Abstract
We have identified a type of secondary structure for the homopurine oligomer d(A-G)10 below pH 6 in 0.01 M Na+ that is characterized by intense CD but only minor hypochromicity. The stability of this helix, designated d(A(+)-G)10, does not depend on oligomer concentration and increases sharply as ionic strength or pH drops, reaching a maximum at 4.0 (melting temperature, 37 degrees C). The pKa for the transition, 5.3 at 25 degrees C and even higher with decreasing temperature and [Na+], is much higher than the intrinsic pKa values for dA or dG residues. While the dA residues are protonated in the helix, further protonation of the dG residues disrupts it. When observed at 280 nm, melting of the helix first results in hypochromicity due to stacking of extrahelical dG residues with neighboring dA residues. The character and temperature dependence of the CD spectra of the constituent dinucleoside monophosphates indicate minimal chirality and base overlap for the A+pG sequences in d(A(+)-G)10 but left-handed twist with some base overlap for the GpA+ sequences. The observed properties are best satisfied by a model for an intramolecular helix with limited base overlap, stabilized by ionic bonds between dA residues protonated at N-1 and downstream negatively charged phosphates brought close due to the backbone helical twist, while Gsyn residues lie external to the helix. This structure could provide additional stabilizing energy for biologically relevant protonated non-B-DNA structures adopted by homopurine.homopyrimidine sequences due to topological stress or specific protein binding.
Full text
PDF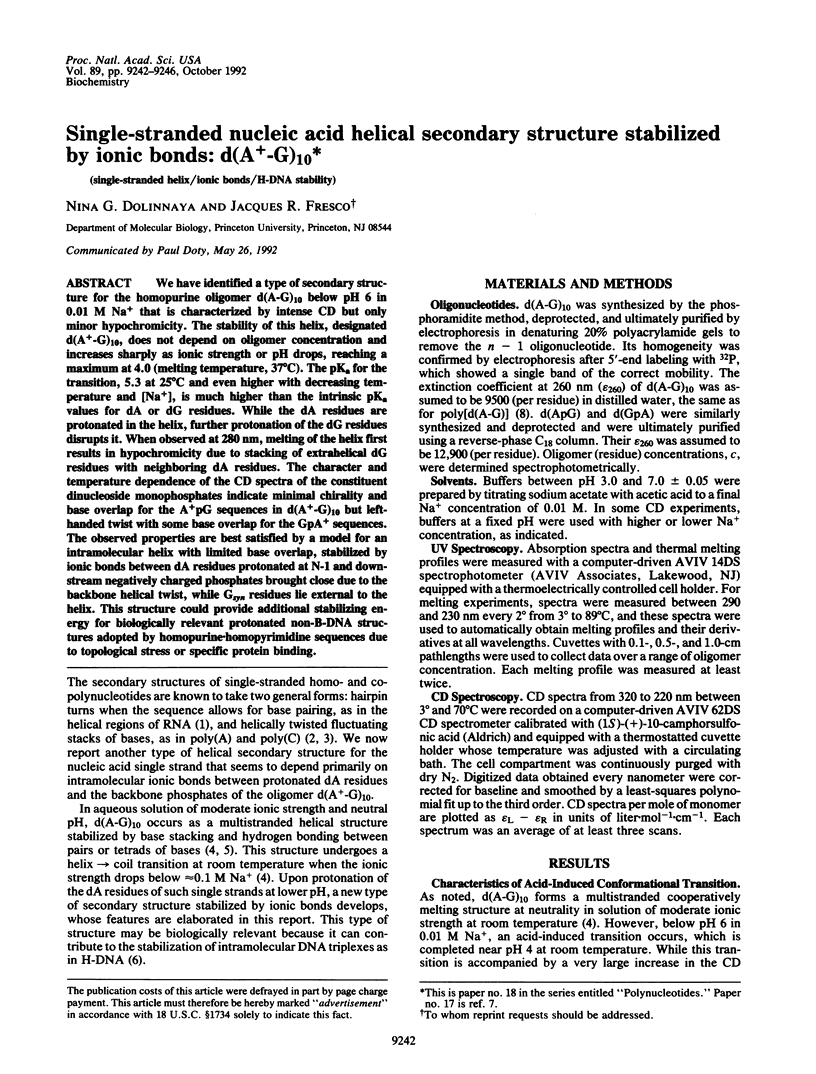
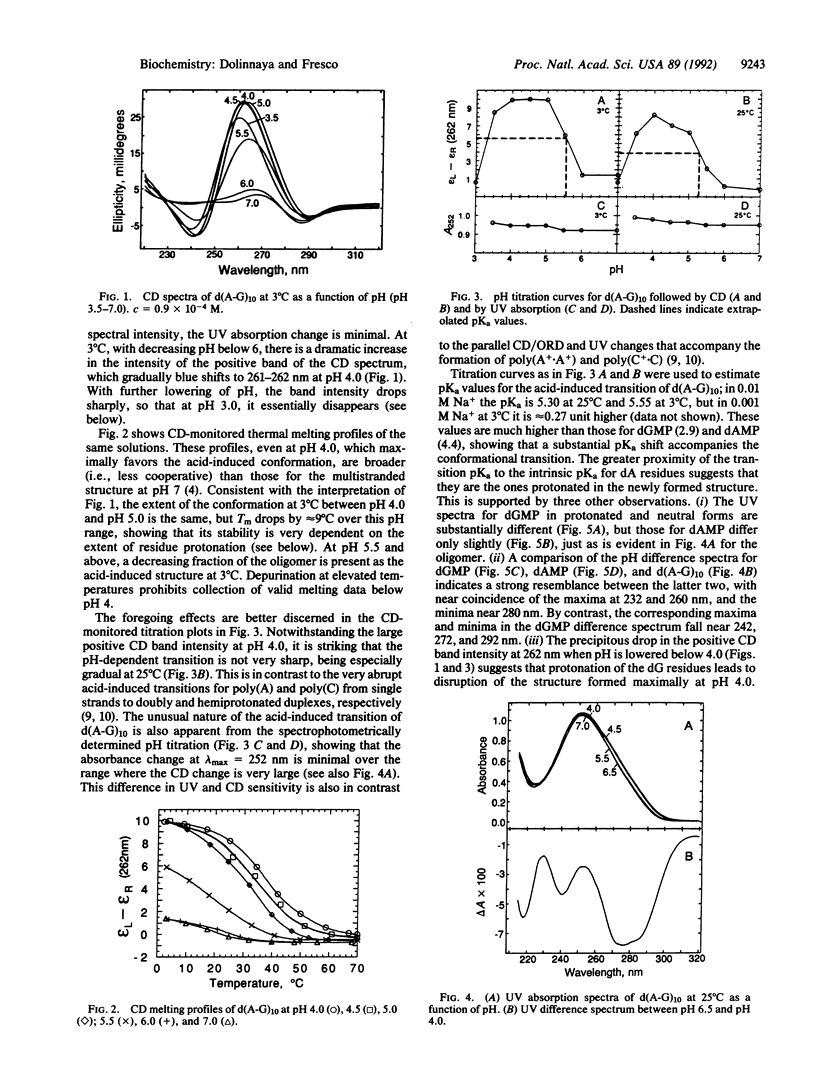
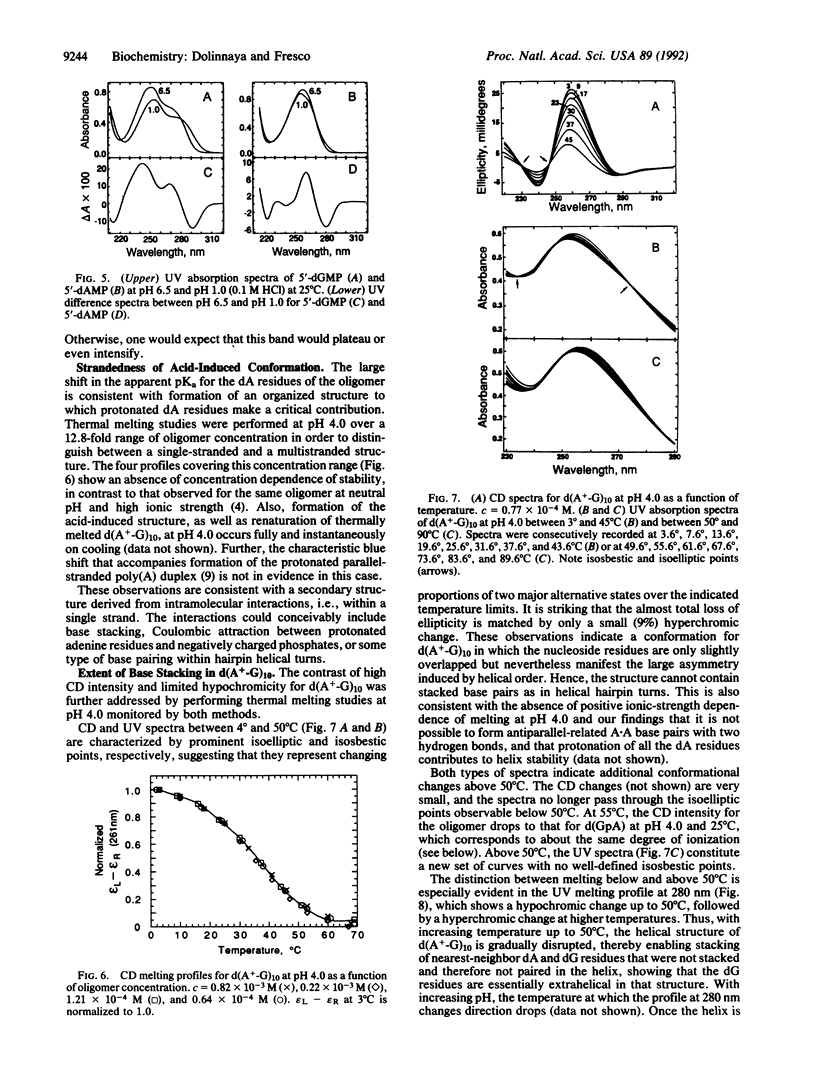
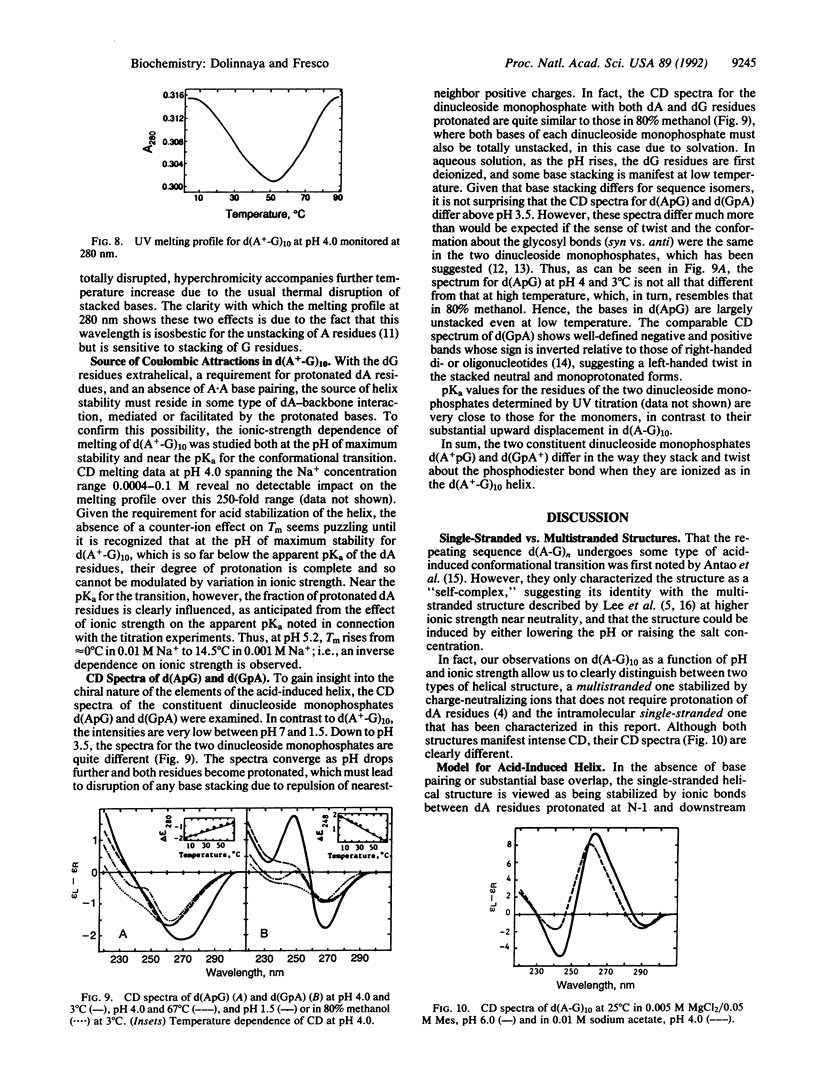
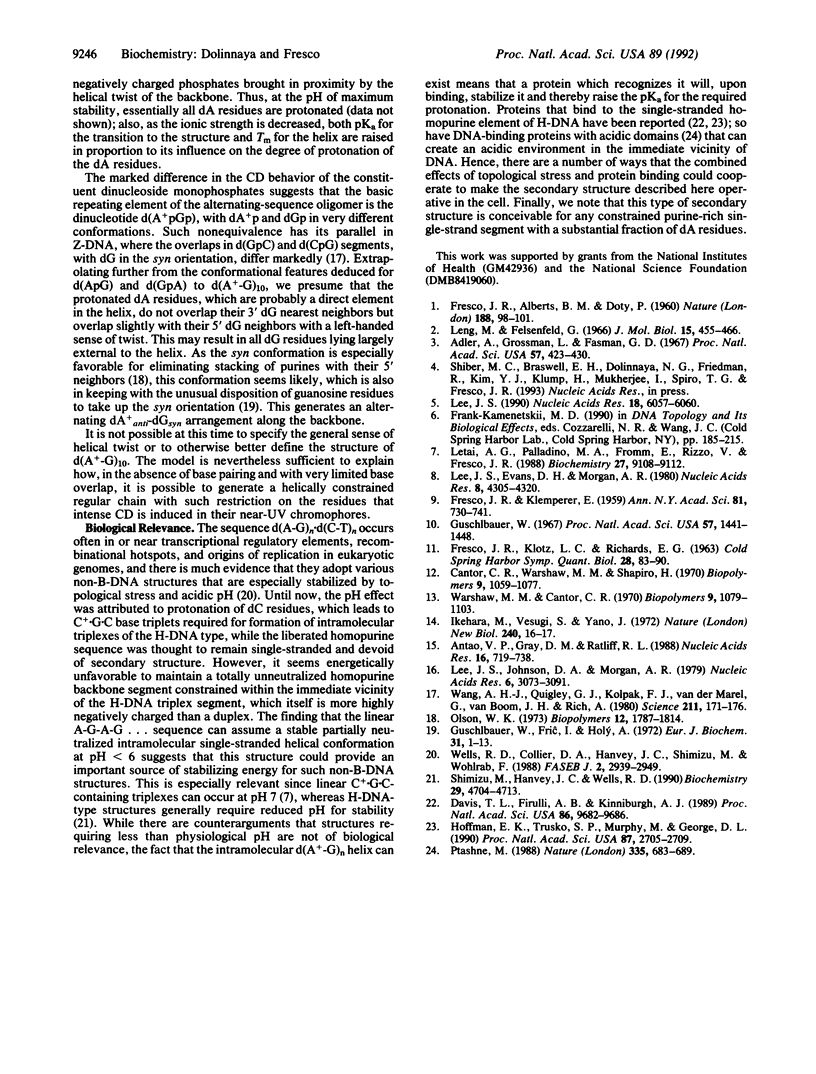
Selected References
These references are in PubMed. This may not be the complete list of references from this article.
- Adler B. A., Grossman L., Fasman G. D. Single-stranded oligomers and polymers of cytidylic and 2'-deoxycytidylic acids: comparative optical rotatory studies. Proc Natl Acad Sci U S A. 1967 Feb;57(2):423–430. doi: 10.1073/pnas.57.2.423. [DOI] [PMC free article] [PubMed] [Google Scholar]
- Antao V. P., Gray D. M., Ratliff R. L. CD of six different conformational rearrangements of poly[d(A-G).d(C-T)] induced by low pH. Nucleic Acids Res. 1988 Jan 25;16(2):719–738. doi: 10.1093/nar/16.2.719. [DOI] [PMC free article] [PubMed] [Google Scholar]
- Cantor C. R., Warshaw M. M., Shapiro H. Oligonucleotide interactions. 3. Circular dichroism studies of the conformation of deoxyoligonucleotides. Biopolymers. 1970;9(9):1059–1077. doi: 10.1002/bip.1970.360090909. [DOI] [PubMed] [Google Scholar]
