Abstract
Using a lactose permease mutant devoid of Cys residue ("C-less permease"), we systematically replaced putative intramembrane charged residues with Cys. Individual replacements for Asp-237, Asp-240, Glu-269, Arg-302, Lys-319, His-322, Glu-325, or Lys-358 abolish active lactose transport. When Asp-237 and Lys-358 are simultaneously replaced with Cys and/or Ala, however, high activity is observed. Therefore, when either Asp-237 or Lys-358 is replaced with a neutral residue, leaving an unpaired charge, the permease is inactivated, but neutral replacement of both residues yields active permease [King, S. C., Hansen, C. L. & Wilson, T. H. (1991) Biochim. Biophys. Acta 1062, 177-186]. Remarkably, moreover, when Asp-237 is interchanged with Lys-358, high activity is observed. The observations provide a strong indication that Asp-237 and Lys-358 interact to form a salt bridge. In addition, the data demonstrate that neither residue nor the salt bridge plays an important role in the transport mechanism. Thirteen additional double mutants were constructed in which a negative and a positively charged residue were replaced with Cys. Only Asp-240-->Cys/Lys-319-->Cys exhibits significant activity, accumulating lactose to 25-30% of the steady state observed with C-less permease. Replacing either Asp-240 or Lys-319 individually with Ala also inactivates the permease, but double mutants with neutral substitutions (Cys and/or Ala) at both positions exhibit essentially the same activity as Asp-240-->Cys/Lys-319-->Cys. In marked contrast to Asp-237 and Lys-358, interchanging Asp-240 and Lys-319 abolishes active lactose transport. The results demonstrate that Asp-240 and Lys-319, like Asp-237 and Lys-358, interact functionally and may form a salt bridge. However, the interaction between