Abstract
Human skeletal muscle is composed of various muscle fiber types. We hypothesized that differences in metabolism between fiber types could be detected noninvasively with 31P nuclear magnetic resonance spectroscopy during maximal exercise. This assumes that during maximal exercise all fiber types are recruited and all vary in the amount of acidosis. The calf muscles of seven subjects were studied. Two different coils were applied: an 11-cm-diameter surface coil and a five-segment meander coil. The meander coil was used to localize the 31P signal to either the medial or the lateral gastrocnemius. Maximal exercise, consisting of rapid plantar flexions, resulted in an 83.7% +/- 7.8% decrease of the phosphocreatine pool and an 8-fold increase of the inorganic phosphate (Pi) pool. At rest the Pi pool was observed as a single resonance (pH 7.0). Toward the end of the first minute of exercise, three subjects showed three distinct Pi peaks. During the second minute of exercise the pH values stabilized at 7.12 +/- 0.12, 6.63 +/- 0.15, and 6.27 +/- 0.23. The same pattern was seen when the signal was collected from the medial or lateral gastrocnemius. In four subjects only two distinct Pi peaks were observed. The Pi peaks had differing relative areas in different subjects, but they were reproducible in each individual. This method allowed us to study the appearance and disappearance of the different Pi peaks, together with the