- Davis T. L., Firulli A. B., Kinniburgh A. J. Ribonucleoprotein and protein factors bind to an H-DNA-forming c-myc DNA element: possible regulators of the c-myc gene. Proc Natl Acad Sci U S A. 1989 Dec;86(24):9682–9686. doi: 10.1073/pnas.86.24.9682. [DOI] [PMC free article] [PubMed] [Google Scholar]
- FRESCO J. R., ALBERTS B. M., DOTY P. Some molecular details of the secondary structure of ribonucleic acid. Nature. 1960 Oct 8;188:98–101. doi: 10.1038/188098a0. [DOI] [PubMed] [Google Scholar]
- FRESCO J. R., KLEMPERER E. Polyriboadenylic acid, a molecular analogue of ribonucleic acid and desoxyribonucleic acid. Ann N Y Acad Sci. 1959 Sep 4;81:730–741. doi: 10.1111/j.1749-6632.1959.tb49354.x. [DOI] [PubMed] [Google Scholar]
- Guschlbauer W., Fric I., Holý A. Oligonucleotide conformations. Optical studies on GpU analogues with modified-uridine residues. Eur J Biochem. 1972 Nov 21;31(1):1–13. doi: 10.1111/j.1432-1033.1972.tb02493.x. [DOI] [PubMed] [Google Scholar]
- Guschlbauer W. Protonated polynucleotide structures. I. The thermal denaturation of polycytidylic acid in acid solution. Proc Natl Acad Sci U S A. 1967 May;57(5):1441–1448. doi: 10.1073/pnas.57.5.1441. [DOI] [PMC free article] [PubMed] [Google Scholar]
- Hoffman E. K., Trusko S. P., Murphy M., George D. L. An S1 nuclease-sensitive homopurine/homopyrimidine domain in the c-Ki-ras promoter interacts with a nuclear factor. Proc Natl Acad Sci U S A. 1990 Apr;87(7):2705–2709. doi: 10.1073/pnas.87.7.2705. [DOI] [PMC free article] [PubMed] [Google Scholar]
