Abstract
The DNA base-pair sequences that serve as gene-regulatory sites have been selected during evolution to provide an appropriate functional binding for a particular protein. In most cases, the function depends on the binding probability, which can be influenced both by the binding strength and by the abundance of the protein in the cell. As a consequence, the same function can be achieved with strong binding sites and a small amount of protein as with weak binding sites and a large amount of protein. However, increasing the protein burden will decrease the growth rate of the cells, even when all functions remain the same. Thus, for maximal growth, the protein levels should be as low as possible and the binding correspondingly strong. On the other hand, sequences with a weaker binding can be formed in many more ways and are, therefore, more probable, and random mutations are more likely to produce them. Thus, the selection pressure against an increased protein burden can be balanced against the random mutational drift in the recognition sequences, thereby tying together the statistics of base-pair choice, the binding strength, and the protein burden. In terms of this model, the selection pressure can be estimated from the properties of a gene-regulatory protein and its recognition sites. A key feature is the mutational randomization pressure that appears as a fundamental force shaping the optimal solutions that provide maximal growth. The model is tested on a number of gene-regulatory systems in Escherichia coli. The same principles should hold for all proteins for which overall activity in the cell is proportional to abundance; then the selective pressure to increase the efficiency of an individual protein cannot be larger than the selective pressure to decrease the total protein burden.
Full text
PDF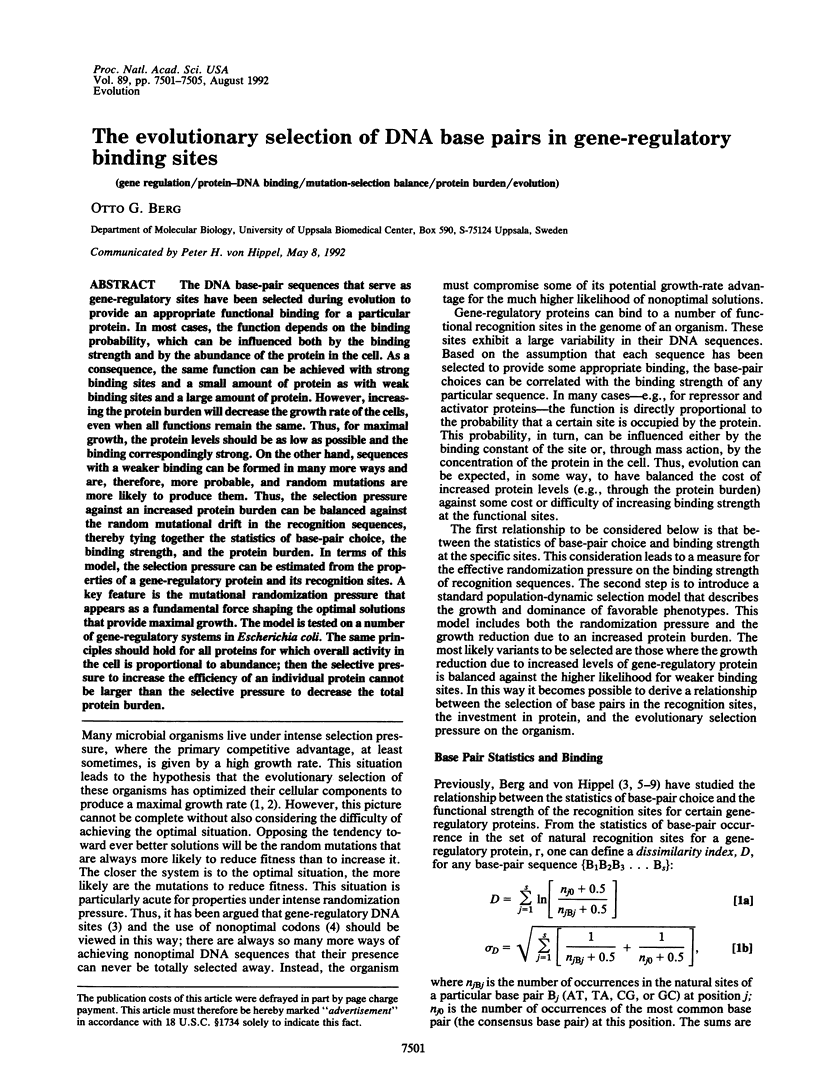
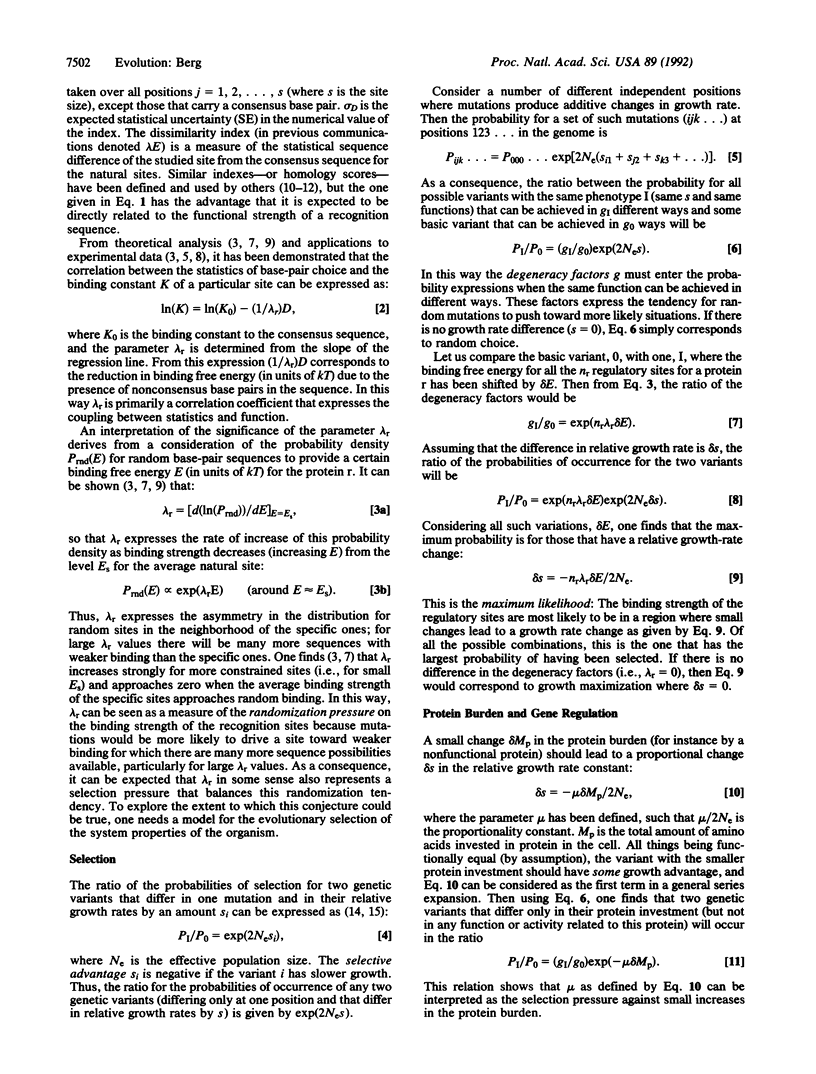
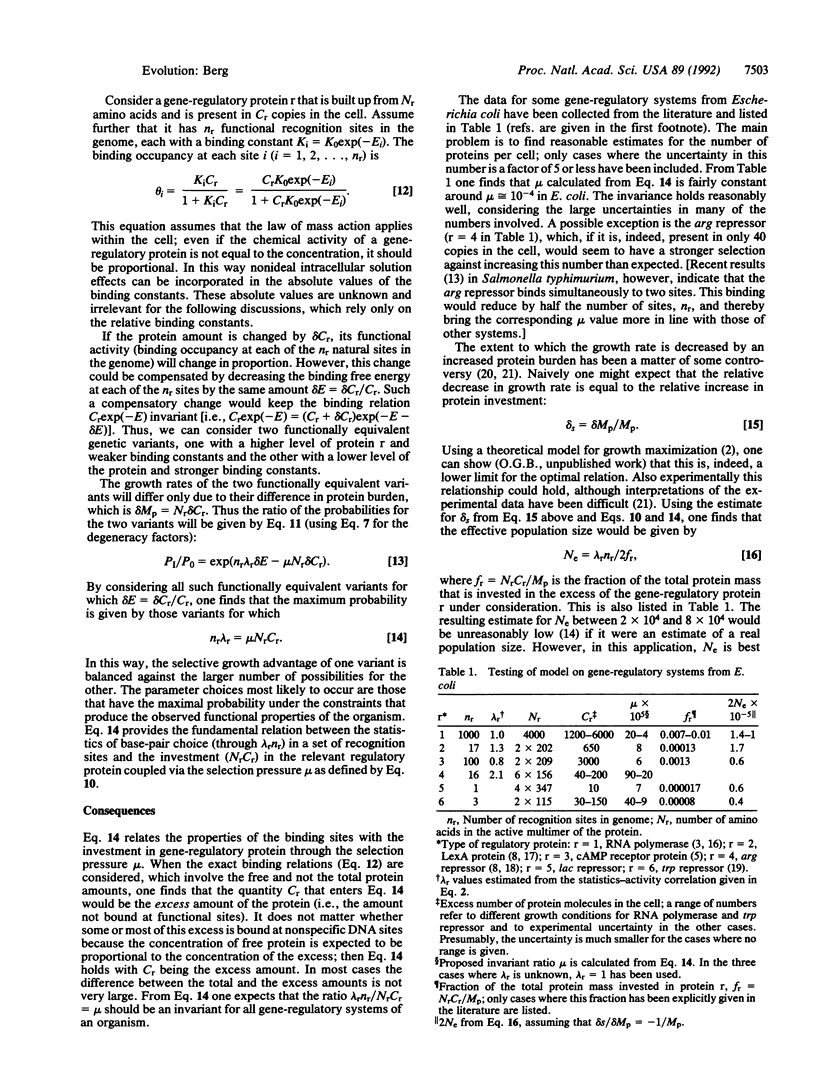
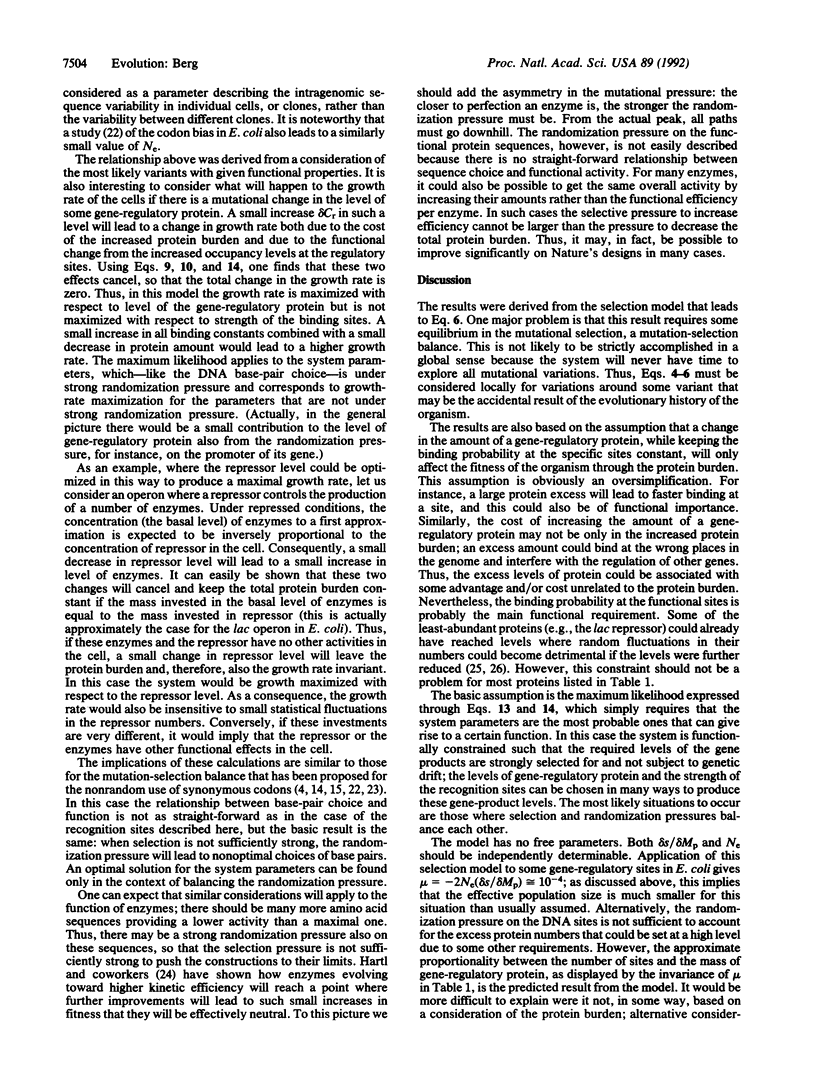
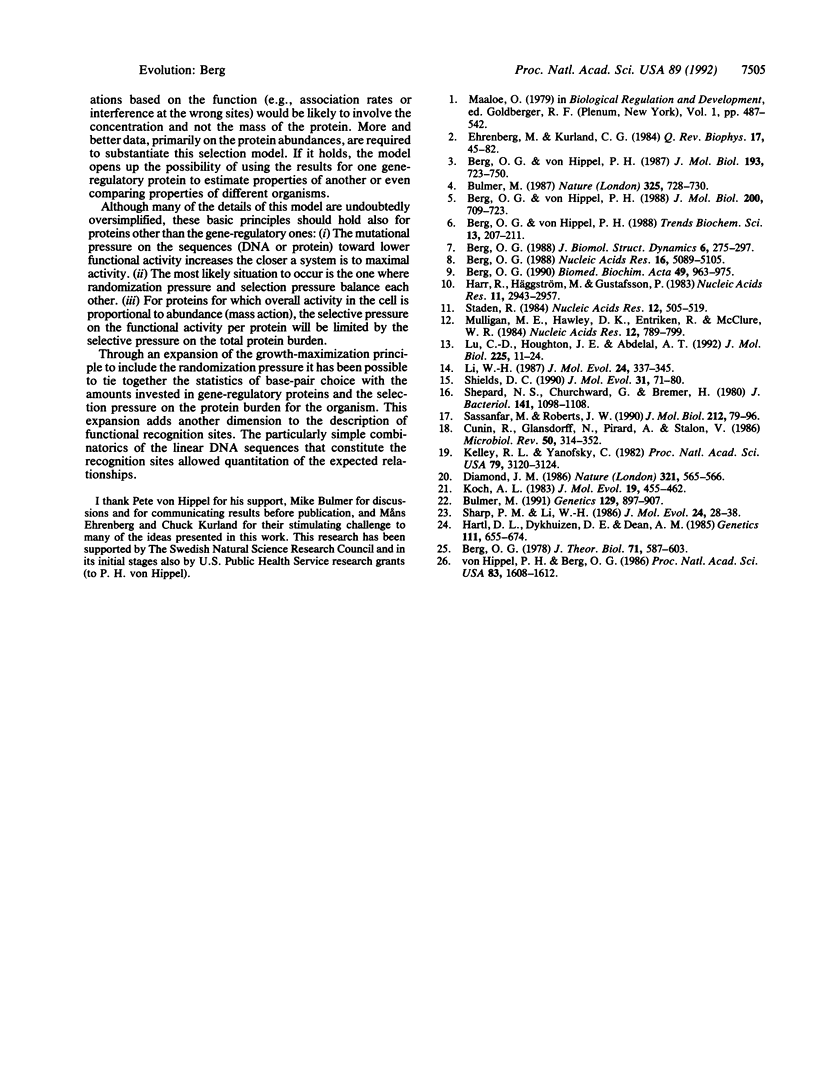
Selected References
These references are in PubMed. This may not be the complete list of references from this article.
- Berg O. G. A model for the statistical fluctuations of protein numbers in a microbial population. J Theor Biol. 1978 Apr 20;71(4):587–603. doi: 10.1016/0022-5193(78)90326-0. [DOI] [PubMed] [Google Scholar]
- Berg O. G. Base-pair specificity of protein-DNA recognition: a statistical-mechanical model. Biomed Biochim Acta. 1990;49(8-9):963–975. [PubMed] [Google Scholar]
- Berg O. G. Selection of DNA binding sites by regulatory proteins. Functional specificity and pseudosite competition. J Biomol Struct Dyn. 1988 Oct;6(2):275–297. doi: 10.1080/07391102.1988.10507713. [DOI] [PubMed] [Google Scholar]
- Berg O. G. Selection of DNA binding sites by regulatory proteins: the LexA protein and the arginine repressor use different strategies for functional specificity. Nucleic Acids Res. 1988 Jun 10;16(11):5089–5105. doi: 10.1093/nar/16.11.5089. [DOI] [PMC free article] [PubMed] [Google Scholar]