Asp-240 and Lys-319 is clearly more complex than the interaction between Asp-237 and Lys-358. In any event, the findings suggest that putative transmembrane helix VII lies next to helices X and XI in the tertiary structure of lactose permease.
Full text
PDF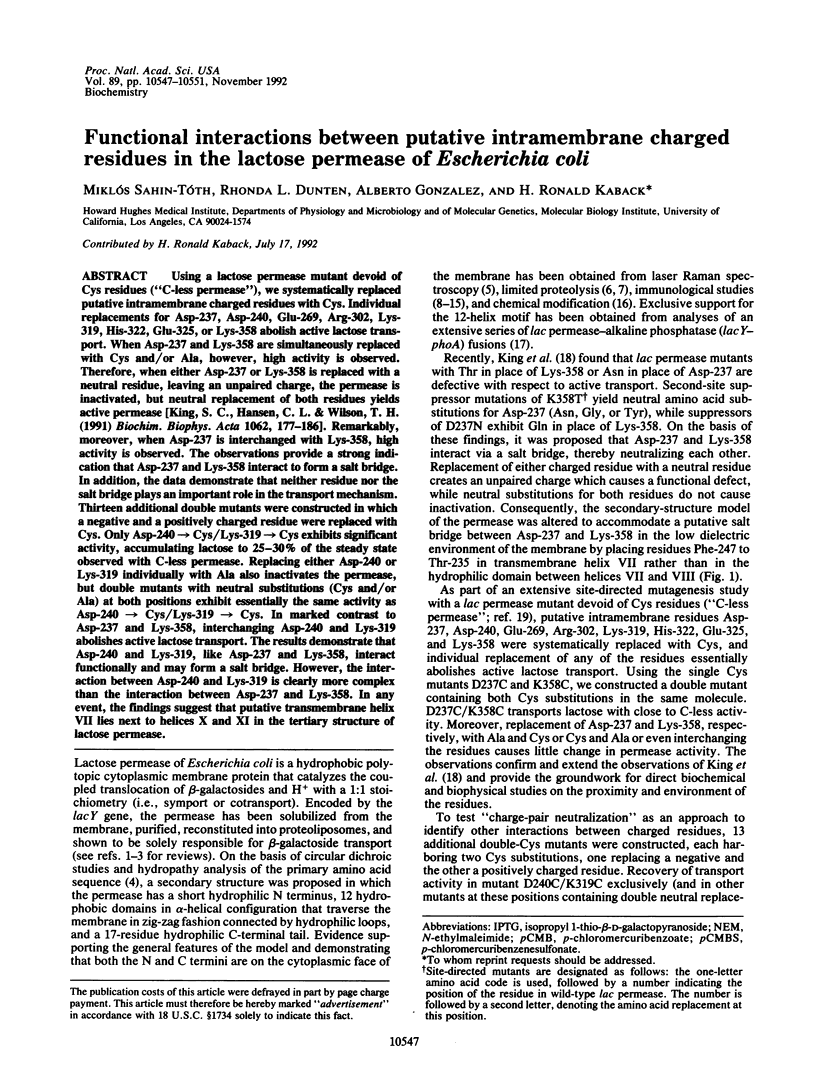
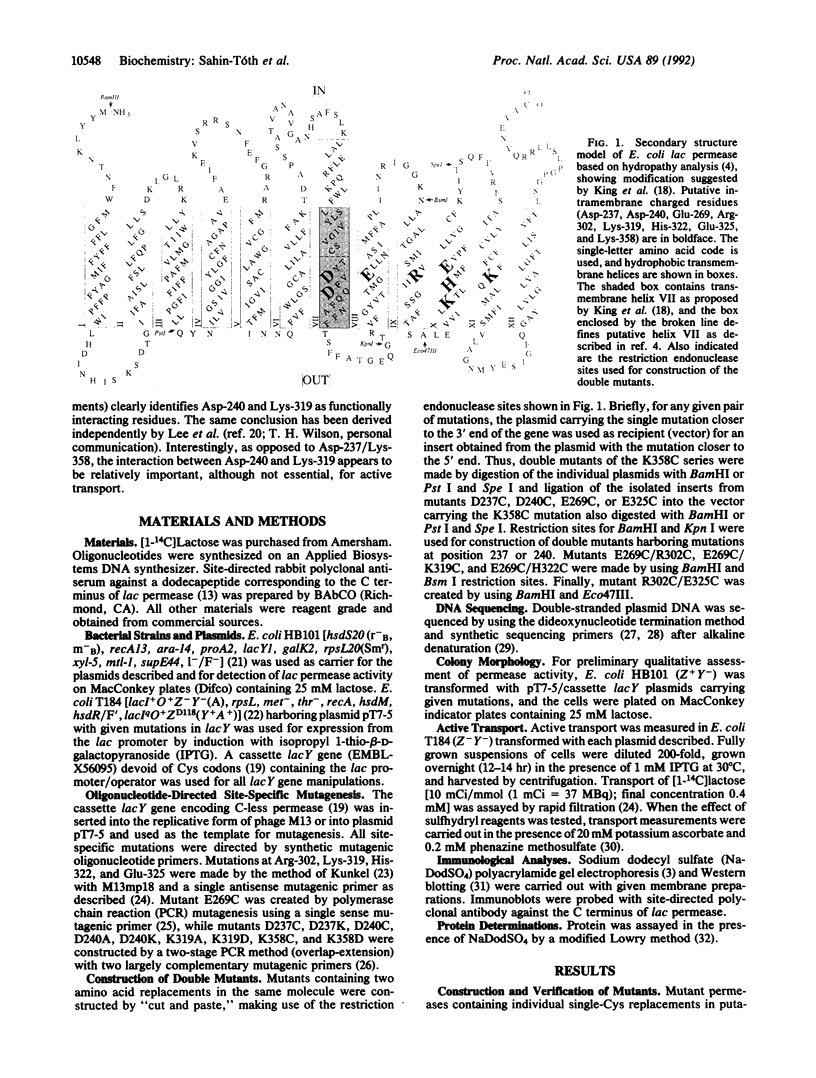
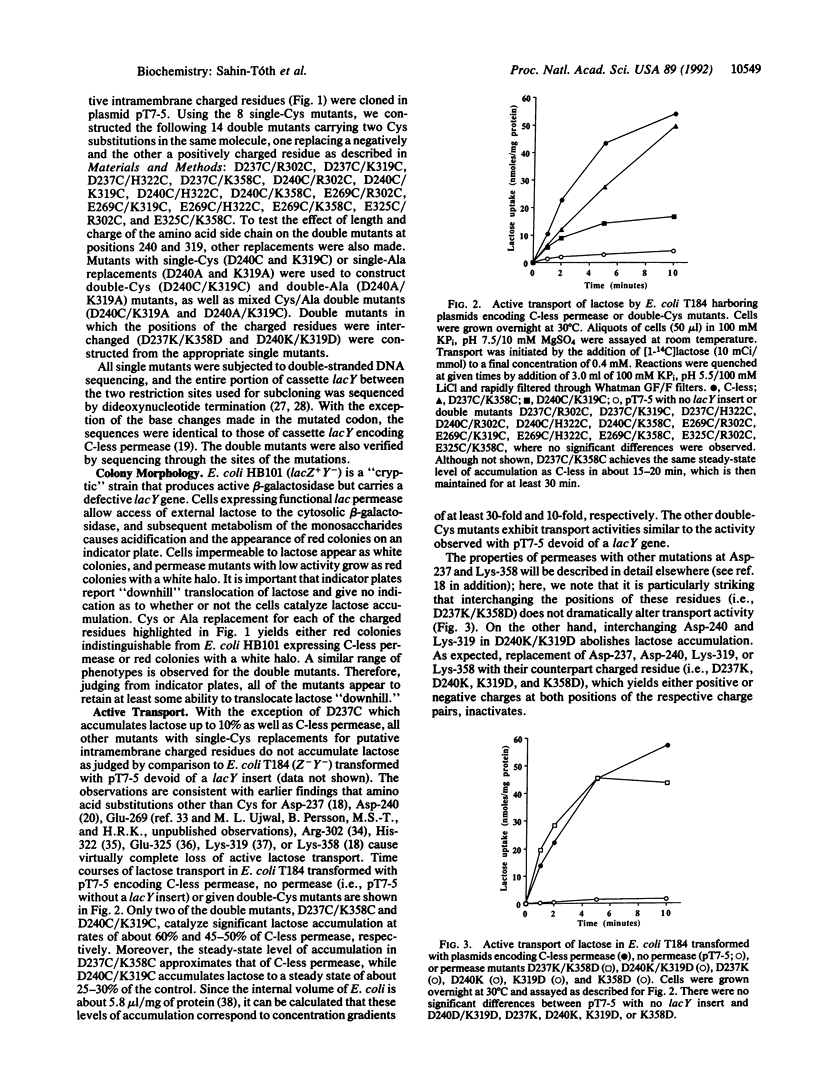
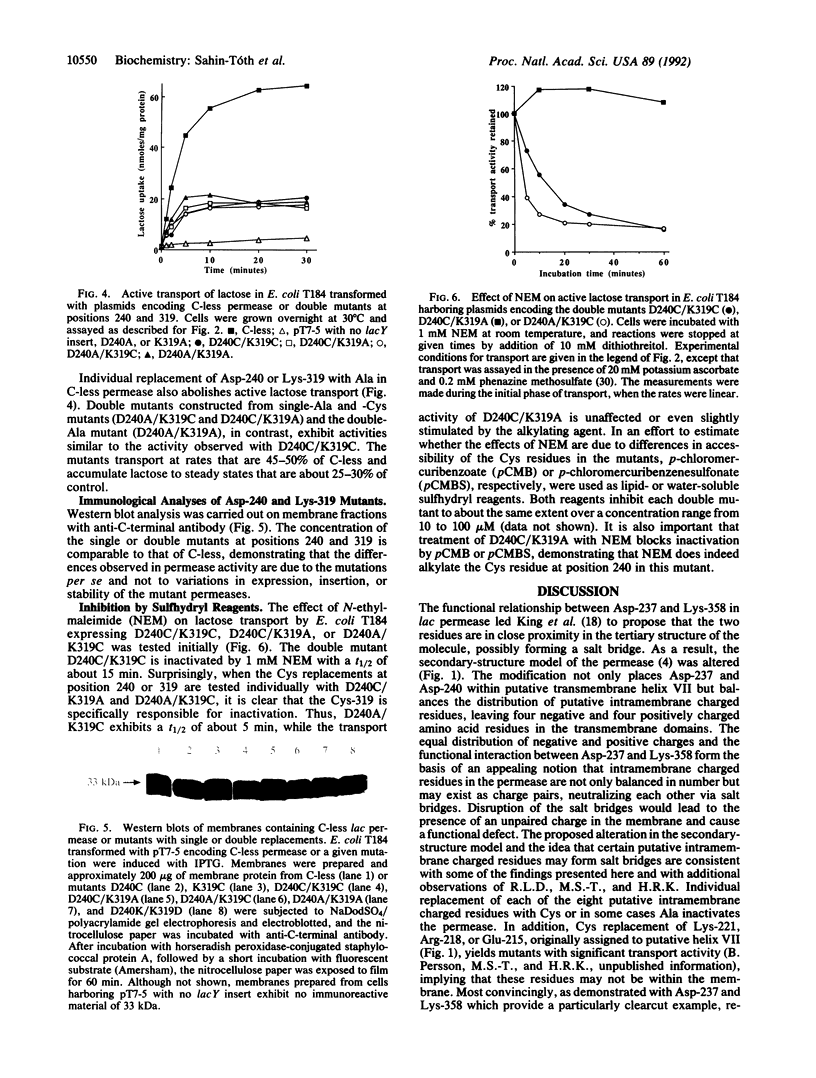
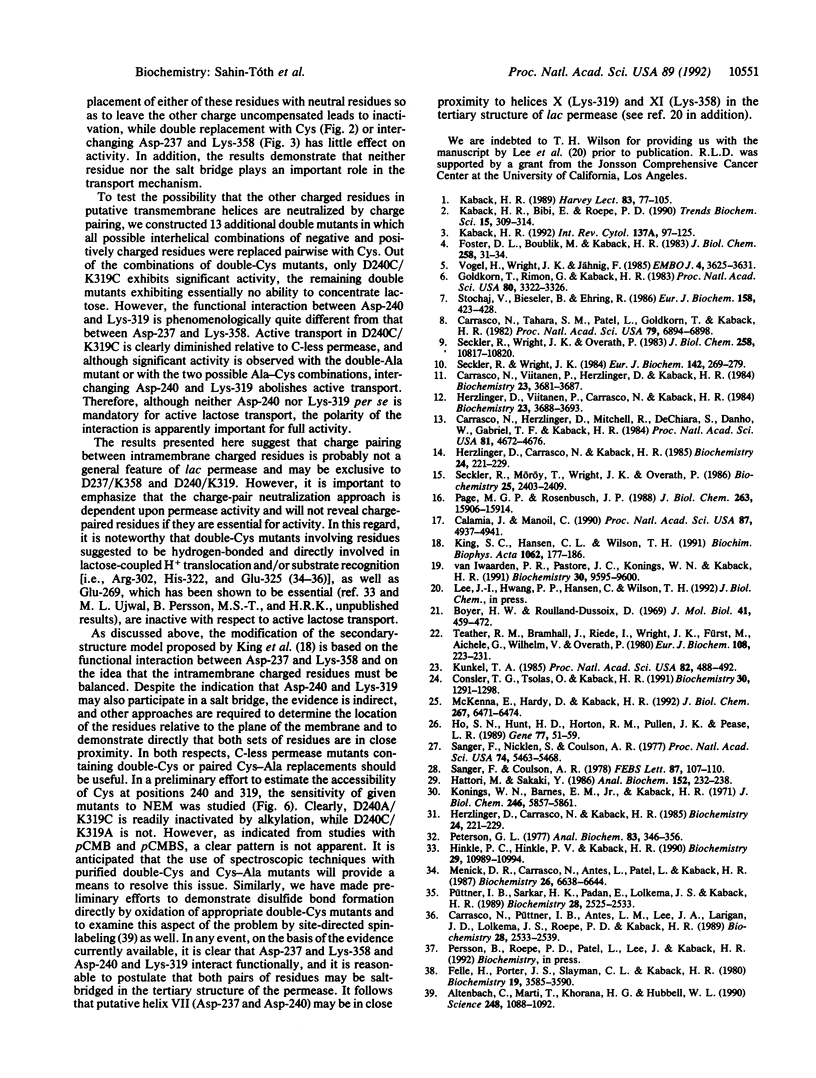
Images in this article
Selected References
These references are in PubMed. This may not be the complete list of references from this article.
