Abstract
Spherical giant liposomes that had encapsulated skeletal-muscle G-actin were made by swelling a dried lipid mixture of dimyristoyl phosphatidylcholine/cardiolipin, 1:1 (wt/wt), in a solution of G-actin/CaCl2 at 0 degree C. Polymerization of the encapsulated G-actin into actin filaments was achieved by raising the temperature to 30 degrees C. We observed the subsequent shape changes of the liposomes by dark-field and differential interference-contrast light microscopy. After approximately 40 min, which was required for completion of actin polymerization, two shapes of liposome were evident: dumbbell and disk. Elongation of the dumbbell-shaped liposomes was concomitant with actin polymerization. Polarization microscopy showed that actin filaments formed thick bundles in the liposomes and that these filaments lay contiguous to the periphery of the liposome. Localization of actin filaments in the liposomes was confirmed by observation of rhodamine phalloidin-conjugated actin filaments by fluorescence microscopy. Both dumbbell- and disk-shaped liposomes were rigid and kept their shapes as far as actin filaments were stabilized. In contrast, liposomes containing bovine serum albumin were fragile, and their shapes continually fluctuated from Brownian motion, indicating that the actin bundles served as mechanical support for the liposome shapes.
Full text
PDF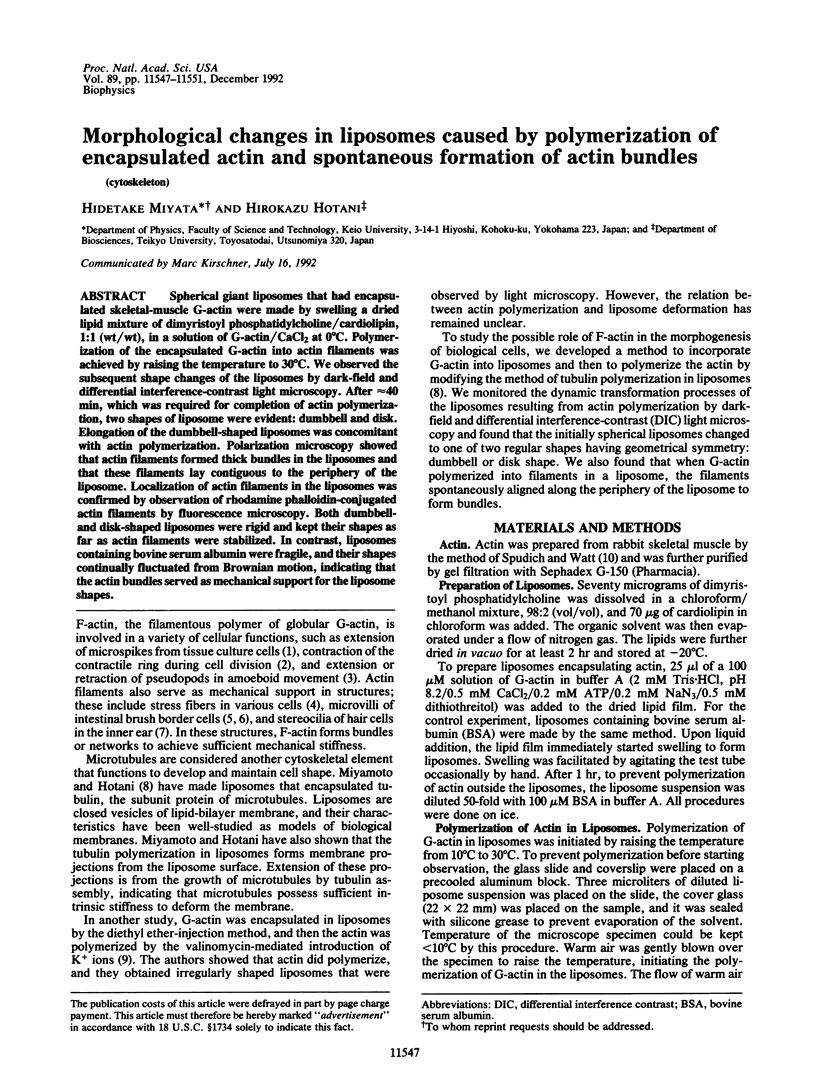
