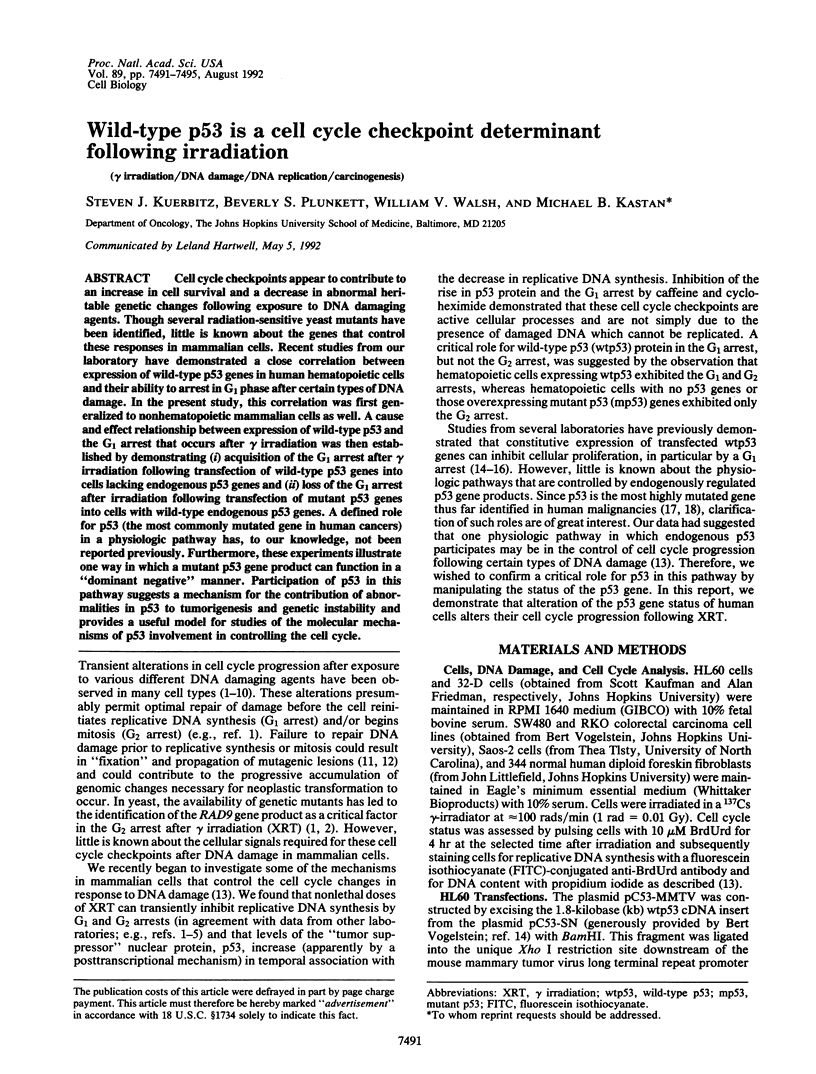
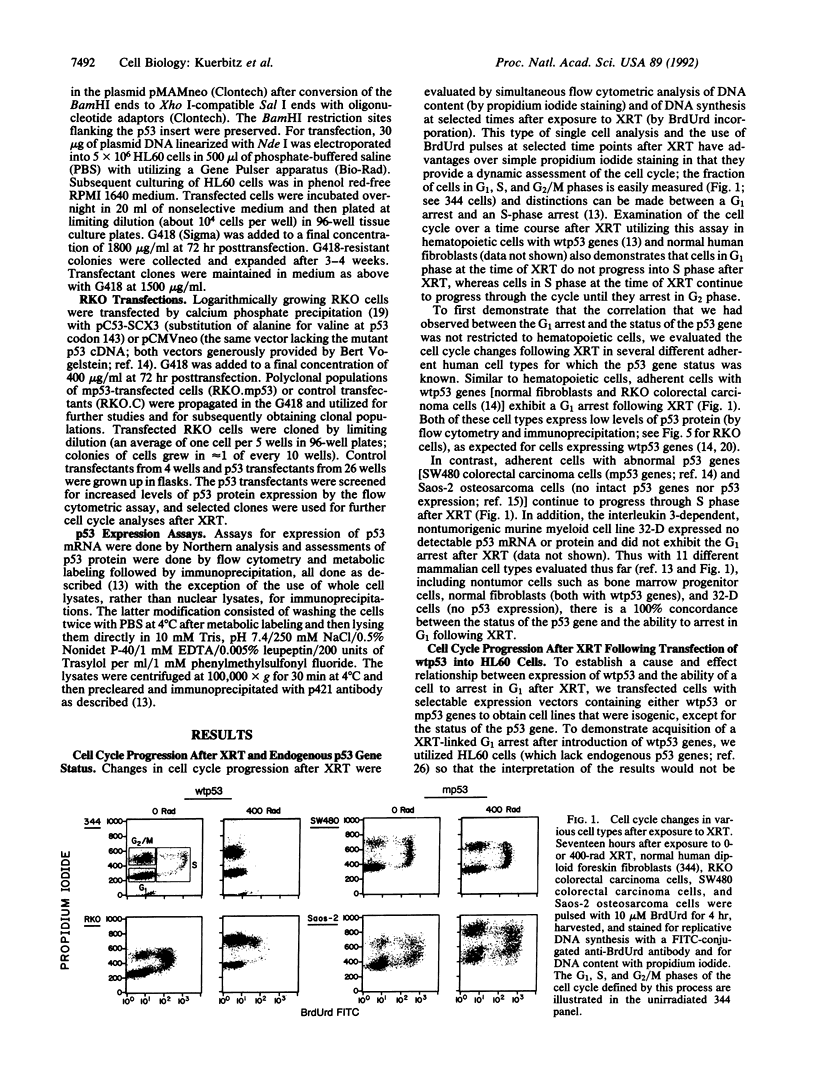
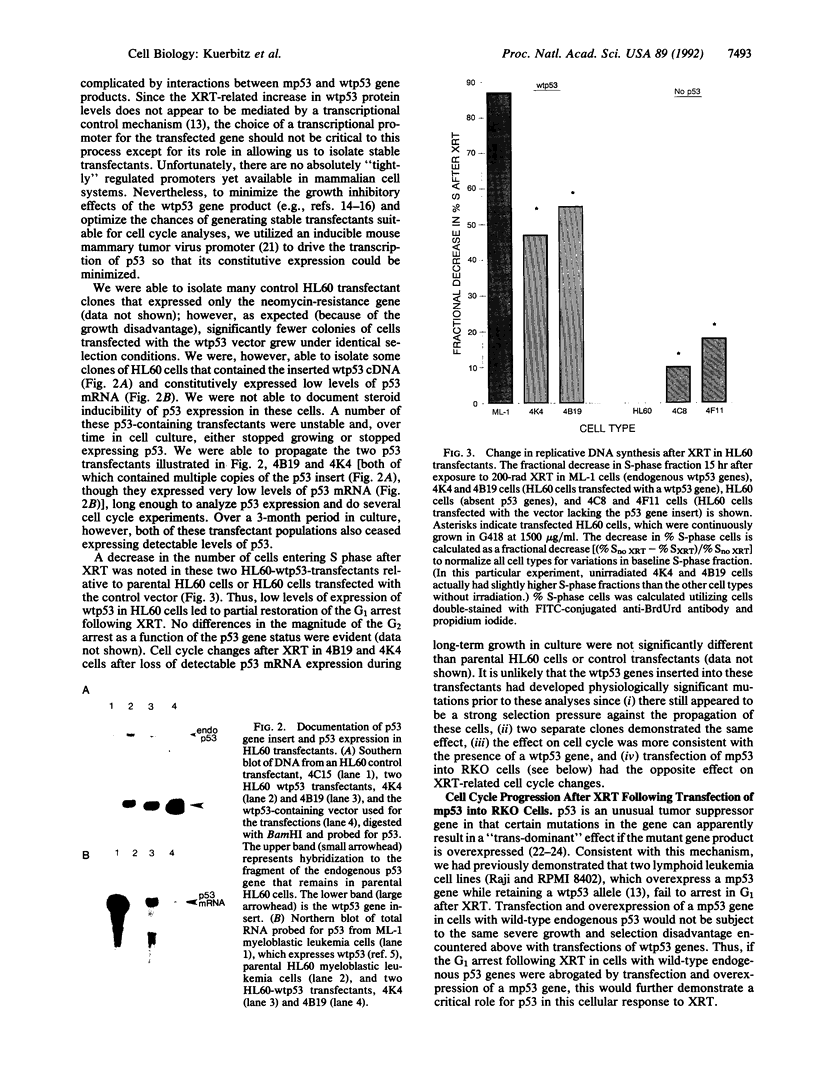
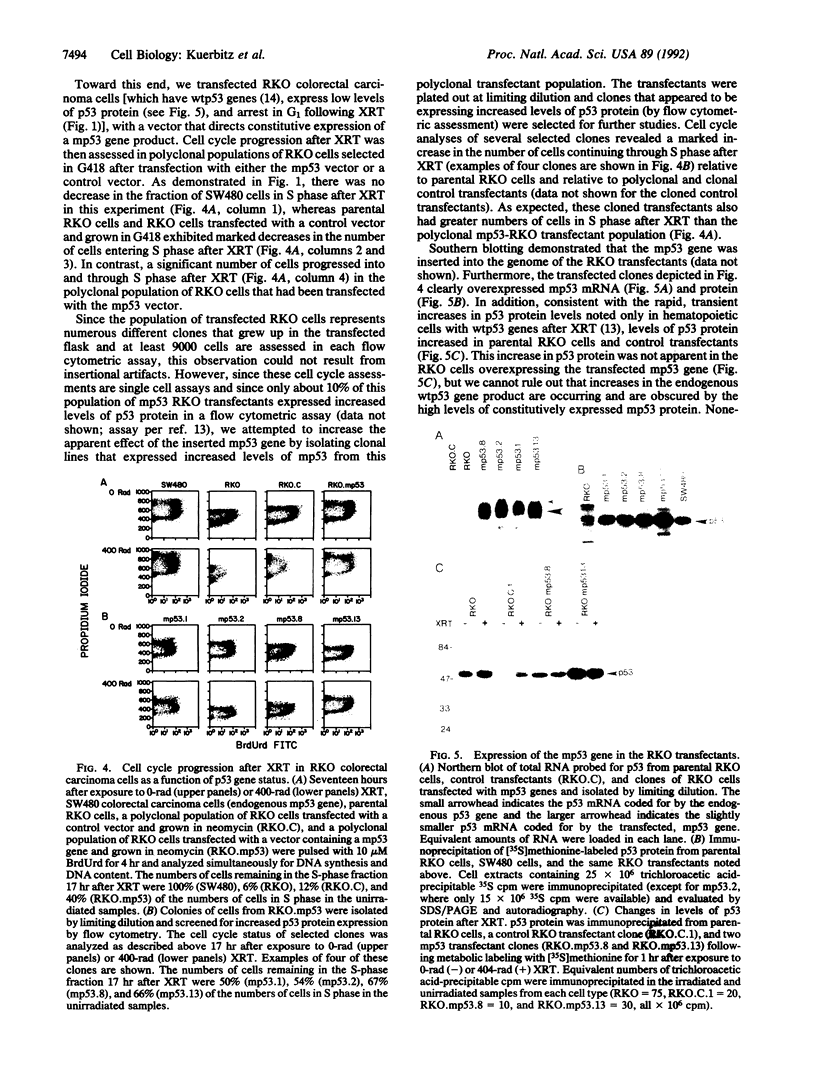
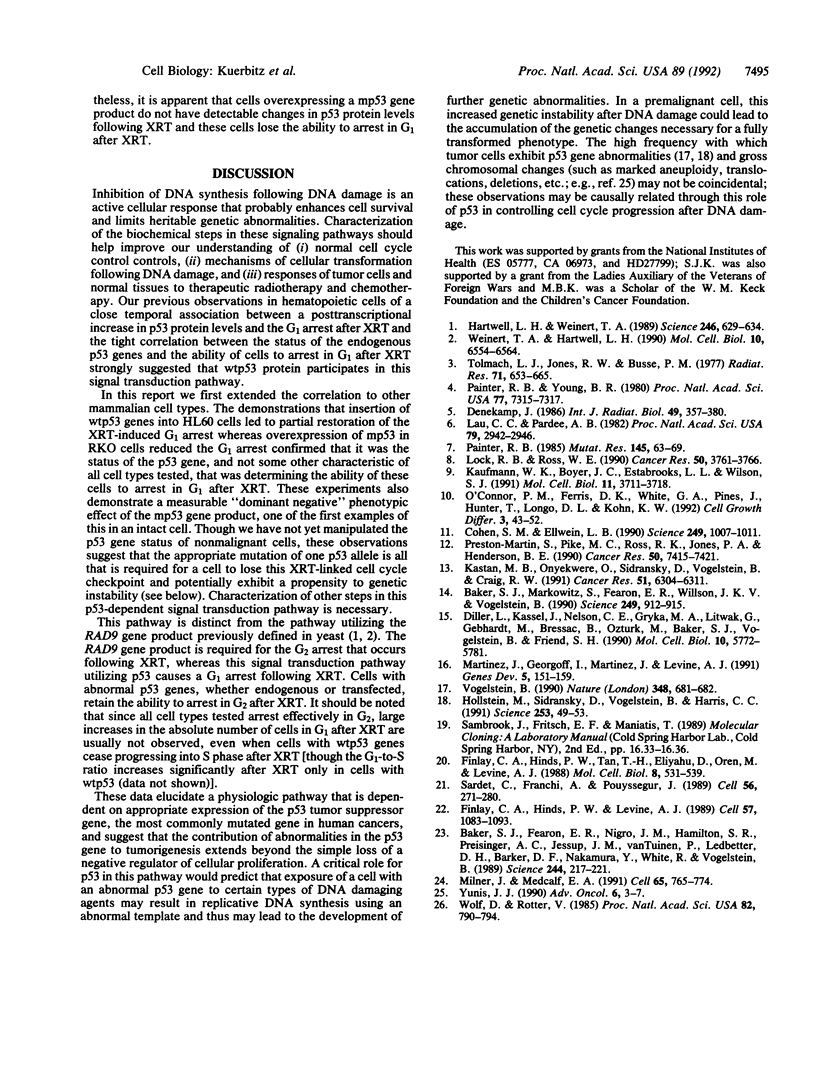
Images in this article
Selected References
These references are in PubMed. This may not be the complete list of references from this article.
- Baker S. J., Fearon E. R., Nigro J. M., Hamilton S. R., Preisinger A. C., Jessup J. M., vanTuinen P., Ledbetter D. H., Barker D. F., Nakamura Y. Chromosome 17 deletions and p53 gene mutations in colorectal carcinomas. Science. 1989 Apr 14;244(4901):217–221. doi: 10.1126/science.2649981. [DOI] [PubMed] [Google Scholar]
- Baker S. J., Markowitz S., Fearon E. R., Willson J. K., Vogelstein B. Suppression of human colorectal carcinoma cell growth by wild-type p53. Science. 1990 Aug 24;249(4971):912–915. doi: 10.1126/science.2144057. [DOI] [PubMed] [Google Scholar]
- Cohen S. M., Ellwein L. B. Cell proliferation in carcinogenesis. Science. 1990 Aug 31;249(4972):1007–1011. doi: 10.1126/science.2204108. [DOI] [PubMed] [Google Scholar]
- Denekamp J. Cell kinetics and radiation biology. Int J Radiat Biol Relat Stud Phys Chem Med. 1986 Feb;49(2):357–380. doi: 10.1080/09553008514552591. [DOI] [PubMed] [Google Scholar]
