Abstract
We report here the presence of fast subthreshold oscillatory potentials recorded in vitro from neurons within layer 4 of the guinea pig frontal cortex. Two types of oscillatory neurons were recorded: (i) One type exhibited subthreshold oscillations whose frequency increased with membrane depolarization and encompassed a range of 10-45 Hz. Action potentials in this type of neuron demonstrated clear after-hyperpolarizations. (ii) The second type of neuron was characterized by narrow-frequency oscillations near 35-50 Hz. These oscillations often outlasted the initiating depolarizing stimulus. No calcium component could be identified in their action potential. In both types of cell the subthreshold oscillations were tetrodotoxin-sensitive, indicating that the depolarizing phase of the oscillation was generated by a voltage-dependent sodium conductance. The initial depolarizing phase was followed by a potassium conductance responsible for the falling phase of the oscillatory wave. In both types of cell, the subthreshold oscillation could trigger spikes at the oscillatory frequency, if the membrane was sufficiently depolarized. Combining intracellular recordings with Lucifer yellow staining showed that the narrow-frequency oscillatory activity was produced by a sparsely spinous interneuron located in layer 4 of the cortex. This neuron has extensive local axonal collaterals that ramify in layers 3 and 4 such that they may contribute to the columnar synchronization of activity in the 40- to 50-Hz range. Cortical activity in this frequency range has been proposed as the basis for the "conjunctive properties" of central nervous system networks.
Full text
PDF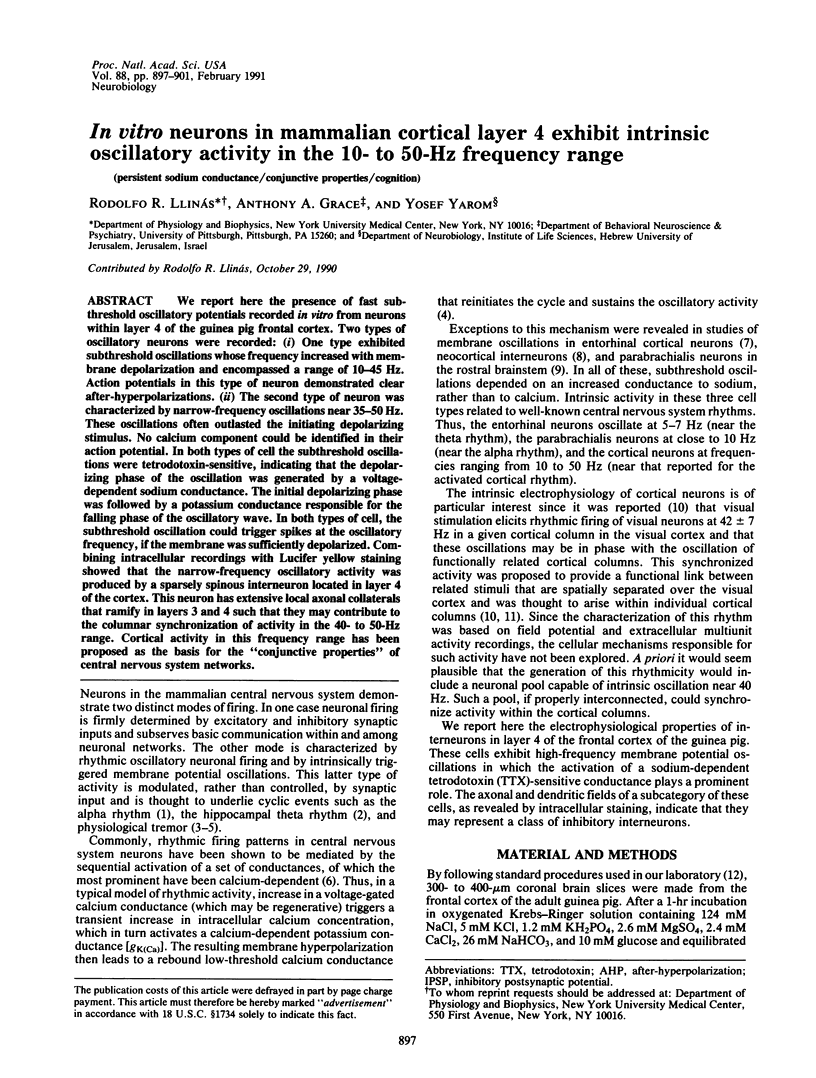
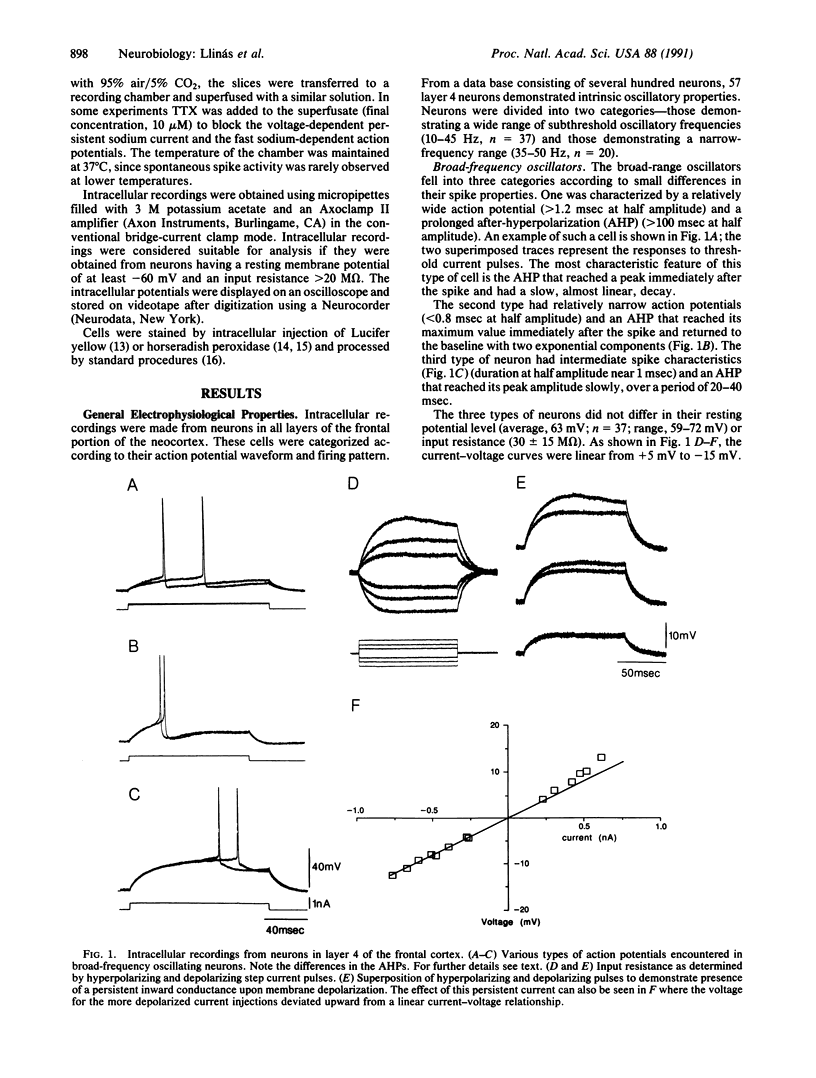
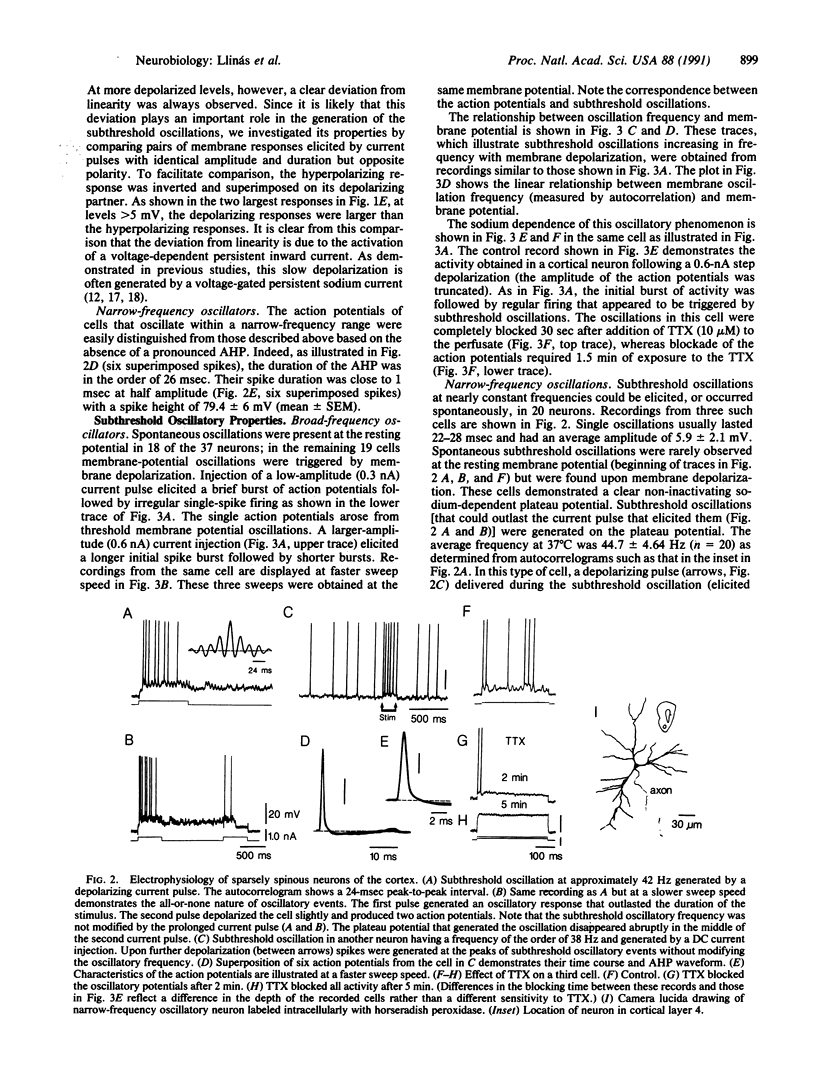
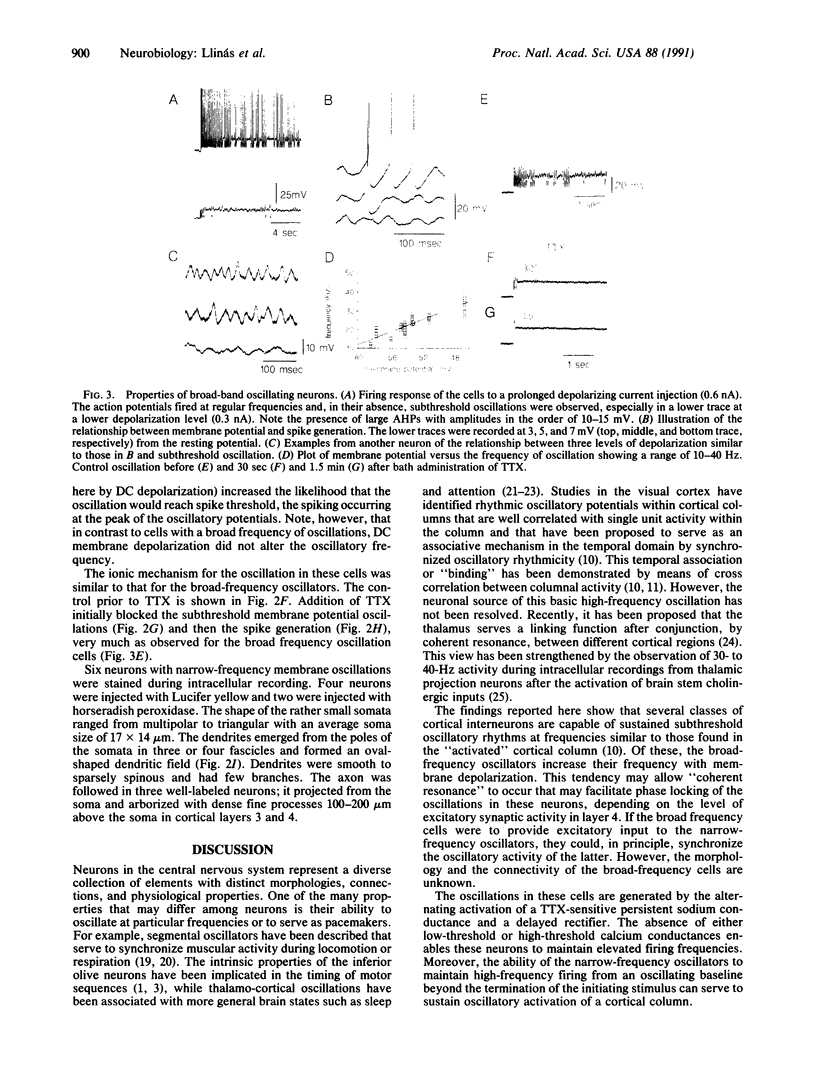
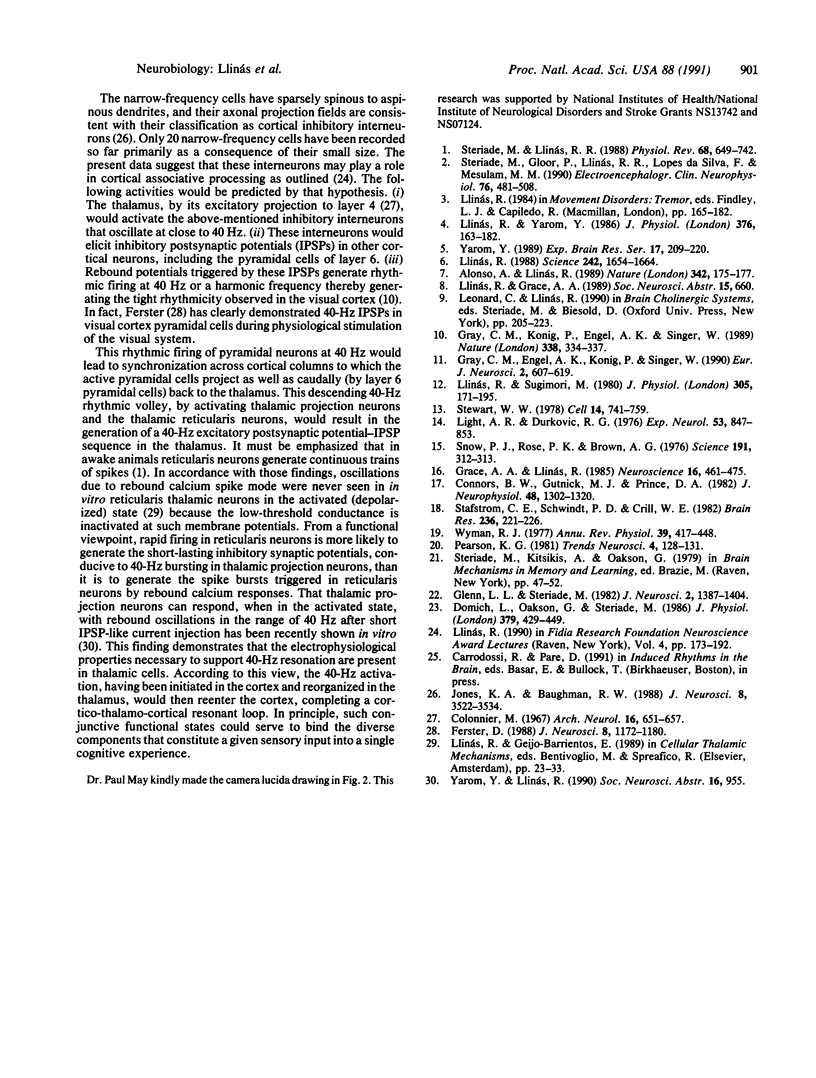
Images in this article
Selected References
These references are in PubMed. This may not be the complete list of references from this article.
- Alonso A., Llinás R. R. Subthreshold Na+-dependent theta-like rhythmicity in stellate cells of entorhinal cortex layer II. Nature. 1989 Nov 9;342(6246):175–177. doi: 10.1038/342175a0. [DOI] [PubMed] [Google Scholar]
- Connors B. W., Gutnick M. J., Prince D. A. Electrophysiological properties of neocortical neurons in vitro. J Neurophysiol. 1982 Dec;48(6):1302–1320. doi: 10.1152/jn.1982.48.6.1302. [DOI] [PubMed] [Google Scholar]
- Domich L., Oakson G., Steriade M. Thalamic burst patterns in the naturally sleeping cat: a comparison between cortically projecting and reticularis neurones. J Physiol. 1986 Oct;379:429–449. doi: 10.1113/jphysiol.1986.sp016262. [DOI] [PMC free article] [PubMed] [Google Scholar]