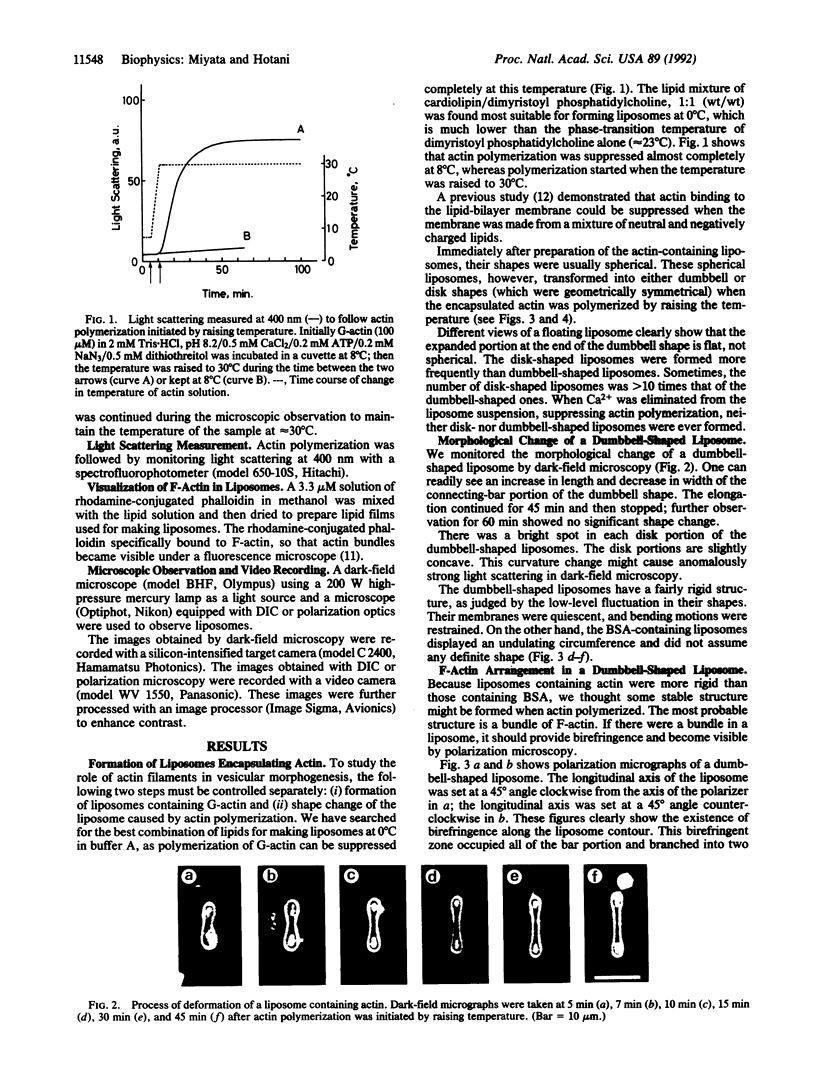
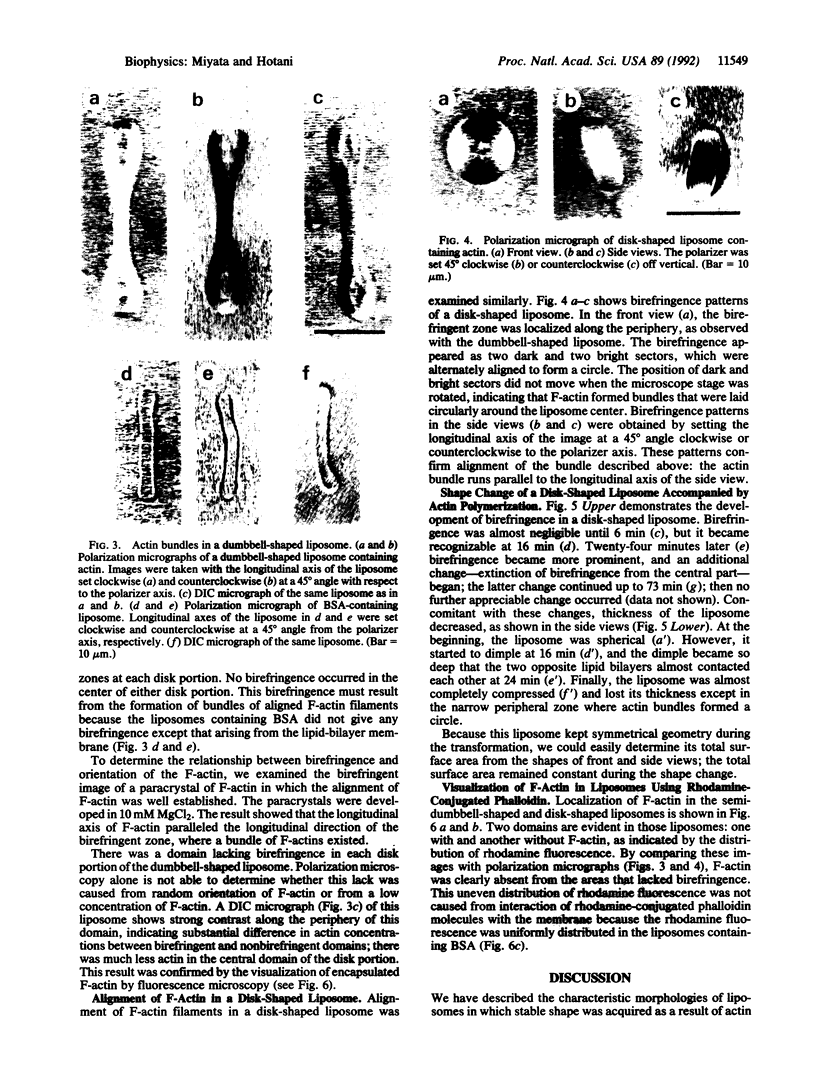
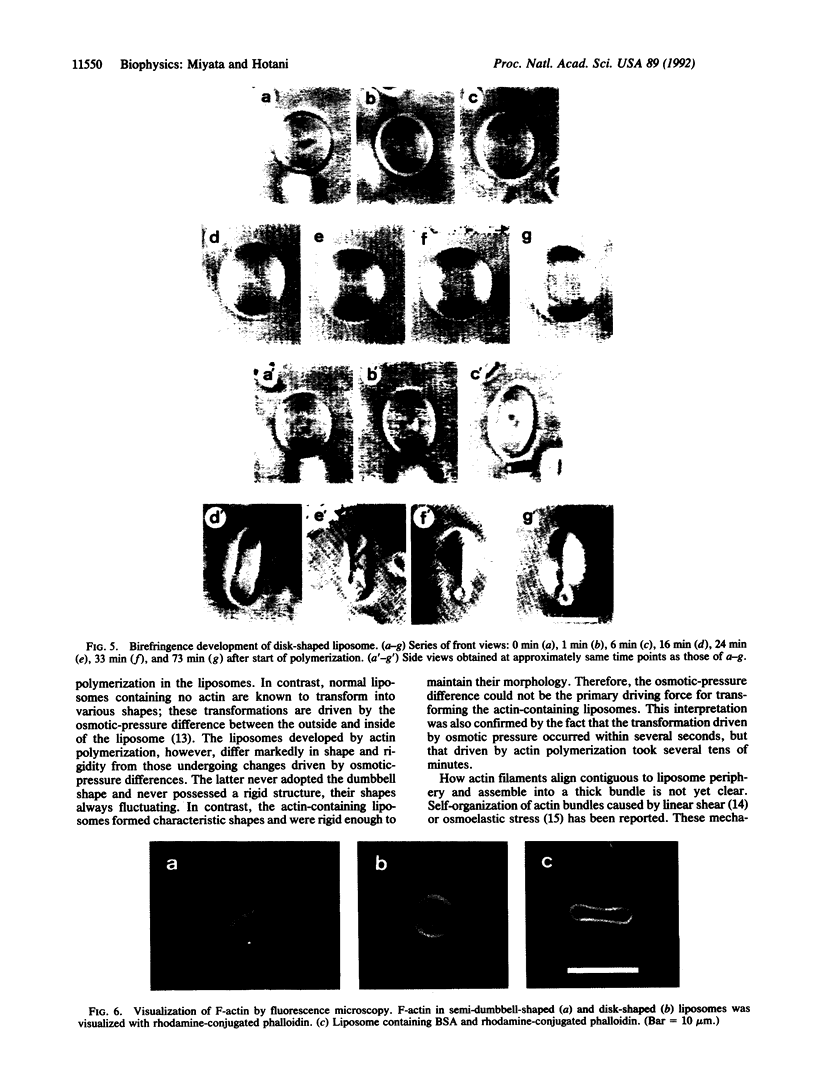
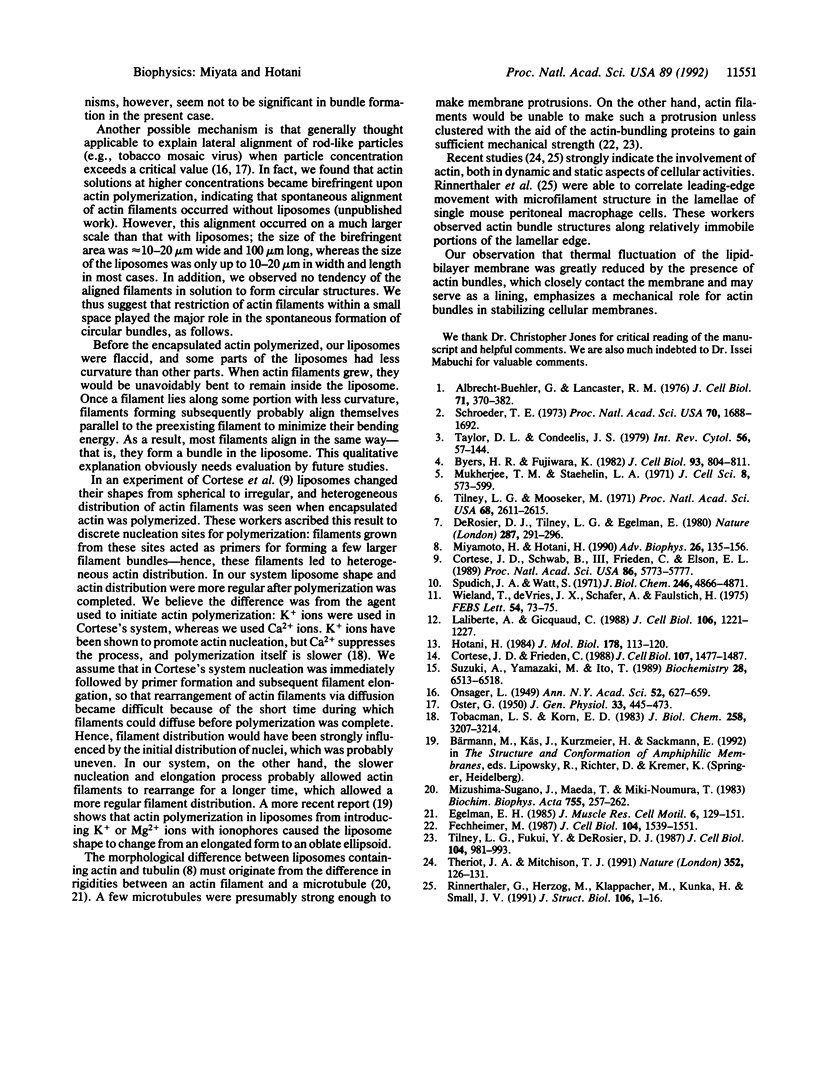
Images in this article
Selected References
These references are in PubMed. This may not be the complete list of references from this article.
- Albrecht-Buehler G., Lancaster R. M. A quantitative description of the extension and retraction of surface protrusions in spreading 3T3 mouse fibroblasts. J Cell Biol. 1976 Nov;71(2):370–382. doi: 10.1083/jcb.71.2.370. [DOI] [PMC free article] [PubMed] [Google Scholar]
- Byers H. R., Fujiwara K. Stress fibers in cells in situ: immunofluorescence visualization with antiactin, antimyosin, and anti-alpha-actinin. J Cell Biol. 1982 Jun;93(3):804–811. doi: 10.1083/jcb.93.3.804. [DOI] [PMC free article] [PubMed] [Google Scholar]
- Cortese J. D., Frieden C. Microheterogeneity of actin gels formed under controlled linear shear. J Cell Biol. 1988 Oct;107(4):1477–1487. doi: 10.1083/jcb.107.4.1477. [DOI] [PMC free article] [PubMed] [Google Scholar]
- Cortese J. D., Schwab B., 3rd, Frieden C., Elson E. L. Actin polymerization induces a shape change in actin-containing vesicles. Proc Natl Acad Sci U S A. 1989 Aug;86(15):5773–5777. doi: 10.1073/pnas.86.15.5773. [DOI] [PMC free article] [PubMed] [Google Scholar]