- Altenbach C., Marti T., Khorana H. G., Hubbell W. L. Transmembrane protein structure: spin labeling of bacteriorhodopsin mutants. Science. 1990 Jun 1;248(4959):1088–1092. doi: 10.1126/science.2160734. [DOI] [PubMed] [Google Scholar]
- Boyer H. W., Roulland-Dussoix D. A complementation analysis of the restriction and modification of DNA in Escherichia coli. J Mol Biol. 1969 May 14;41(3):459–472. doi: 10.1016/0022-2836(69)90288-5. [DOI] [PubMed] [Google Scholar]
- Calamia J., Manoil C. lac permease of Escherichia coli: topology and sequence elements promoting membrane insertion. Proc Natl Acad Sci U S A. 1990 Jul;87(13):4937–4941. doi: 10.1073/pnas.87.13.4937. [DOI] [PMC free article] [PubMed] [Google Scholar]
- Carrasco N., Herzlinger D., Mitchell R., DeChiara S., Danho W., Gabriel T. F., Kaback H. R. Intramolecular dislocation of the COOH terminus of the lac carrier protein in reconstituted proteoliposomes. Proc Natl Acad Sci U S A. 1984 Aug;81(15):4672–4676. doi: 10.1073/pnas.81.15.4672. [DOI] [PMC free article] [PubMed] [Google Scholar]
- Carrasco N., Püttner I. B., Antes L. M., Lee J. A., Larigan J. D., Lolkema J. S., Roepe P. D., Kaback H. R. Characterization of site-directed mutants in the lac permease of Escherichia coli. 2. Glutamate-325 replacements. Biochemistry. 1989 Mar 21;28(6):2533–2539. doi: 10.1021/bi00432a028. [DOI] [PubMed] [Google Scholar]
- Carrasco N., Tahara S. M., Patel L., Goldkorn T., Kaback H. R. Preparation, characterization, and properties of monoclonal antibodies against the lac carrier protein from Escherichia coli. Proc Natl Acad Sci U S A. 1982 Nov;79(22):6894–6898. doi: 10.1073/pnas.79.22.6894. [DOI] [PMC free article] [PubMed] [Google Scholar]
- Carrasco N., Viitanen P., Herzlinger D., Kaback H. R. Monoclonal antibodies against the lac carrier protein from Escherichia coli. 1. Functional studies. Biochemistry. 1984 Jul 31;23(16):3681–3687. doi: 10.1021/bi00311a017. [DOI] [PubMed] [Google Scholar]
- Consler T. G., Tsolas O., Kaback H. R. Role of proline residues in the structure and function of a membrane transport protein. Biochemistry. 1991 Feb 5;30(5):1291–1298. doi: 10.1021/bi00219a019. [DOI] [PubMed] [Google Scholar]
- Felle H., Porter J. S., Slayman C. L., Kaback H. R. Quantitative measurements of membrane potential in Escherichia coli. Biochemistry. 1980 Jul 22;19(15):3585–3590. doi: 10.1021/bi00556a026. [DOI] [PubMed] [Google Scholar]
- Foster D. L., Boublik M., Kaback H. R. Structure of the lac carrier protein of Escherichia coli. J Biol Chem. 1983 Jan 10;258(1):31–34. [PubMed] [Google Scholar]
- Goldkorn T., Rimon G., Kaback H. R. Topology of the lac carrier protein in the membrane of Escherichia coli. Proc Natl Acad Sci U S A. 1983 Jun;80(11):3322–3326. doi: 10.1073/pnas.80.11.3322. [DOI] [PMC free article] [PubMed] [Google Scholar]
- Hattori M., Sakaki Y. Dideoxy sequencing method using denatured plasmid templates. Anal Biochem. 1986 Feb 1;152(2):232–238. doi: 10.1016/0003-2697(86)90403-3. [DOI] [PubMed] [Google Scholar]