- Diller L., Kassel J., Nelson C. E., Gryka M. A., Litwak G., Gebhardt M., Bressac B., Ozturk M., Baker S. J., Vogelstein B. p53 functions as a cell cycle control protein in osteosarcomas. Mol Cell Biol. 1990 Nov;10(11):5772–5781. doi: 10.1128/mcb.10.11.5772. [DOI] [PMC free article] [PubMed] [Google Scholar]
- Finlay C. A., Hinds P. W., Levine A. J. The p53 proto-oncogene can act as a suppressor of transformation. Cell. 1989 Jun 30;57(7):1083–1093. doi: 10.1016/0092-8674(89)90045-7. [DOI] [PubMed] [Google Scholar]
- Finlay C. A., Hinds P. W., Tan T. H., Eliyahu D., Oren M., Levine A. J. Activating mutations for transformation by p53 produce a gene product that forms an hsc70-p53 complex with an altered half-life. Mol Cell Biol. 1988 Feb;8(2):531–539. doi: 10.1128/mcb.8.2.531. [DOI] [PMC free article] [PubMed] [Google Scholar]
- Hartwell L. H., Weinert T. A. Checkpoints: controls that ensure the order of cell cycle events. Science. 1989 Nov 3;246(4930):629–634. doi: 10.1126/science.2683079. [DOI] [PubMed] [Google Scholar]
