Abstract
The timing of neocortical regional specification was examined using a monoclonal antibody, designated PC3.1, that binds a 29-kDa polypeptide and recognizes a neuronal subpopulation located in the lateral but not dorsomedial neocortex in the rat. When lateral cortical tissue fragments at embryonic days 12 and 16 were maintained in an organotypic culture system, a substantial number of neurons became PC3.1-immunopositive. In marked contrast, considerably fewer, if any, PC3.1-positive neurons were observed in cultures of dorsal cortical tissue. The selective appearance of PC3.1-immunopositive neurons was also observed in dissociated cultures derived from the lateral, but not dorsal, cortical primordium at embryonic day 13 and later. In light of previous reports showing that the interactions between developing neocortical neurons and cortical afferents begin at embryonic day 14 or later, our findings imply that some regional specification occurs well before these interactions and suggest the importance of elements intrinsic to the neocortex in establishing neocortical regional specificity. Furthermore, [3H]thymidine birth-dating experiments revealed that the majority of presumptive PC3.1-immunopositive neurons underwent their final mitosis around embryonic day 15, suggesting that the regional specification events for these neurons occur before their neurogenesis.
Full text
PDF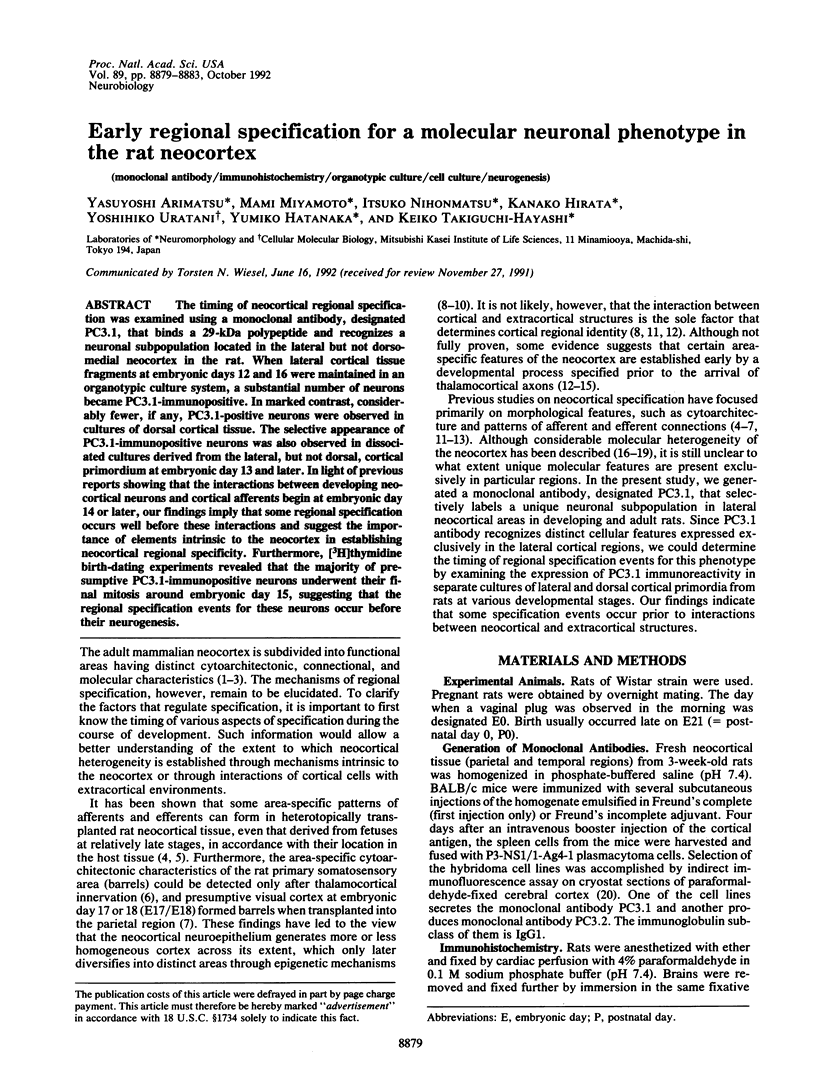
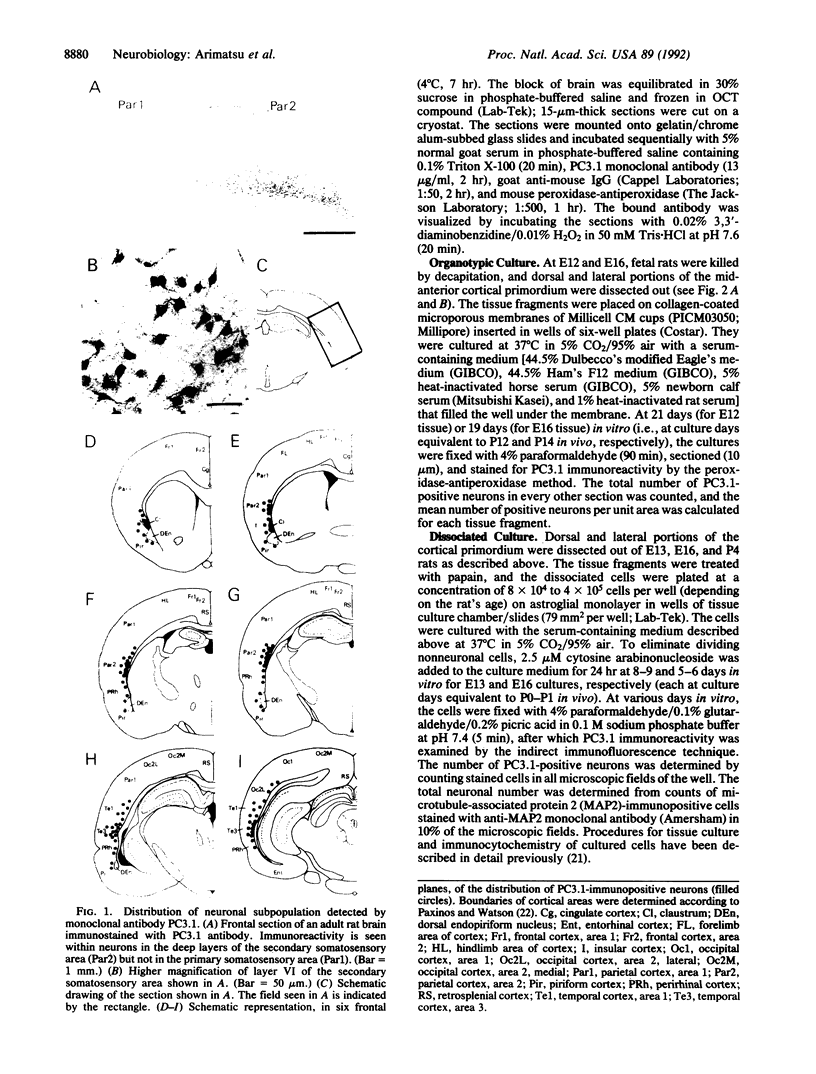
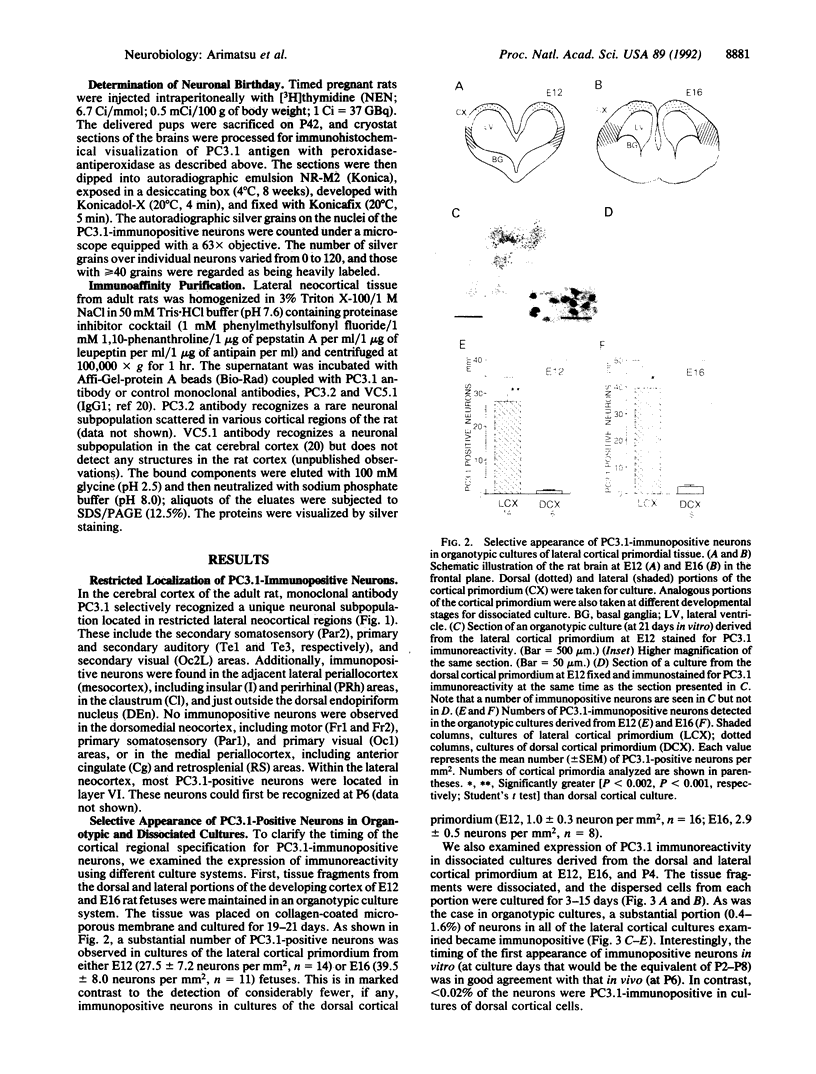
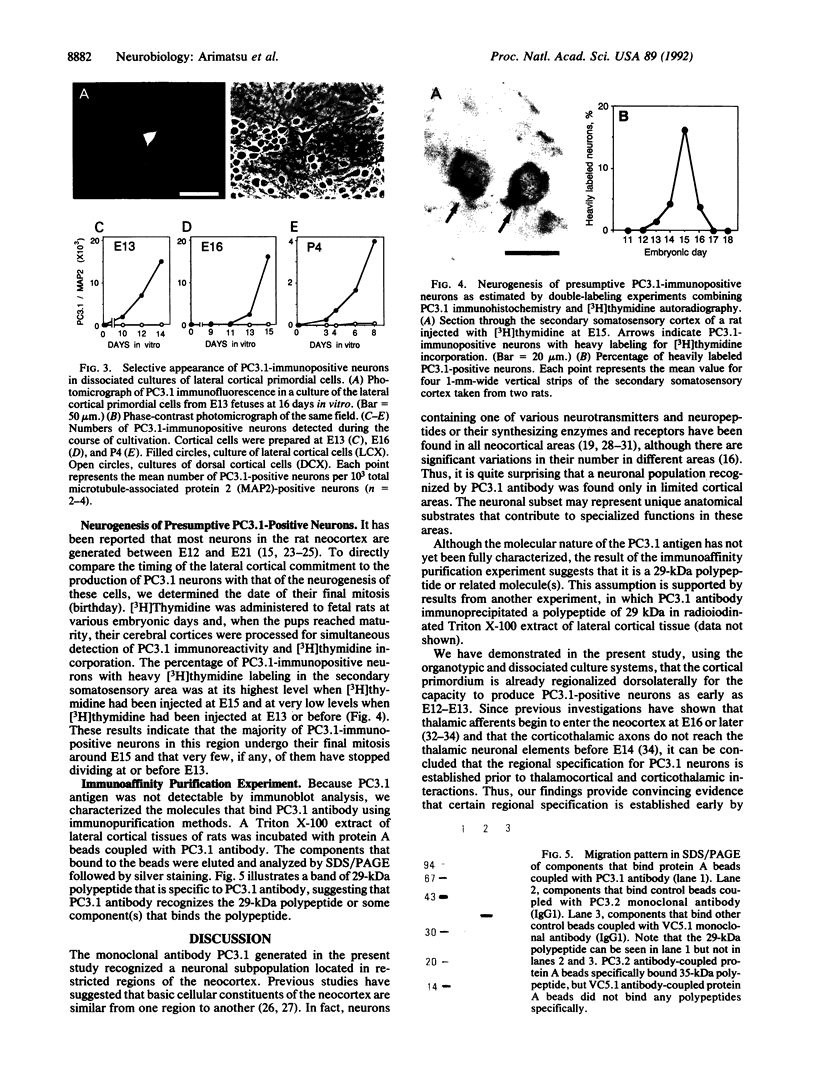
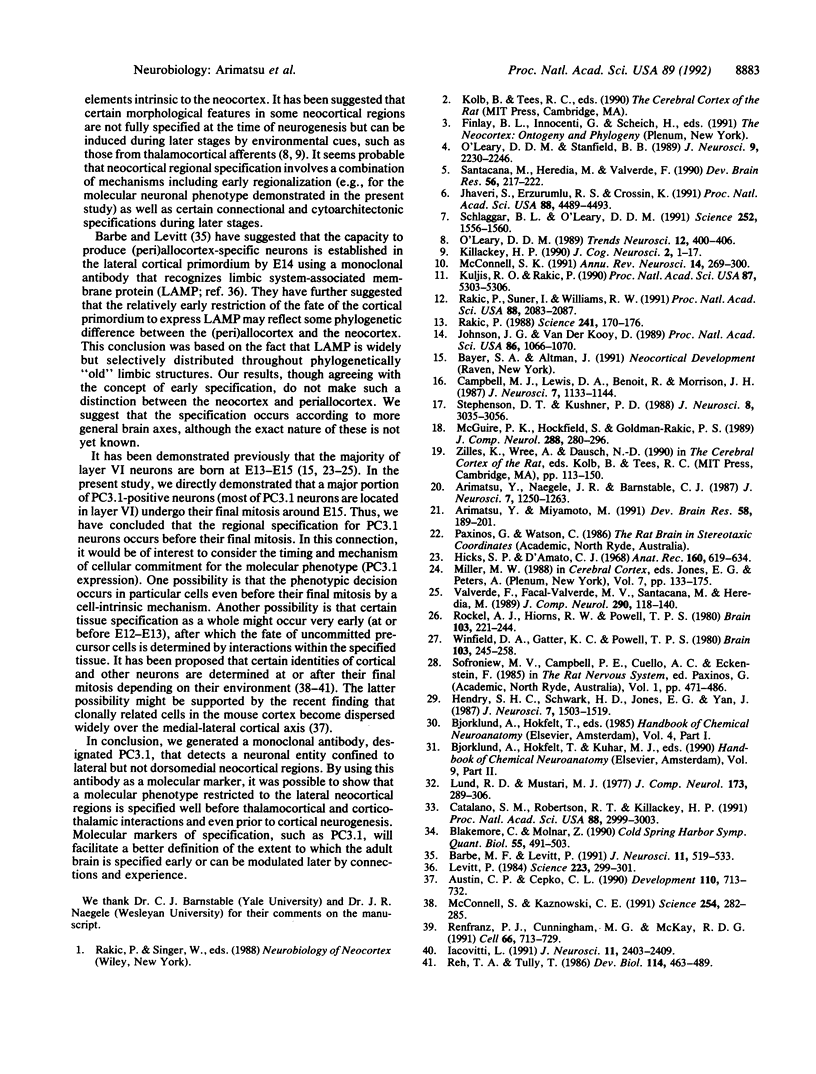
Images in this article
Selected References
These references are in PubMed. This may not be the complete list of references from this article.