- Ferster D. Spatially opponent excitation and inhibition in simple cells of the cat visual cortex. J Neurosci. 1988 Apr;8(4):1172–1180. doi: 10.1523/JNEUROSCI.08-04-01172.1988. [DOI] [PMC free article] [PubMed] [Google Scholar]
- Glenn L. L., Steriade M. Discharge rate and excitability of cortically projecting intralaminar thalamic neurons during waking and sleep states. J Neurosci. 1982 Oct;2(10):1387–1404. doi: 10.1523/JNEUROSCI.02-10-01387.1982. [DOI] [PMC free article] [PubMed] [Google Scholar]
- Grace A. A., Llinás R. Morphological artifacts induced in intracellularly stained neurons by dehydration: circumvention using rapid dimethyl sulfoxide clearing. Neuroscience. 1985 Oct;16(2):461–475. doi: 10.1016/0306-4522(85)90018-1. [DOI] [PubMed] [Google Scholar]
- Gray C. M., König P., Engel A. K., Singer W. Oscillatory responses in cat visual cortex exhibit inter-columnar synchronization which reflects global stimulus properties. Nature. 1989 Mar 23;338(6213):334–337. doi: 10.1038/338334a0. [DOI] [PubMed] [Google Scholar]
- Gray Charles M., Engel Andreas K., König Peter, Singer Wolf. Stimulus-Dependent Neuronal Oscillations in Cat Visual Cortex: Receptive Field Properties and Feature Dependence. Eur J Neurosci. 1990;2(7):607–619. doi: 10.1111/j.1460-9568.1990.tb00450.x. [DOI] [PubMed] [Google Scholar]