- DeRosier D. J., Tilney L. G., Egelman E. Actin in the inner ear: the remarkable structure of the stereocilium. Nature. 1980 Sep 25;287(5780):291–296. doi: 10.1038/287291a0. [DOI] [PubMed] [Google Scholar]
- Egelman E. H. The structure of F-actin. J Muscle Res Cell Motil. 1985 Apr;6(2):129–151. doi: 10.1007/BF00713056. [DOI] [PubMed] [Google Scholar]
- Fechheimer M. The Dictyostelium discoideum 30,000-dalton protein is an actin filament-bundling protein that is selectively present in filopodia. J Cell Biol. 1987 Jun;104(6):1539–1551. doi: 10.1083/jcb.104.6.1539. [DOI] [PMC free article] [PubMed] [Google Scholar]
- Hotani H., Miyamoto H. Dynamic features of microtubules as visualized by dark-field microscopy. Adv Biophys. 1990;26:135–156. doi: 10.1016/0065-227x(90)90010-q. [DOI] [PubMed] [Google Scholar]
- Hotani H. Transformation pathways of liposomes. J Mol Biol. 1984 Sep 5;178(1):113–120. doi: 10.1016/0022-2836(84)90234-1. [DOI] [PubMed] [Google Scholar]
- LURIE M. B. The use of the rabbit in experimental chemotherapy of tuberculosis. Ann N Y Acad Sci. 1949 Dec 14;52(5):627-36, illust. doi: 10.1111/j.1749-6632.1949.tb53952.x. [DOI] [PubMed] [Google Scholar]
- Laliberte A., Gicquaud C. Polymerization of actin by positively charged liposomes. J Cell Biol. 1988 Apr;106(4):1221–1227. doi: 10.1083/jcb.106.4.1221. [DOI] [PMC free article] [PubMed] [Google Scholar]