- Hollstein M., Sidransky D., Vogelstein B., Harris C. C. p53 mutations in human cancers. Science. 1991 Jul 5;253(5015):49–53. doi: 10.1126/science.1905840. [DOI] [PubMed] [Google Scholar]
- Kastan M. B., Onyekwere O., Sidransky D., Vogelstein B., Craig R. W. Participation of p53 protein in the cellular response to DNA damage. Cancer Res. 1991 Dec 1;51(23 Pt 1):6304–6311. [PubMed] [Google Scholar]
- Kaufmann W. K., Boyer J. C., Estabrooks L. L., Wilson S. J. Inhibition of replicon initiation in human cells following stabilization of topoisomerase-DNA cleavable complexes. Mol Cell Biol. 1991 Jul;11(7):3711–3718. doi: 10.1128/mcb.11.7.3711. [DOI] [PMC free article] [PubMed] [Google Scholar]
- Lau C. C., Pardee A. B. Mechanism by which caffeine potentiates lethality of nitrogen mustard. Proc Natl Acad Sci U S A. 1982 May;79(9):2942–2946. doi: 10.1073/pnas.79.9.2942. [DOI] [PMC free article] [PubMed] [Google Scholar]
- Lock R. B., Ross W. E. Inhibition of p34cdc2 kinase activity by etoposide or irradiation as a mechanism of G2 arrest in Chinese hamster ovary cells. Cancer Res. 1990 Jun 15;50(12):3761–3766. [PubMed] [Google Scholar]
- Martinez J., Georgoff I., Martinez J., Levine A. J. Cellular localization and cell cycle regulation by a temperature-sensitive p53 protein. Genes Dev. 1991 Feb;5(2):151–159. doi: 10.1101/gad.5.2.151. [DOI] [PubMed] [Google Scholar]