- Jones K. A., Baughman R. W. NMDA- and non-NMDA-receptor components of excitatory synaptic potentials recorded from cells in layer V of rat visual cortex. J Neurosci. 1988 Sep;8(9):3522–3534. doi: 10.1523/JNEUROSCI.08-09-03522.1988. [DOI] [PMC free article] [PubMed] [Google Scholar]
- Light A. R., Durkovic R. G. Horseradish peroxidase: an improvement in intracellular staining of single, electrophysiologically characterized neurons. Exp Neurol. 1976 Dec;53(3):847–853. doi: 10.1016/0014-4886(76)90159-x. [DOI] [PubMed] [Google Scholar]
- Llinás R. R. The intrinsic electrophysiological properties of mammalian neurons: insights into central nervous system function. Science. 1988 Dec 23;242(4886):1654–1664. doi: 10.1126/science.3059497. [DOI] [PubMed] [Google Scholar]
- Llinás R., Sugimori M. Electrophysiological properties of in vitro Purkinje cell somata in mammalian cerebellar slices. J Physiol. 1980 Aug;305:171–195. doi: 10.1113/jphysiol.1980.sp013357. [DOI] [PMC free article] [PubMed] [Google Scholar]
- Llinás R., Yarom Y. Oscillatory properties of guinea-pig inferior olivary neurones and their pharmacological modulation: an in vitro study. J Physiol. 1986 Jul;376:163–182. doi: 10.1113/jphysiol.1986.sp016147. [DOI] [PMC free article] [PubMed] [Google Scholar]