- Arimatsu Y., Miyamoto M. Survival-promoting effect of NGF on in vitro septohippocampal neurons with cholinergic and GABAergic phenotypes. Brain Res Dev Brain Res. 1991 Feb 22;58(2):189–201. doi: 10.1016/0165-3806(91)90005-4. [DOI] [PubMed] [Google Scholar]
- Arimatsu Y., Naegele J. R., Barnstable C. J. Molecular markers of neuronal subpopulations in layers 4, 5, and 6 of cat primary visual cortex. J Neurosci. 1987 Apr;7(4):1250–1263. doi: 10.1523/JNEUROSCI.07-04-01250.1987. [DOI] [PMC free article] [PubMed] [Google Scholar]
- Austin C. P., Cepko C. L. Cellular migration patterns in the developing mouse cerebral cortex. Development. 1990 Nov;110(3):713–732. doi: 10.1242/dev.110.3.713. [DOI] [PubMed] [Google Scholar]
- Barbe M. F., Levitt P. The early commitment of fetal neurons to the limbic cortex. J Neurosci. 1991 Feb;11(2):519–533. doi: 10.1523/JNEUROSCI.11-02-00519.1991. [DOI] [PMC free article] [PubMed] [Google Scholar]
- Blakemore C., Molnár Z. Factors involved in the establishment of specific interconnections between thalamus and cerebral cortex. Cold Spring Harb Symp Quant Biol. 1990;55:491–504. doi: 10.1101/sqb.1990.055.01.048. [DOI] [PubMed] [Google Scholar]
- Campbell M. J., Lewis D. A., Benoit R., Morrison J. H. Regional heterogeneity in the distribution of somatostatin-28- and somatostatin-28(1-12)-immunoreactive profiles in monkey neocortex. J Neurosci. 1987 Apr;7(4):1133–1144. doi: 10.1523/JNEUROSCI.07-04-01133.1987. [DOI] [PMC free article] [PubMed] [Google Scholar]
- Catalano S. M., Robertson R. T., Killackey H. P. Early ingrowth of thalamocortical afferents to the neocortex of the prenatal rat. Proc Natl Acad Sci U S A. 1991 Apr 15;88(8):2999–3003. doi: 10.1073/pnas.88.8.2999. [DOI] [PMC free article] [PubMed] [Google Scholar]
- Hendry S. H., Schwark H. D., Jones E. G., Yan J. Numbers and proportions of GABA-immunoreactive neurons in different areas of monkey cerebral cortex. J Neurosci. 1987 May;7(5):1503–1519. doi: 10.1523/JNEUROSCI.07-05-01503.1987. [DOI] [PMC free article] [PubMed] [Google Scholar]
- Hicks S. P., D'Amato C. J. Cell migrations to the isocortex in the rat. Anat Rec. 1968 Mar;160(3):619–634. doi: 10.1002/ar.1091600311. [DOI] [PubMed] [Google Scholar]
- Iacovitti L. Effects of a novel differentiation factor on the development of catecholamine traits in noncatecholamine neurons from various regions of the rat brain: studies in tissue culture. J Neurosci. 1991 Aug;11(8):2403–2409. doi: 10.1523/JNEUROSCI.11-08-02403.1991. [DOI] [PMC free article] [PubMed] [Google Scholar]
- Jhaveri S., Erzurumlu R. S., Crossin K. Barrel construction in rodent neocortex: role of thalamic afferents versus extracellular matrix molecules. Proc Natl Acad Sci U S A. 1991 May 15;88(10):4489–4493. doi: 10.1073/pnas.88.10.4489. [DOI] [PMC free article] [PubMed] [Google Scholar]
- Johnston J. G., van der Kooy D. Protooncogene expression identifies a transient columnar organization of the forebrain within the late embryonic ventricular zone. Proc Natl Acad Sci U S A. 1989 Feb;86(3):1066–1070. doi: 10.1073/pnas.86.3.1066. [DOI] [PMC free article] [PubMed] [Google Scholar]
- Kuljis R. O., Rakic P. Hypercolumns in primate visual cortex can develop in the absence of cues from photoreceptors. Proc Natl Acad Sci U S A. 1990 Jul;87(14):5303–5306. doi: 10.1073/pnas.87.14.5303. [DOI] [PMC free article] [PubMed] [Google Scholar]
- Levitt P. A monoclonal antibody to limbic system neurons. Science. 1984 Jan 20;223(4633):299–301. doi: 10.1126/science.6199842. [DOI] [PubMed] [Google Scholar]