changes in pH. Because multiple Pi peaks were seen in single muscles they most likely identify different muscle fiber types.
Full text
PDF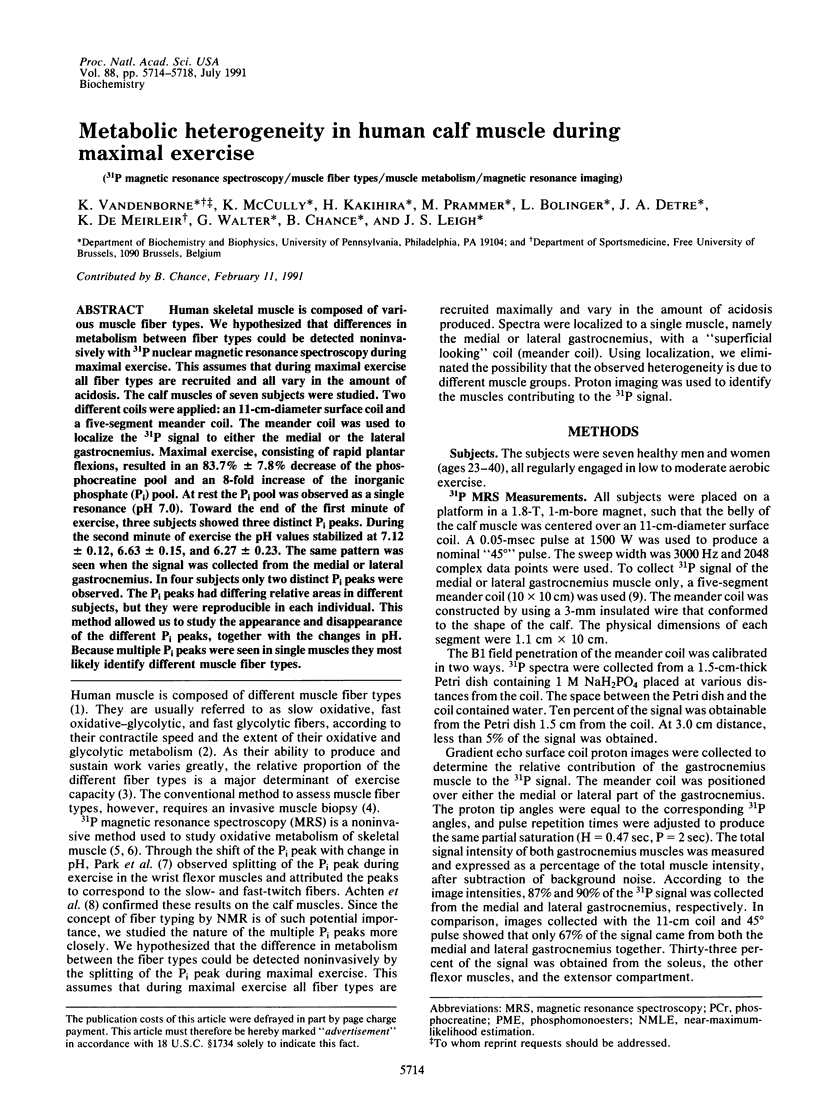
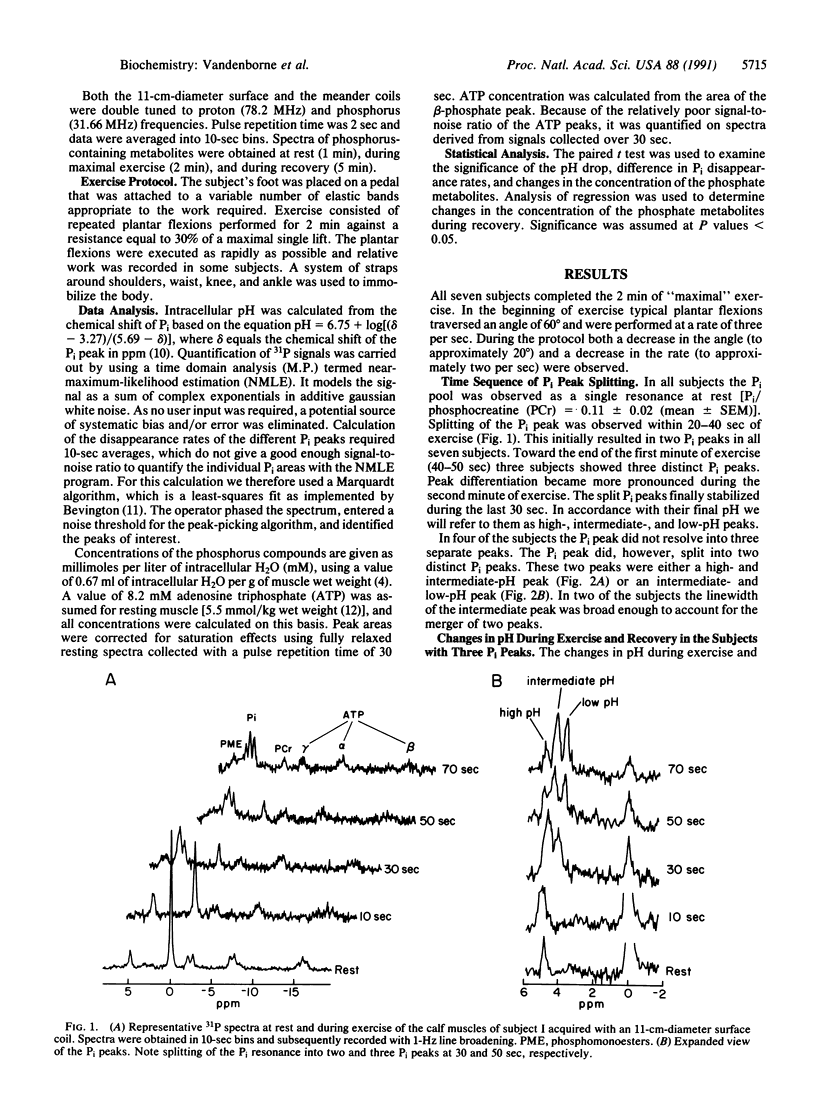
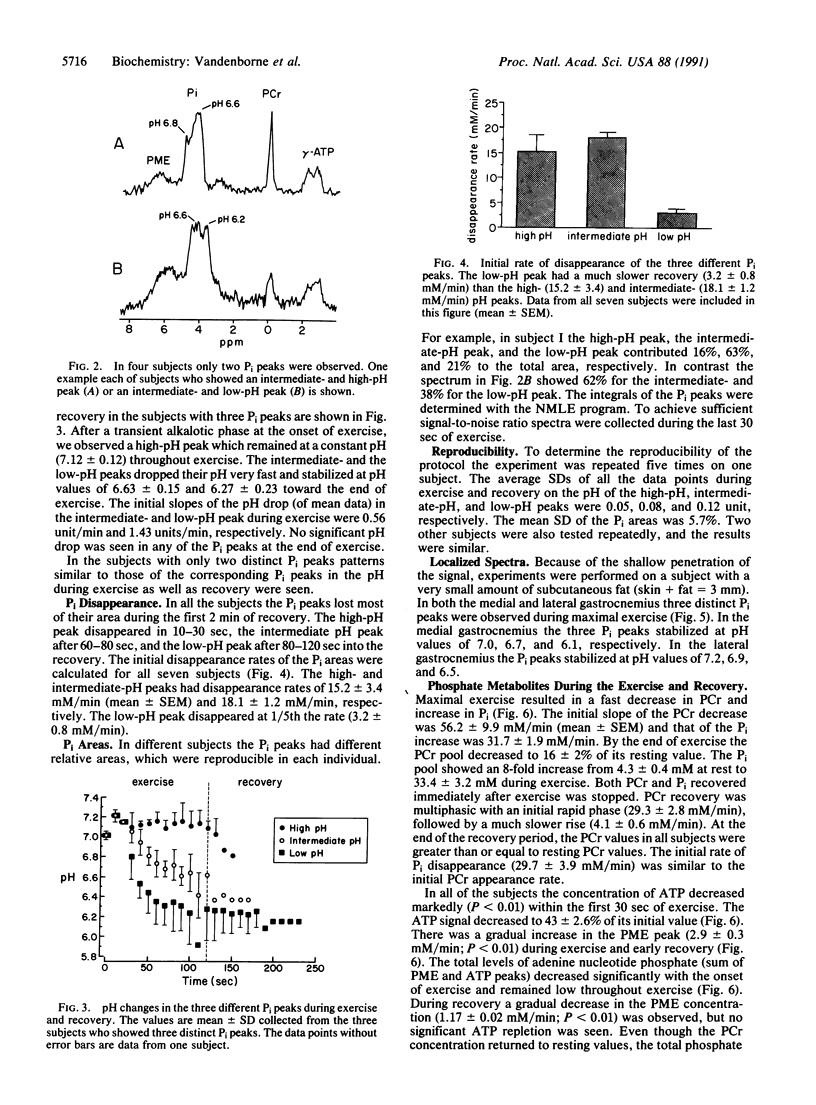
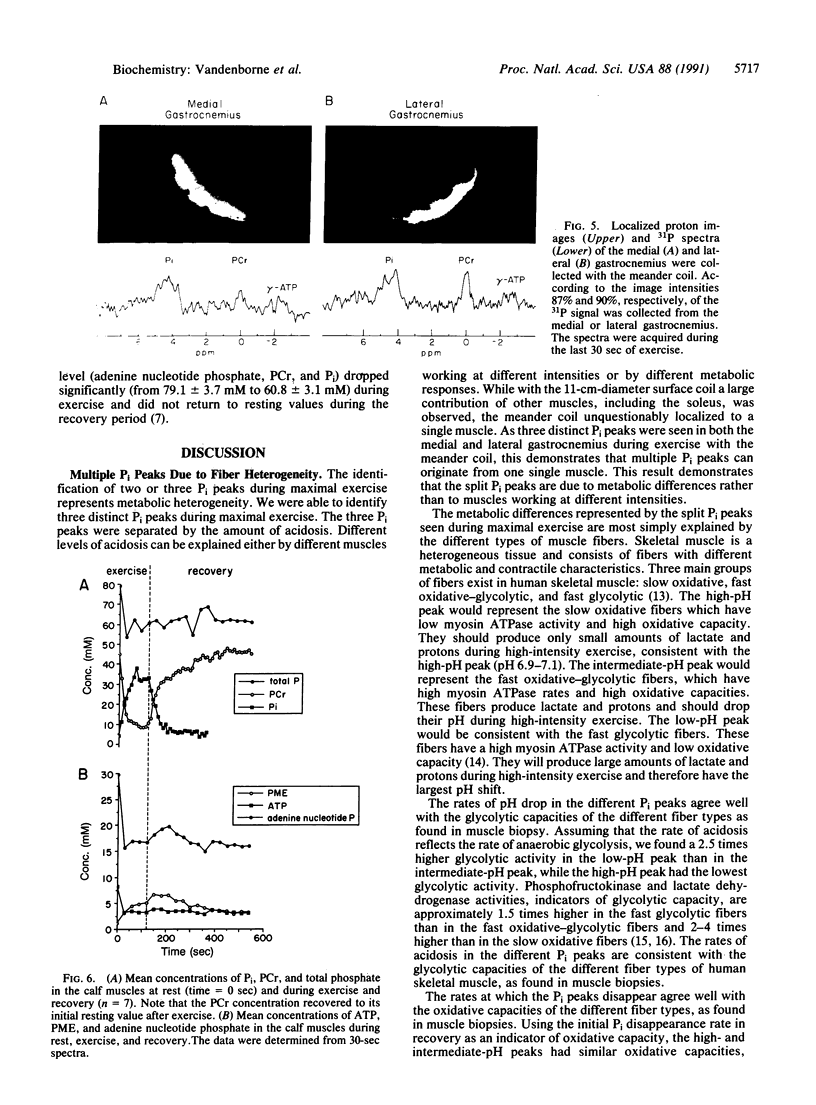
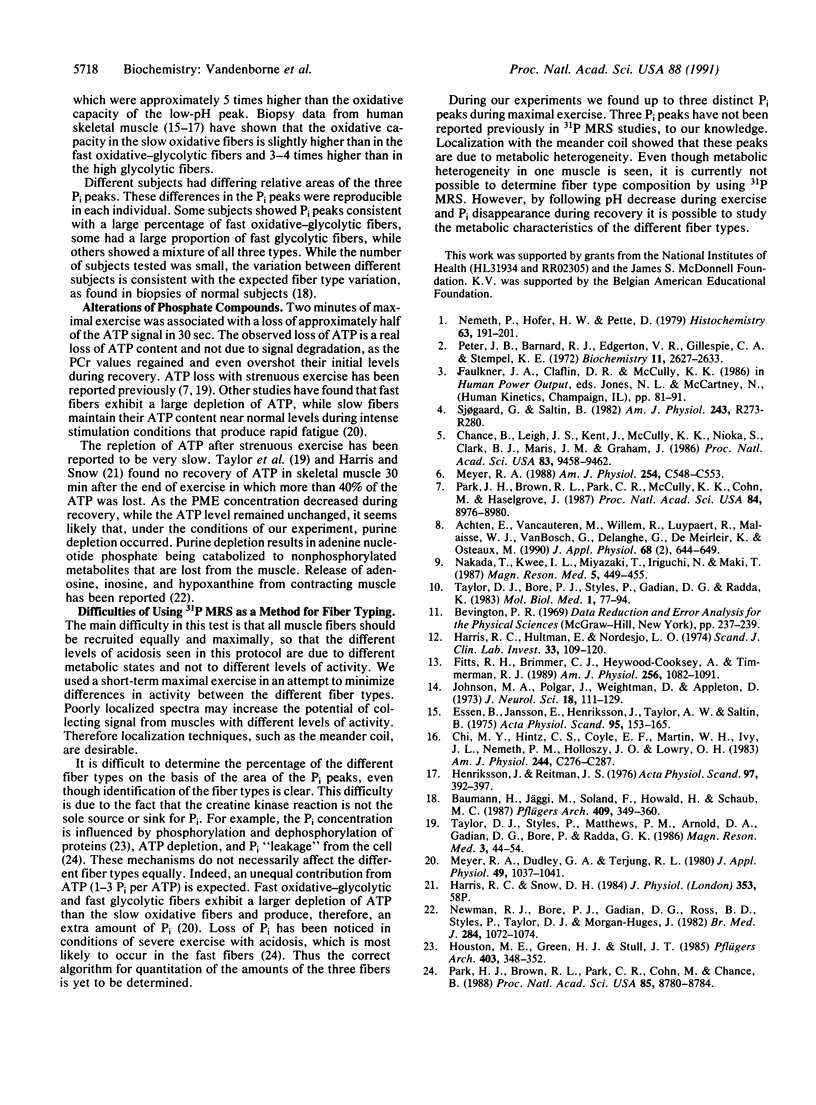
Images in this article
Selected References
These references are in PubMed. This may not be the complete list of references from this article.