- Mizushima-Sugano J., Maeda T., Miki-Noumura T. Flexural rigidity of singlet microtubules estimated from statistical analysis of their contour lengths and end-to-end distances. Biochim Biophys Acta. 1983 Jan 25;755(2):257–262. doi: 10.1016/0304-4165(83)90212-x. [DOI] [PubMed] [Google Scholar]
- Mukherjee T. M., Staehelin L. A. The fine-structural organization of the brush border of intestinal epithelial cells. J Cell Sci. 1971 May;8(3):573–599. doi: 10.1242/jcs.8.3.573. [DOI] [PubMed] [Google Scholar]
- OSTER G. Two-phase formation in solutions of tobacco mosaic virus and the problem of long-range forces. J Gen Physiol. 1950 May 20;33(5):445–473. doi: 10.1085/jgp.33.5.445. [DOI] [PMC free article] [PubMed] [Google Scholar]
- Rinnerthaler G., Herzog M., Klappacher M., Kunka H., Small J. V. Leading edge movement and ultrastructure in mouse macrophages. J Struct Biol. 1991 Feb;106(1):1–16. doi: 10.1016/1047-8477(91)90058-5. [DOI] [PubMed] [Google Scholar]
- Schroeder T. E. Actin in dividing cells: contractile ring filaments bind heavy meromyosin. Proc Natl Acad Sci U S A. 1973 Jun;70(6):1688–1692. doi: 10.1073/pnas.70.6.1688. [DOI] [PMC free article] [PubMed] [Google Scholar]
- Spudich J. A., Watt S. The regulation of rabbit skeletal muscle contraction. I. Biochemical studies of the interaction of the tropomyosin-troponin complex with actin and the proteolytic fragments of myosin. J Biol Chem. 1971 Aug 10;246(15):4866–4871. [PubMed] [Google Scholar]