- Berg O. G., von Hippel P. H. Selection of DNA binding sites by regulatory proteins. II. The binding specificity of cyclic AMP receptor protein to recognition sites. J Mol Biol. 1988 Apr 20;200(4):709–723. doi: 10.1016/0022-2836(88)90482-2. [DOI] [PubMed] [Google Scholar]
- Berg O. G., von Hippel P. H. Selection of DNA binding sites by regulatory proteins. Statistical-mechanical theory and application to operators and promoters. J Mol Biol. 1987 Feb 20;193(4):723–750. doi: 10.1016/0022-2836(87)90354-8. [DOI] [PubMed] [Google Scholar]
- Berg O. G., von Hippel P. H. Selection of DNA binding sites by regulatory proteins. Trends Biochem Sci. 1988 Jun;13(6):207–211. doi: 10.1016/0968-0004(88)90085-0. [DOI] [PubMed] [Google Scholar]
- Bulmer M. Coevolution of codon usage and transfer RNA abundance. Nature. 1987 Feb 19;325(6106):728–730. doi: 10.1038/325728a0. [DOI] [PubMed] [Google Scholar]
- Bulmer M. The selection-mutation-drift theory of synonymous codon usage. Genetics. 1991 Nov;129(3):897–907. doi: 10.1093/genetics/129.3.897. [DOI] [PMC free article] [PubMed] [Google Scholar]
- Cunin R., Glansdorff N., Piérard A., Stalon V. Biosynthesis and metabolism of arginine in bacteria. Microbiol Rev. 1986 Sep;50(3):314–352. doi: 10.1128/mr.50.3.314-352.1986. [DOI] [PMC free article] [PubMed] [Google Scholar]
- Diamond J. M. Why do disused proteins become genetically lost or repressed? Nature. 1986 Jun 5;321(6070):565–566. doi: 10.1038/321565a0. [DOI] [PubMed] [Google Scholar]
