Abstract
The physical mapping of complex genomes is based on the construction of a genomic library and the determination of the overlaps between the inserts of the mapping clones in order to generate an ordered, cloned representation of nearly all the sequences present in the target genome. Evaluation of the relative efficiency of experimental procedures used to accomplish this goal must minimally include a comparison of the fraction of the genome covered by the ordered arrays (or "contigs"), the average size of the contigs, and the cost, in terms of time and resources, required to generate the map. Sequence-tagged-site (STS) content mapping is one strategy that has been proposed and is being utilized for this type of experiment. This paper describes three STS selection schemes and presents computer simulations of contig-building experiments based on these procedures. The results of these simulations suggest that a nonrandom STS strategy that uses paired probes requires one-third to one-fourth as many STS assays as are required in random and nonpaired approaches, and also results in a map that has both greater genome coverage and a larger average contig size. This strategy promises to reduce the time and cost required to build a high-quality physical map.
Full text
PDF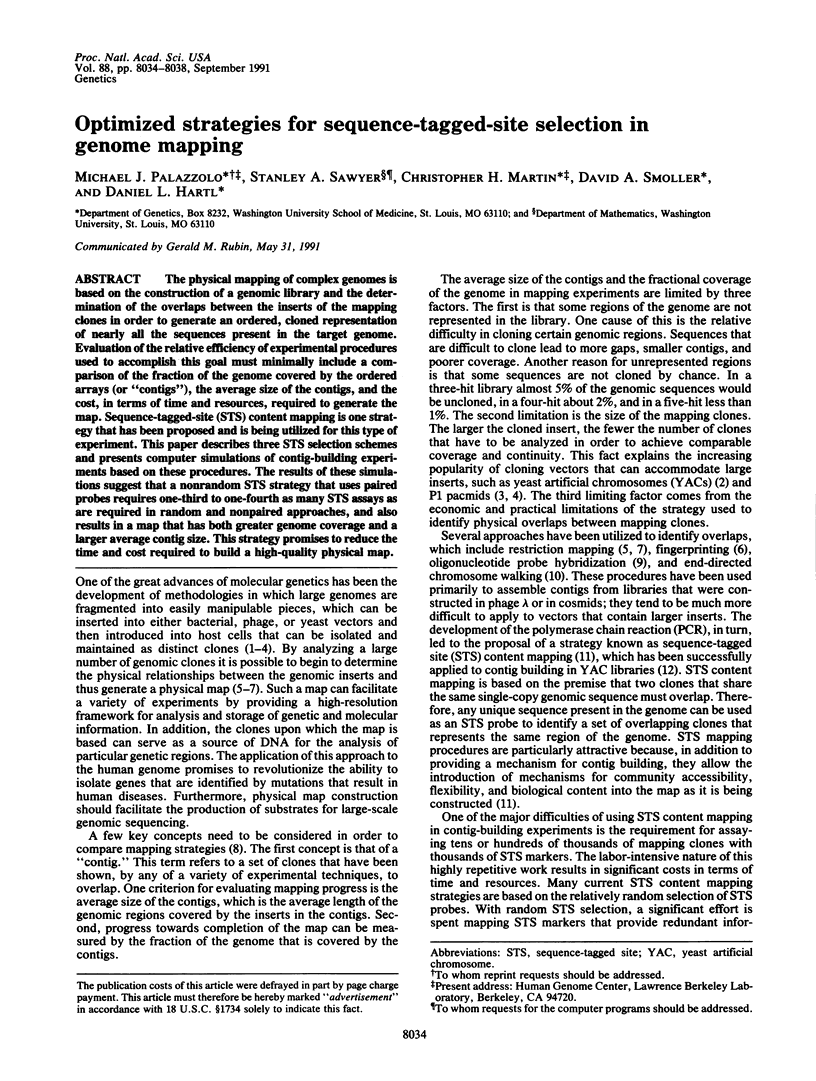
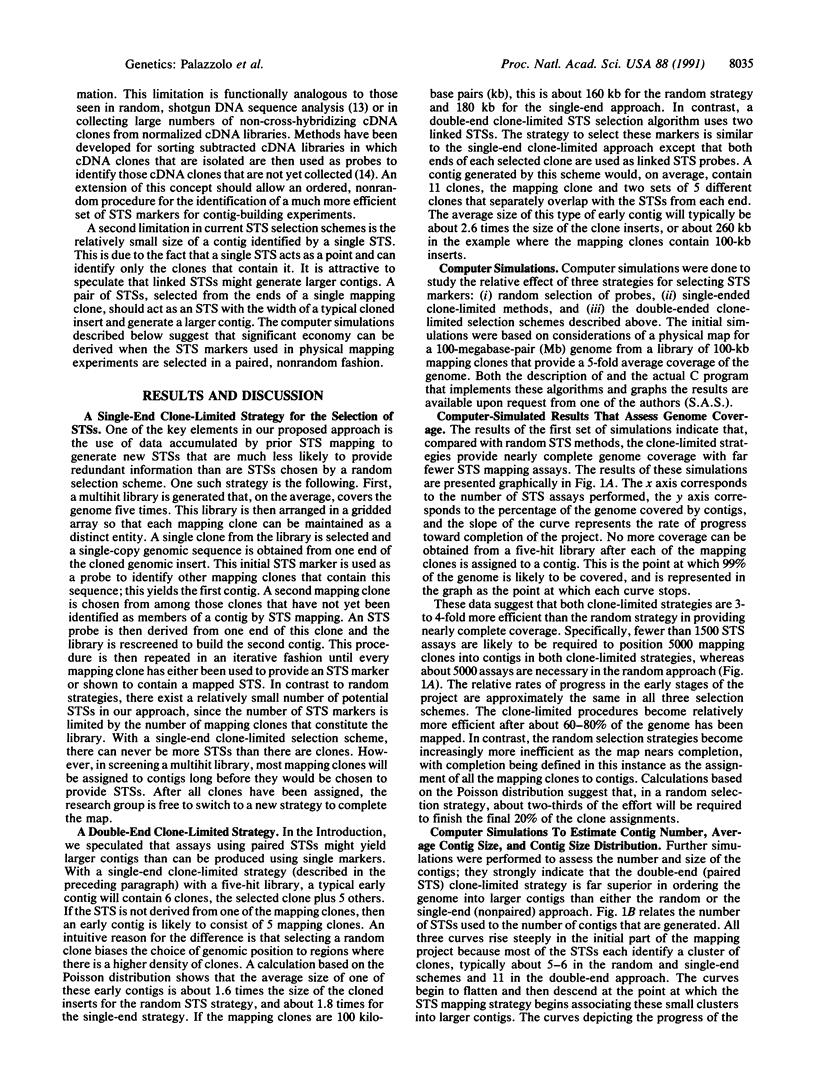
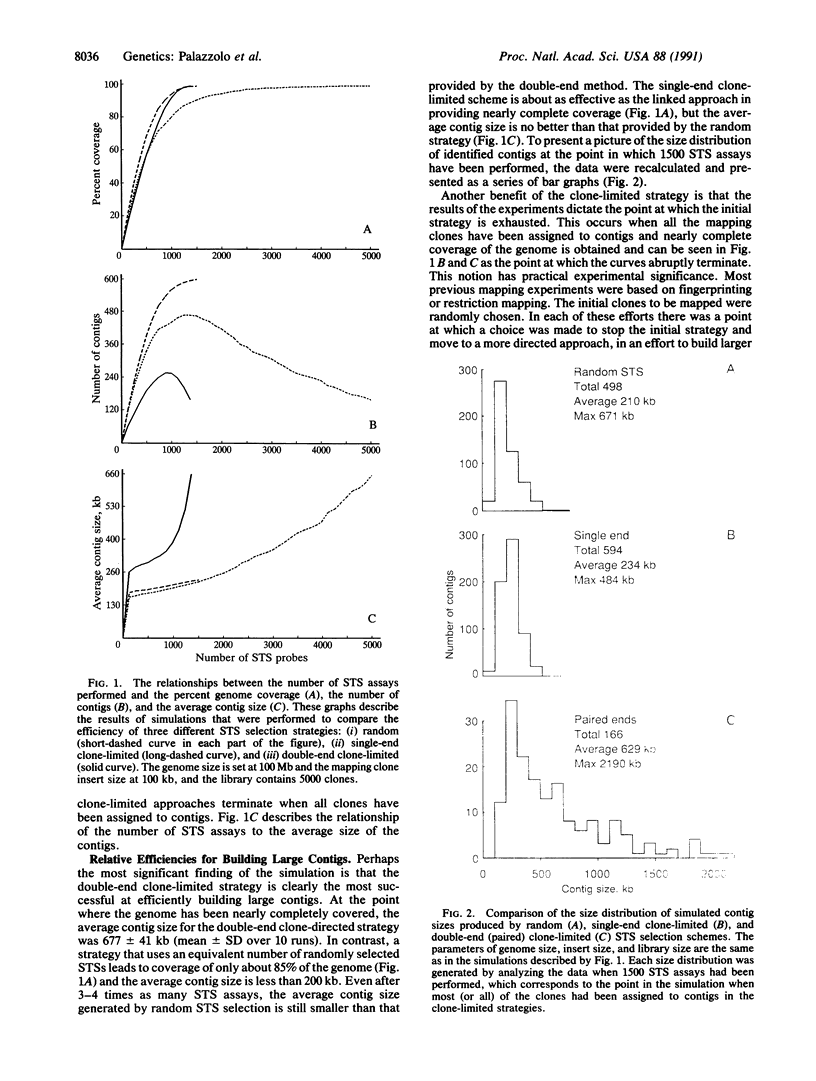
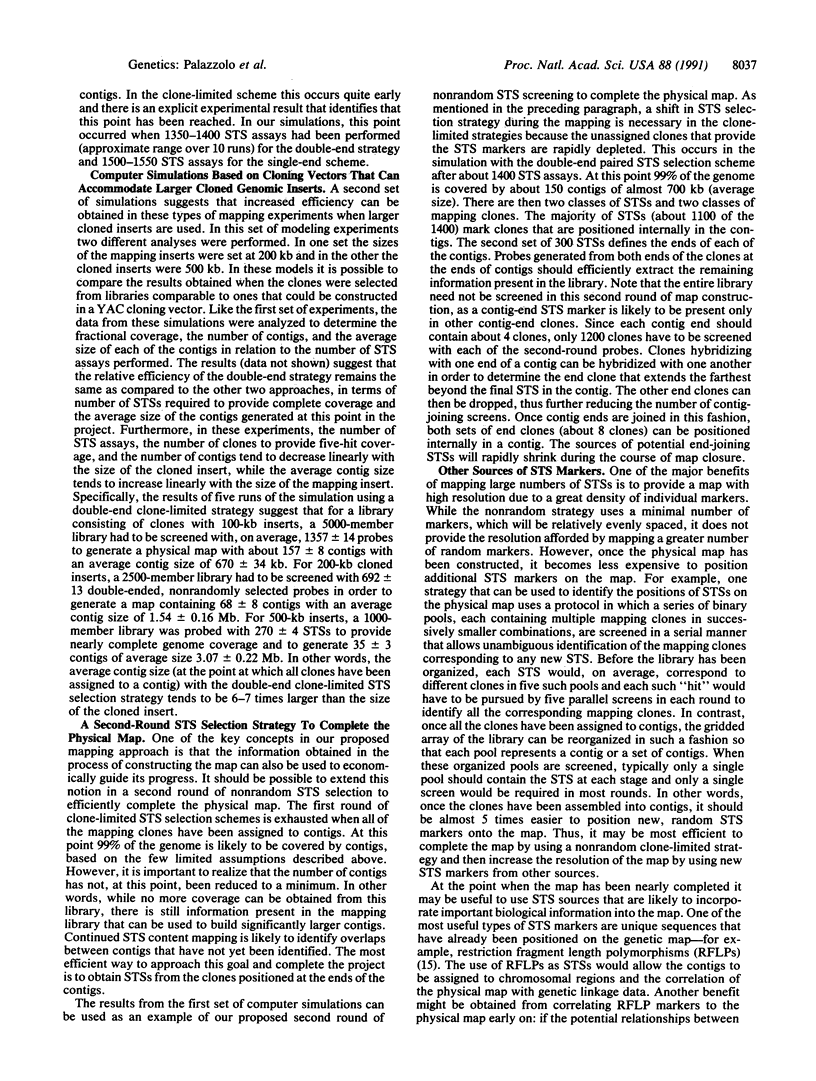
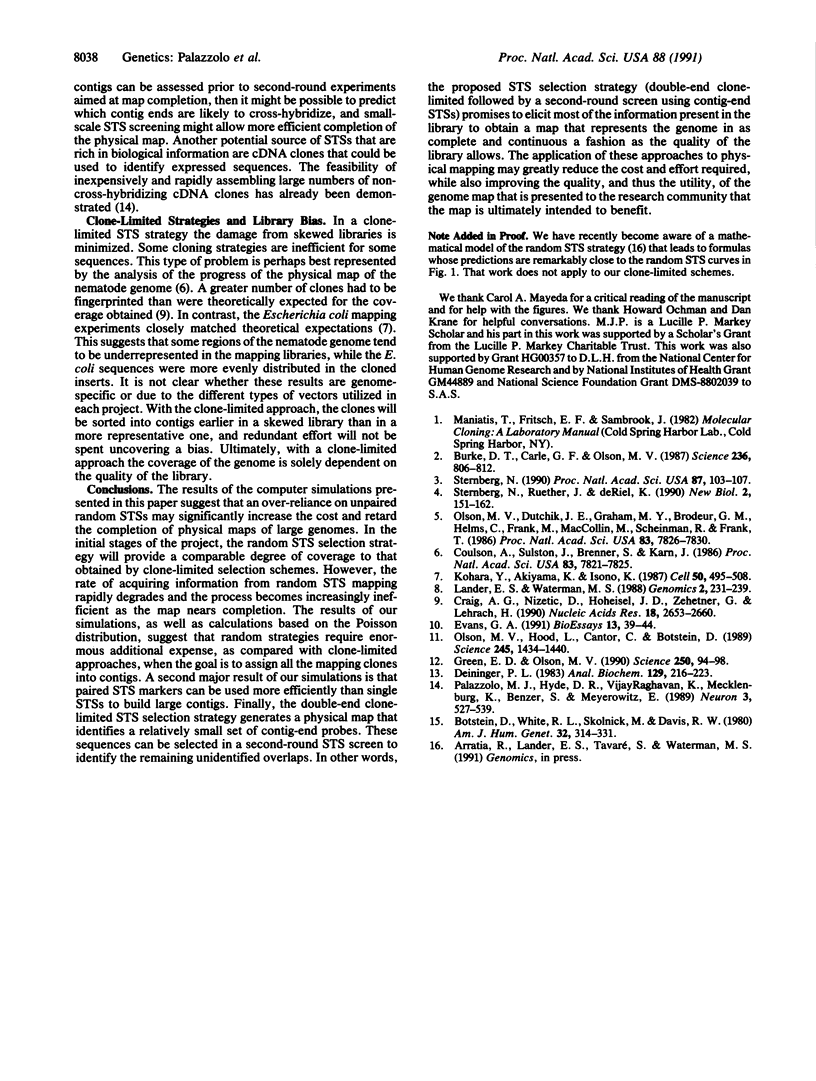
Images in this article
Selected References
These references are in PubMed. This may not be the complete list of references from this article.