- Ikehara M., Uesugi S., Yano J. Left-handed helical polynucleotides with D-sugar phosphodiester backbones. Nat New Biol. 1972 Nov 1;240(96):16–17. doi: 10.1038/newbio240016a0. [DOI] [PubMed] [Google Scholar]
- Lee J. S., Evans D. H., Morgan A. R. Polypurine DNAs and RNAs form secondary structures which may be tetra-stranded. Nucleic Acids Res. 1980 Sep 25;8(18):4305–4320. doi: 10.1093/nar/8.18.4305. [DOI] [PMC free article] [PubMed] [Google Scholar]
- Lee J. S., Johnson D. A., Morgan A. R. Complexes formed by (pyrimidine)n . (purine)n DNAs on lowering the pH are three-stranded. Nucleic Acids Res. 1979 Jul 11;6(9):3073–3091. doi: 10.1093/nar/6.9.3073. [DOI] [PMC free article] [PubMed] [Google Scholar]
- Lee J. S. The stability of polypurine tetraplexes in the presence of mono- and divalent cations. Nucleic Acids Res. 1990 Oct 25;18(20):6057–6060. doi: 10.1093/nar/18.20.6057. [DOI] [PMC free article] [PubMed] [Google Scholar]
- Leng M., Felsenfeld G. A study of polyadenylic acid at neutral pH. J Mol Biol. 1966 Feb;15(2):455–466. doi: 10.1016/s0022-2836(66)80121-3. [DOI] [PubMed] [Google Scholar]
- Letai A. G., Palladino M. A., Fromm E., Rizzo V., Fresco J. R. Specificity in formation of triple-stranded nucleic acid helical complexes: studies with agarose-linked polyribonucleotide affinity columns. Biochemistry. 1988 Dec 27;27(26):9108–9112. doi: 10.1021/bi00426a007. [DOI] [PubMed] [Google Scholar]