- Achten E., Van Cauteren M., Willem R., Luypaert R., Malaisse W. J., Van Bosch G., Delanghe G., De Meirleir K., Osteaux M. 31P-NMR spectroscopy and the metabolic properties of different muscle fibers. J Appl Physiol (1985) 1990 Feb;68(2):644–649. doi: 10.1152/jappl.1990.68.2.644. [DOI] [PubMed] [Google Scholar]
- Baumann H., Jäggi M., Soland F., Howald H., Schaub M. C. Exercise training induces transitions of myosin isoform subunits within histochemically typed human muscle fibres. Pflugers Arch. 1987 Aug;409(4-5):349–360. doi: 10.1007/BF00583788. [DOI] [PubMed] [Google Scholar]
- Chance B., Leigh J. S., Jr, Kent J., McCully K., Nioka S., Clark B. J., Maris J. M., Graham T. Multiple controls of oxidative metabolism in living tissues as studied by phosphorus magnetic resonance. Proc Natl Acad Sci U S A. 1986 Dec;83(24):9458–9462. doi: 10.1073/pnas.83.24.9458. [DOI] [PMC free article] [PubMed] [Google Scholar]
- Chi M. M., Hintz C. S., Coyle E. F., Martin W. H., 3rd, Ivy J. L., Nemeth P. M., Holloszy J. O., Lowry O. H. Effects of detraining on enzymes of energy metabolism in individual human muscle fibers. Am J Physiol. 1983 Mar;244(3):C276–C287. doi: 10.1152/ajpcell.1983.244.3.C276. [DOI] [PubMed] [Google Scholar]
- Essén B., Jansson E., Henriksson J., Taylor A. W., Saltin B. Metabolic characteristics of fibre types in human skeletal muscle. Acta Physiol Scand. 1975 Oct;95(2):153–165. doi: 10.1111/j.1748-1716.1975.tb10038.x. [DOI] [PubMed] [Google Scholar]
- Harris R. C., Hultman E., Nordesjö L. O. Glycogen, glycolytic intermediates and high-energy phosphates determined in biopsy samples of musculus quadriceps femoris of man at rest. Methods and variance of values. Scand J Clin Lab Invest. 1974 Apr;33(2):109–120. [PubMed] [Google Scholar]
- Henriksson J., Reitman J. S. Quantitative measures of enzyme activities in type I and type II muscle fibres of man after training. Acta Physiol Scand. 1976 Jul;97(3):392–397. doi: 10.1111/j.1748-1716.1976.tb10279.x. [DOI] [PubMed] [Google Scholar]
- Houston M. E., Green H. J., Stull J. T. Myosin light chain phosphorylation and isometric twitch potentiation in intact human muscle. Pflugers Arch. 1985 Apr;403(4):348–352. doi: 10.1007/BF00589245. [DOI] [PubMed] [Google Scholar]
- Johnson M. A., Polgar J., Weightman D., Appleton D. Data on the distribution of fibre types in thirty-six human muscles. An autopsy study. J Neurol Sci. 1973 Jan;18(1):111–129. doi: 10.1016/0022-510x(73)90023-3. [DOI] [PubMed] [Google Scholar]