- Snow P. J., Rose P. K., Brown A. G. Tracing axons and axon collaterals of spinal neurons using intracellular injection of horseradish peroxidase. Science. 1976 Jan 23;191(4224):312–313. doi: 10.1126/science.54936. [DOI] [PubMed] [Google Scholar]
- Stafstrom C. E., Schwindt P. C., Crill W. E. Negative slope conductance due to a persistent subthreshold sodium current in cat neocortical neurons in vitro. Brain Res. 1982 Mar 18;236(1):221–226. doi: 10.1016/0006-8993(82)90050-6. [DOI] [PubMed] [Google Scholar]
- Steriade M., Gloor P., Llinás R. R., Lopes de Silva F. H., Mesulam M. M. Report of IFCN Committee on Basic Mechanisms. Basic mechanisms of cerebral rhythmic activities. Electroencephalogr Clin Neurophysiol. 1990 Dec;76(6):481–508. doi: 10.1016/0013-4694(90)90001-z. [DOI] [PubMed] [Google Scholar]
- Steriade M., Llinás R. R. The functional states of the thalamus and the associated neuronal interplay. Physiol Rev. 1988 Jul;68(3):649–742. doi: 10.1152/physrev.1988.68.3.649. [DOI] [PubMed] [Google Scholar]
- Stewart W. W. Functional connections between cells as revealed by dye-coupling with a highly fluorescent naphthalimide tracer. Cell. 1978 Jul;14(3):741–759. doi: 10.1016/0092-8674(78)90256-8. [DOI] [PubMed] [Google Scholar]
- Wyman R. J. Neural generation of the breathing rhythm. Annu Rev Physiol. 1977;39:417–448. doi: 10.1146/annurev.ph.39.030177.002221. [DOI] [PubMed] [Google Scholar]