- Ehrenberg M., Kurland C. G. Costs of accuracy determined by a maximal growth rate constraint. Q Rev Biophys. 1984 Feb;17(1):45–82. doi: 10.1017/s0033583500005254. [DOI] [PubMed] [Google Scholar]
- Harr R., Häggström M., Gustafsson P. Search algorithm for pattern match analysis of nucleic acid sequences. Nucleic Acids Res. 1983 May 11;11(9):2943–2957. doi: 10.1093/nar/11.9.2943. [DOI] [PMC free article] [PubMed] [Google Scholar]
- Hartl D. L., Dykhuizen D. E., Dean A. M. Limits of adaptation: the evolution of selective neutrality. Genetics. 1985 Nov;111(3):655–674. doi: 10.1093/genetics/111.3.655. [DOI] [PMC free article] [PubMed] [Google Scholar]
- Kelley R. L., Yanofsky C. Trp aporepressor production is controlled by autogenous regulation and inefficient translation. Proc Natl Acad Sci U S A. 1982 May;79(10):3120–3124. doi: 10.1073/pnas.79.10.3120. [DOI] [PMC free article] [PubMed] [Google Scholar]
- Koch A. L. The protein burden of lac operon products. J Mol Evol. 1983;19(6):455–462. doi: 10.1007/BF02102321. [DOI] [PubMed] [Google Scholar]
- Li W. H. Models of nearly neutral mutations with particular implications for nonrandom usage of synonymous codons. J Mol Evol. 1987;24(4):337–345. doi: 10.1007/BF02134132. [DOI] [PubMed] [Google Scholar]
- Lu C. D., Houghton J. E., Abdelal A. T. Characterization of the arginine repressor from Salmonella typhimurium and its interactions with the carAB operator. J Mol Biol. 1992 May 5;225(1):11–24. doi: 10.1016/0022-2836(92)91022-h. [DOI] [PubMed] [Google Scholar]