- Milner J., Medcalf E. A. Cotranslation of activated mutant p53 with wild type drives the wild-type p53 protein into the mutant conformation. Cell. 1991 May 31;65(5):765–774. doi: 10.1016/0092-8674(91)90384-b. [DOI] [PubMed] [Google Scholar]
- O'Connor P. M., Ferris D. K., White G. A., Pines J., Hunter T., Longo D. L., Kohn K. W. Relationships between cdc2 kinase, DNA cross-linking, and cell cycle perturbations induced by nitrogen mustard. Cell Growth Differ. 1992 Jan;3(1):43–52. [PubMed] [Google Scholar]
- Painter R. B. Inhibition and recovery of DNA synthesis in human cells after exposure to ultraviolet light. Mutat Res. 1985 Jan-Mar;145(1-2):63–69. doi: 10.1016/0167-8817(85)90041-0. [DOI] [PubMed] [Google Scholar]
- Painter R. B., Young B. R. Radiosensitivity in ataxia-telangiectasia: a new explanation. Proc Natl Acad Sci U S A. 1980 Dec;77(12):7315–7317. doi: 10.1073/pnas.77.12.7315. [DOI] [PMC free article] [PubMed] [Google Scholar]
- Preston-Martin S., Pike M. C., Ross R. K., Jones P. A., Henderson B. E. Increased cell division as a cause of human cancer. Cancer Res. 1990 Dec 1;50(23):7415–7421. [PubMed] [Google Scholar]