- Botstein D., White R. L., Skolnick M., Davis R. W. Construction of a genetic linkage map in man using restriction fragment length polymorphisms. Am J Hum Genet. 1980 May;32(3):314–331. [PMC free article] [PubMed] [Google Scholar]
- Burke D. T., Carle G. F., Olson M. V. Cloning of large segments of exogenous DNA into yeast by means of artificial chromosome vectors. Science. 1987 May 15;236(4803):806–812. doi: 10.1126/science.3033825. [DOI] [PubMed] [Google Scholar]
- Coulson A., Sulston J., Brenner S., Karn J. Toward a physical map of the genome of the nematode Caenorhabditis elegans. Proc Natl Acad Sci U S A. 1986 Oct;83(20):7821–7825. doi: 10.1073/pnas.83.20.7821. [DOI] [PMC free article] [PubMed] [Google Scholar]
- Craig A. G., Nizetic D., Hoheisel J. D., Zehetner G., Lehrach H. Ordering of cosmid clones covering the herpes simplex virus type I (HSV-I) genome: a test case for fingerprinting by hybridisation. Nucleic Acids Res. 1990 May 11;18(9):2653–2660. doi: 10.1093/nar/18.9.2653. [DOI] [PMC free article] [PubMed] [Google Scholar]
- Deininger P. L. Random subcloning of sonicated DNA: application to shotgun DNA sequence analysis. Anal Biochem. 1983 Feb 15;129(1):216–223. doi: 10.1016/0003-2697(83)90072-6. [DOI] [PubMed] [Google Scholar]
- Evans G. A. Combinatoric strategies for genome mapping. Bioessays. 1991 Jan;13(1):39–44. doi: 10.1002/bies.950130108. [DOI] [PubMed] [Google Scholar]
- Green E. D., Olson M. V. Chromosomal region of the cystic fibrosis gene in yeast artificial chromosomes: a model for human genome mapping. Science. 1990 Oct 5;250(4977):94–98. doi: 10.1126/science.2218515. [DOI] [PubMed] [Google Scholar]
- Kohara Y., Akiyama K., Isono K. The physical map of the whole E. coli chromosome: application of a new strategy for rapid analysis and sorting of a large genomic library. Cell. 1987 Jul 31;50(3):495–508. doi: 10.1016/0092-8674(87)90503-4. [DOI] [PubMed] [Google Scholar]
- Lander E. S., Waterman M. S. Genomic mapping by fingerprinting random clones: a mathematical analysis. Genomics. 1988 Apr;2(3):231–239. doi: 10.1016/0888-7543(88)90007-9. [DOI] [PubMed] [Google Scholar]
- Olson M. V., Dutchik J. E., Graham M. Y., Brodeur G. M., Helms C., Frank M., MacCollin M., Scheinman R., Frank T. Random-clone strategy for genomic restriction mapping in yeast. Proc Natl Acad Sci U S A. 1986 Oct;83(20):7826–7830. doi: 10.1073/pnas.83.20.7826. [DOI] [PMC free article] [PubMed] [Google Scholar]
- Olson M., Hood L., Cantor C., Botstein D. A common language for physical mapping of the human genome. Science. 1989 Sep 29;245(4925):1434–1435. doi: 10.1126/science.2781285. [DOI] [PubMed] [Google Scholar]
- Palazzolo M. J., Hyde D. R., VijayRaghavan K., Mecklenburg K., Benzer S., Meyerowitz E. Use of a new strategy to isolate and characterize 436 Drosophila cDNA clones corresponding to RNAs detected in adult heads but not in early embryos. Neuron. 1989 Oct;3(4):527–539. doi: 10.1016/0896-6273(89)90211-0. [DOI] [PubMed] [Google Scholar]
- Sternberg N. Bacteriophage P1 cloning system for the isolation, amplification, and recovery of DNA fragments as large as 100 kilobase pairs. Proc Natl Acad Sci U S A. 1990 Jan;87(1):103–107. doi: 10.1073/pnas.87.1.103. [DOI] [PMC free article] [PubMed] [Google Scholar]
- Sternberg N., Ruether J., deRiel K. Generation of a 50,000-member human DNA library with an average DNA insert size of 75-100 kbp in a bacteriophage P1 cloning vector. New Biol. 1990 Feb;2(2):151–162. [PubMed] [Google Scholar]