- Olson W. K. Syn-anti effects on the spatial configuration of polynucleotide chains. Biopolymers. 1973;12(8):1787–1814. doi: 10.1002/bip.1973.360120808. [DOI] [PubMed] [Google Scholar]
- Ptashne M. How eukaryotic transcriptional activators work. Nature. 1988 Oct 20;335(6192):683–689. doi: 10.1038/335683a0. [DOI] [PubMed] [Google Scholar]
- Shimizu M., Hanvey J. C., Wells R. D. Multiple non-B-DNA conformations of polypurine.polypyrimidine sequences in plasmids. Biochemistry. 1990 May 15;29(19):4704–4713. doi: 10.1021/bi00471a027. [DOI] [PubMed] [Google Scholar]
- Wang A. J., Quigley G. J., Kolpak F. J., van der Marel G., van Boom J. H., Rich A. Left-handed double helical DNA: variations in the backbone conformation. Science. 1981 Jan 9;211(4478):171–176. doi: 10.1126/science.7444458. [DOI] [PubMed] [Google Scholar]
- Warshaw M. M., Cantor C. R. Oligonucleotide interactions. IV. Conformational differences between deoxy- and ribodinucleoside phosphates. Biopolymers. 1970;9(9):1079–1103. doi: 10.1002/bip.1970.360090910. [DOI] [PubMed] [Google Scholar]
- Wells R. D., Collier D. A., Hanvey J. C., Shimizu M., Wohlrab F. The chemistry and biology of unusual DNA structures adopted by oligopurine.oligopyrimidine sequences. FASEB J. 1988 Nov;2(14):2939–2949. [PubMed] [Google Scholar]