- Sardet C., Franchi A., Pouysségur J. Molecular cloning, primary structure, and expression of the human growth factor-activatable Na+/H+ antiporter. Cell. 1989 Jan 27;56(2):271–280. doi: 10.1016/0092-8674(89)90901-x. [DOI] [PubMed] [Google Scholar]
- Tolmach L. J., Jones R. W., Busse P. M. The action of caffeine on X-irradiated HeLa cells. I. Delayed inhibition of DNA synthesis. Radiat Res. 1977 Sep;71(3):653–665. [PubMed] [Google Scholar]
- Vogelstein B. Cancer. A deadly inheritance. Nature. 1990 Dec 20;348(6303):681–682. doi: 10.1038/348681a0. [DOI] [PubMed] [Google Scholar]
- Weinert T. A., Hartwell L. H. Characterization of RAD9 of Saccharomyces cerevisiae and evidence that its function acts posttranslationally in cell cycle arrest after DNA damage. Mol Cell Biol. 1990 Dec;10(12):6554–6564. doi: 10.1128/mcb.10.12.6554. [DOI] [PMC free article] [PubMed] [Google Scholar]
- Wolf D., Rotter V. Major deletions in the gene encoding the p53 tumor antigen cause lack of p53 expression in HL-60 cells. Proc Natl Acad Sci U S A. 1985 Feb;82(3):790–794. doi: 10.1073/pnas.82.3.790. [DOI] [PMC free article] [PubMed] [Google Scholar]