- Suzuki A., Yamazaki M., Ito T. Osmoelastic coupling in biological structures: formation of parallel bundles of actin filaments in a crystalline-like structure caused by osmotic stress. Biochemistry. 1989 Jul 25;28(15):6513–6518. doi: 10.1021/bi00441a052. [DOI] [PubMed] [Google Scholar]
- Taylor D. L., Condeelis J. S. Cytoplasmic structure and contractility in amoeboid cells. Int Rev Cytol. 1979;56:57–144. doi: 10.1016/s0074-7696(08)61821-5. [DOI] [PubMed] [Google Scholar]
- Theriot J. A., Mitchison T. J. Actin microfilament dynamics in locomoting cells. Nature. 1991 Jul 11;352(6331):126–131. doi: 10.1038/352126a0. [DOI] [PubMed] [Google Scholar]
- Tilney L. G., Fukui Y., DeRosier D. J. Movement of the actin filament bundle in Mytilus sperm: a new mechanism is proposed. J Cell Biol. 1987 Apr;104(4):981–993. doi: 10.1083/jcb.104.4.981. [DOI] [PMC free article] [PubMed] [Google Scholar]
- Tilney L. G., Mooseker M. Actin in the brush-border of epithelial cells of the chicken intestine. Proc Natl Acad Sci U S A. 1971 Oct;68(10):2611–2615. doi: 10.1073/pnas.68.10.2611. [DOI] [PMC free article] [PubMed] [Google Scholar]
- Tobacman L. S., Korn E. D. The kinetics of actin nucleation and polymerization. J Biol Chem. 1983 Mar 10;258(5):3207–3214. [PubMed] [Google Scholar]
- Wieland T., de Vries J. X., Schäfer A., Faulstich H. Spectroscopic evidence for the interaction of phalloidin with actin. FEBS Lett. 1975 Jun 1;54(1):73–75. doi: 10.1016/0014-5793(75)81071-4. [DOI] [PubMed] [Google Scholar]