- Lund R. D., Mustari M. J. Development of the geniculocortical pathway in rats. J Comp Neurol. 1977 May 15;173(2):289–306. doi: 10.1002/cne.901730206. [DOI] [PubMed] [Google Scholar]
- McConnell S. K., Kaznowski C. E. Cell cycle dependence of laminar determination in developing neocortex. Science. 1991 Oct 11;254(5029):282–285. doi: 10.1126/science.254.5029.282. [DOI] [PubMed] [Google Scholar]
- McConnell S. K. The generation of neuronal diversity in the central nervous system. Annu Rev Neurosci. 1991;14:269–300. doi: 10.1146/annurev.ne.14.030191.001413. [DOI] [PubMed] [Google Scholar]
- McGuire P. K., Hockfield S., Goldman-Rakic P. S. Distribution of cat-301 immunoreactivity in the frontal and parietal lobes of the macaque monkey. J Comp Neurol. 1989 Oct 8;288(2):280–296. doi: 10.1002/cne.902880207. [DOI] [PubMed] [Google Scholar]
- O'Leary D. D. Do cortical areas emerge from a protocortex? Trends Neurosci. 1989 Oct;12(10):400–406. doi: 10.1016/0166-2236(89)90080-5. [DOI] [PubMed] [Google Scholar]
- O'Leary D. D., Stanfield B. B. Selective elimination of axons extended by developing cortical neurons is dependent on regional locale: experiments utilizing fetal cortical transplants. J Neurosci. 1989 Jul;9(7):2230–2246. doi: 10.1523/JNEUROSCI.09-07-02230.1989. [DOI] [PMC free article] [PubMed] [Google Scholar]
- Rakic P. Specification of cerebral cortical areas. Science. 1988 Jul 8;241(4862):170–176. doi: 10.1126/science.3291116. [DOI] [PubMed] [Google Scholar]
- Rakic P., Suñer I., Williams R. W. A novel cytoarchitectonic area induced experimentally within the primate visual cortex. Proc Natl Acad Sci U S A. 1991 Mar 15;88(6):2083–2087. doi: 10.1073/pnas.88.6.2083. [DOI] [PMC free article] [PubMed] [Google Scholar]
- Reh T. A., Tully T. Regulation of tyrosine hydroxylase-containing amacrine cell number in larval frog retina. Dev Biol. 1986 Apr;114(2):463–469. doi: 10.1016/0012-1606(86)90210-1. [DOI] [PubMed] [Google Scholar]
- Renfranz P. J., Cunningham M. G., McKay R. D. Region-specific differentiation of the hippocampal stem cell line HiB5 upon implantation into the developing mammalian brain. Cell. 1991 Aug 23;66(4):713–729. doi: 10.1016/0092-8674(91)90116-g. [DOI] [PubMed] [Google Scholar]
- Rockel A. J., Hiorns R. W., Powell T. P. The basic uniformity in structure of the neocortex. Brain. 1980 Jun;103(2):221–244. doi: 10.1093/brain/103.2.221. [DOI] [PubMed] [Google Scholar]
- Santacana M., Heredia M., Valverde F. Transplant connectivity in the rat cerebral cortex. A carbocyanine study. Brain Res Dev Brain Res. 1990 Nov 1;56(2):217–222. doi: 10.1016/0165-3806(90)90085-d. [DOI] [PubMed] [Google Scholar]
- Schlaggar B. L., O'Leary D. D. Potential of visual cortex to develop an array of functional units unique to somatosensory cortex. Science. 1991 Jun 14;252(5012):1556–1560. doi: 10.1126/science.2047863. [DOI] [PubMed] [Google Scholar]
- Stephenson D. T., Kushner P. D. An atlas of a rare neuronal surface antigen in the rat central nervous system. J Neurosci. 1988 Aug;8(8):3035–3056. doi: 10.1523/JNEUROSCI.08-08-03035.1988. [DOI] [PMC free article] [PubMed] [Google Scholar]
- Valverde F., Facal-Valverde M. V., Santacana M., Heredia M. Development and differentiation of early generated cells of sublayer VIb in the somatosensory cortex of the rat: a correlated Golgi and autoradiographic study. J Comp Neurol. 1989 Dec 1;290(1):118–140. doi: 10.1002/cne.902900108. [DOI] [PubMed] [Google Scholar]
- Winfield D. A., Gatter K. C., Powell T. P. An electron microscopic study of the types and proportions of neurons in the cortex of the motor and visual areas of the cat and rat. Brain. 1980 Jun;103(2):245–258. doi: 10.1093/brain/103.2.245. [DOI] [PubMed] [Google Scholar]