- Meyer R. A. A linear model of muscle respiration explains monoexponential phosphocreatine changes. Am J Physiol. 1988 Apr;254(4 Pt 1):C548–C553. doi: 10.1152/ajpcell.1988.254.4.C548. [DOI] [PubMed] [Google Scholar]
- Meyer R. A., Dudley G. A., Terjung R. L. Ammonia and IMP in different skeletal muscle fibers after exercise in rats. J Appl Physiol Respir Environ Exerc Physiol. 1980 Dec;49(6):1037–1041. doi: 10.1152/jappl.1980.49.6.1037. [DOI] [PubMed] [Google Scholar]
- Nakada T., Kwee I. L., Miyazaki T., Iriguchi N., Maki T. 31P NMR spectroscopy of the stomach by zig-zag coil. Magn Reson Med. 1987 Nov;5(5):449–455. doi: 10.1002/mrm.1910050506. [DOI] [PubMed] [Google Scholar]
- Nemeth P., Hofer H. W., Pette D. Metabolic heterogeneity of muscle fibers classified by myosin ATPase. Histochemistry. 1979 Sep;63(2):191–201. doi: 10.1007/BF00644541. [DOI] [PubMed] [Google Scholar]
- Newman R. J., Bore P. J., Chan L., Gadian D. G., Styles P., Taylor D., Radda G. K. Nuclear magnetic resonance studies of forearm muscle in Duchenne dystrophy. Br Med J (Clin Res Ed) 1982 Apr 10;284(6322):1072–1074. doi: 10.1136/bmj.284.6322.1072. [DOI] [PMC free article] [PubMed] [Google Scholar]
- Park J. H., Brown R. L., Park C. R., Cohn M., Chance B. Energy metabolism of the untrained muscle of elite runners as observed by 31P magnetic resonance spectroscopy: evidence suggesting a genetic endowment for endurance exercise. Proc Natl Acad Sci U S A. 1988 Dec;85(23):8780–8784. doi: 10.1073/pnas.85.23.8780. [DOI] [PMC free article] [PubMed] [Google Scholar]
- Park J. H., Brown R. L., Park C. R., McCully K., Cohn M., Haselgrove J., Chance B. Functional pools of oxidative and glycolytic fibers in human muscle observed by 31P magnetic resonance spectroscopy during exercise. Proc Natl Acad Sci U S A. 1987 Dec;84(24):8976–8980. doi: 10.1073/pnas.84.24.8976. [DOI] [PMC free article] [PubMed] [Google Scholar]
- Peter J. B., Barnard R. J., Edgerton V. R., Gillespie C. A., Stempel K. E. Metabolic profiles of three fiber types of skeletal muscle in guinea pigs and rabbits. Biochemistry. 1972 Jul 4;11(14):2627–2633. doi: 10.1021/bi00764a013. [DOI] [PubMed] [Google Scholar]
- Taylor D. J., Bore P. J., Styles P., Gadian D. G., Radda G. K. Bioenergetics of intact human muscle. A 31P nuclear magnetic resonance study. Mol Biol Med. 1983 Jul;1(1):77–94. [PubMed] [Google Scholar]
- Taylor D. J., Styles P., Matthews P. M., Arnold D. A., Gadian D. G., Bore P., Radda G. K. Energetics of human muscle: exercise-induced ATP depletion. Magn Reson Med. 1986 Feb;3(1):44–54. doi: 10.1002/mrm.1910030107. [DOI] [PubMed] [Google Scholar]