- Herzlinger D., Carrasco N., Kaback H. R. Functional and immunochemical characterization of a mutant of Escherichia coli energy uncoupled for lactose transport. Biochemistry. 1985 Jan 1;24(1):221–229. doi: 10.1021/bi00322a032. [DOI] [PubMed] [Google Scholar]
- Herzlinger D., Carrasco N., Kaback H. R. Functional and immunochemical characterization of a mutant of Escherichia coli energy uncoupled for lactose transport. Biochemistry. 1985 Jan 1;24(1):221–229. doi: 10.1021/bi00322a032. [DOI] [PubMed] [Google Scholar]
- Herzlinger D., Viitanen P., Carrasco N., Kaback H. R. Monoclonal antibodies against the lac carrier protein from Escherichia coli. 2. Binding studies with membrane vesicles and proteoliposomes reconstituted with purified lac carrier protein. Biochemistry. 1984 Jul 31;23(16):3688–3693. doi: 10.1021/bi00311a018. [DOI] [PubMed] [Google Scholar]
- Hinkle P. C., Hinkle P. V., Kaback H. R. Information content of amino acid residues in putative helix VIII of the lac permease from Escherichia coli. Biochemistry. 1990 Dec 11;29(49):10989–10994. doi: 10.1021/bi00501a017. [DOI] [PubMed] [Google Scholar]
- Ho S. N., Hunt H. D., Horton R. M., Pullen J. K., Pease L. R. Site-directed mutagenesis by overlap extension using the polymerase chain reaction. Gene. 1989 Apr 15;77(1):51–59. doi: 10.1016/0378-1119(89)90358-2. [DOI] [PubMed] [Google Scholar]
- Kaback H. R., Bibi E., Roepe P. D. Beta-galactoside transport in E. coli: a functional dissection of lac permease. Trends Biochem Sci. 1990 Aug;15(8):309–314. doi: 10.1016/0968-0004(90)90020-c. [DOI] [PubMed] [Google Scholar]
- Kaback H. R. In and out and up and down with lac permease. Int Rev Cytol. 1992;137:97–125. doi: 10.1016/s0074-7696(08)62674-1. [DOI] [PubMed] [Google Scholar]
- Kaback H. R. Molecular biology of active transport: from membrane to molecule to mechanism. Harvey Lect. 1987;83:77–105. [PubMed] [Google Scholar]
- King S. C., Hansen C. L., Wilson T. H. The interaction between aspartic acid 237 and lysine 358 in the lactose carrier of Escherichia coli. Biochim Biophys Acta. 1991 Feb 25;1062(2):177–186. doi: 10.1016/0005-2736(91)90390-t. [DOI] [PubMed] [Google Scholar]
- Konings W. N., Barnes E. M., Jr, Kaback H. R. Mechanisms of active transport in isolated membrane vesicles. 2. The coupling of reduced phenazine methosulfate to the concentrative uptake of beta-galactosides and amino acids. J Biol Chem. 1971 Oct 10;246(19):5857–5861. [PubMed] [Google Scholar]
- Kunkel T. A. Rapid and efficient site-specific mutagenesis without phenotypic selection. Proc Natl Acad Sci U S A. 1985 Jan;82(2):488–492. doi: 10.1073/pnas.82.2.488. [DOI] [PMC free article] [PubMed] [Google Scholar]
- McKenna E., Hardy D., Kaback H. R. Evidence that the final turn of the last transmembrane helix in the lactose permease is required for folding. J Biol Chem. 1992 Apr 5;267(10):6471–6474. [PubMed] [Google Scholar]
- Menick D. R., Carrasco N., Antes L., Patel L., Kaback H. R. lac permease of Escherichia coli: arginine-302 as a component of the postulated proton relay. Biochemistry. 1987 Oct 20;26(21):6638–6644. doi: 10.1021/bi00395a012. [DOI] [PubMed] [Google Scholar]