- Mulligan M. E., Hawley D. K., Entriken R., McClure W. R. Escherichia coli promoter sequences predict in vitro RNA polymerase selectivity. Nucleic Acids Res. 1984 Jan 11;12(1 Pt 2):789–800. doi: 10.1093/nar/12.1part2.789. [DOI] [PMC free article] [PubMed] [Google Scholar]
- Sassanfar M., Roberts J. W. Nature of the SOS-inducing signal in Escherichia coli. The involvement of DNA replication. J Mol Biol. 1990 Mar 5;212(1):79–96. doi: 10.1016/0022-2836(90)90306-7. [DOI] [PubMed] [Google Scholar]
- Sharp P. M., Li W. H. An evolutionary perspective on synonymous codon usage in unicellular organisms. J Mol Evol. 1986;24(1-2):28–38. doi: 10.1007/BF02099948. [DOI] [PubMed] [Google Scholar]
- Shepherd N. S., Churchward G., Bremer H. Synthesis and activity of ribonucleic acid polymerase in Escherichia coli. J Bacteriol. 1980 Mar;141(3):1098–1108. doi: 10.1128/jb.141.3.1098-1108.1980. [DOI] [PMC free article] [PubMed] [Google Scholar]
- Shields D. C. Switches in species-specific codon preferences: the influence of mutation biases. J Mol Evol. 1990 Aug;31(2):71–80. doi: 10.1007/BF02109476. [DOI] [PubMed] [Google Scholar]
- Staden R. Computer methods to locate signals in nucleic acid sequences. Nucleic Acids Res. 1984 Jan 11;12(1 Pt 2):505–519. doi: 10.1093/nar/12.1part2.505. [DOI] [PMC free article] [PubMed] [Google Scholar]
- von Hippel P. H., Berg O. G. On the specificity of DNA-protein interactions. Proc Natl Acad Sci U S A. 1986 Mar;83(6):1608–1612. doi: 10.1073/pnas.83.6.1608. [DOI] [PMC free article] [PubMed] [Google Scholar]