- Page M. G., Rosenbusch J. P. Topography of lactose permease from Escherichia coli. J Biol Chem. 1988 Nov 5;263(31):15906–15914. [PubMed] [Google Scholar]
- Peterson G. L. A simplification of the protein assay method of Lowry et al. which is more generally applicable. Anal Biochem. 1977 Dec;83(2):346–356. doi: 10.1016/0003-2697(77)90043-4. [DOI] [PubMed] [Google Scholar]
- Püttner I. B., Sarkar H. K., Padan E., Lolkema J. S., Kaback H. R. Characterization of site-directed mutants in the lac permease of Escherichia coli. 1. Replacement of histidine residues. Biochemistry. 1989 Mar 21;28(6):2525–2533. doi: 10.1021/bi00432a027. [DOI] [PubMed] [Google Scholar]
- Sanger F., Coulson A. R. The use of thin acrylamide gels for DNA sequencing. FEBS Lett. 1978 Mar 1;87(1):107–110. doi: 10.1016/0014-5793(78)80145-8. [DOI] [PubMed] [Google Scholar]
- Sanger F., Nicklen S., Coulson A. R. DNA sequencing with chain-terminating inhibitors. Proc Natl Acad Sci U S A. 1977 Dec;74(12):5463–5467. doi: 10.1073/pnas.74.12.5463. [DOI] [PMC free article] [PubMed] [Google Scholar]
- Seckler R., Möröy T., Wright J. K., Overath P. Anti-peptide antibodies and proteases as structural probes for the lactose/H+ transporter of Escherichia coli: a loop around amino acid residue 130 faces the cytoplasmic side of the membrane. Biochemistry. 1986 May 6;25(9):2403–2409. doi: 10.1021/bi00357a016. [DOI] [PubMed] [Google Scholar]
- Seckler R., Wright J. K., Overath P. Peptide-specific antibody locates the COOH terminus of the lactose carrier of Escherichia coli on the cytoplasmic side of the plasma membrane. J Biol Chem. 1983 Sep 25;258(18):10817–10820. [PubMed] [Google Scholar]
- Seckler R., Wright J. K. Sidedness of native membrane vesicles of Escherichia coli and orientation of the reconstituted lactose: H+ carrier. Eur J Biochem. 1984 Jul 16;142(2):269–279. doi: 10.1111/j.1432-1033.1984.tb08281.x. [DOI] [PubMed] [Google Scholar]
- Stochaj U., Bieseler B., Ehring R. Limited proteolysis of lactose permease from Escherichia coli. Eur J Biochem. 1986 Jul 15;158(2):423–428. doi: 10.1111/j.1432-1033.1986.tb09770.x. [DOI] [PubMed] [Google Scholar]
- Teather R. M., Bramhall J., Riede I., Wright J. K., Fürst M., Aichele G., Wilhelm U., Overath P. Lactose carrier protein of Escherichia coli. Structure and expression of plasmids carrying the Y gene of the lac operon. Eur J Biochem. 1980;108(1):223–231. doi: 10.1111/j.1432-1033.1980.tb04715.x. [DOI] [PubMed] [Google Scholar]
- Vogel H., Wright J. K., Jähnig F. The structure of the lactose permease derived from Raman spectroscopy and prediction methods. EMBO J. 1985 Dec 16;4(13A):3625–3631. doi: 10.1002/j.1460-2075.1985.tb04126.x. [DOI] [PMC free article] [PubMed] [Google Scholar]
- van Iwaarden P. R., Pastore J. C., Konings W. N., Kaback H. R. Construction of a functional lactose permease devoid of cysteine residues. Biochemistry. 1991 Oct 8;30(40):9595–9600. doi: 10.1021/bi00104a005. [DOI] [PubMed] [Google Scholar]