Abstract
We consider the question of how to design proteins. How can we find "good" amino acid sequences (i) that fold to a desired "target" structure as a native conformation of lowest accessible free energy and (ii) that will not simultaneously fold to many other conformations of the same free energy? Current protein designs often focus on helix propensities and turns. We focus here on designing the hydrophobicity. For a model of self-avoiding hydrophobic/polar chains on two-dimensional square lattices, geometric proofs and exhaustive enumerations show the following results. (i) The strategy hydrophobic residues inside/polar residues outside is not optimal. Placement of additional hydrophobic residues on the surface is often necessary. (ii) To avoid unwanted conformations, the designed sequence must have neither too many nor too few hydrophobic residues. (iii) The computational complexity of inverse folding appears to be in a different class than folding: unlike the folding problem, the design problem does not scale exponentially with chain length. Some design strategies, described here for the lattice model, produce good sequences and scale only linearly with chain length.
Full text
PDF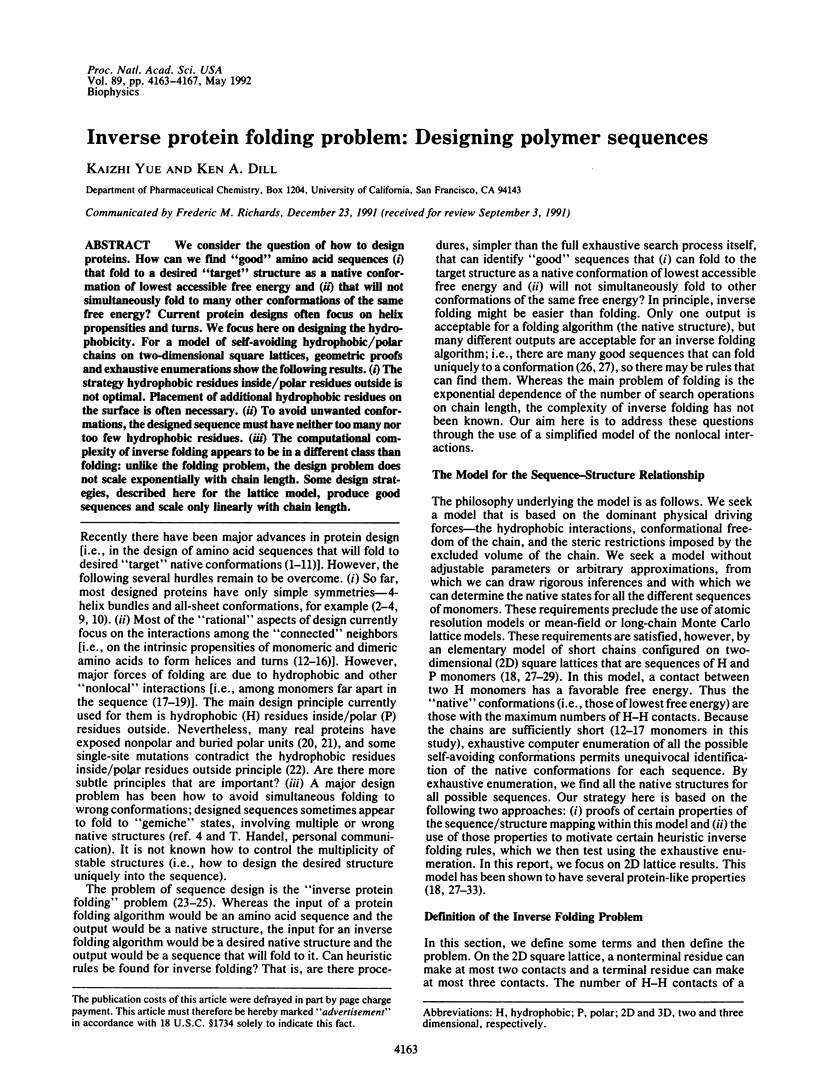
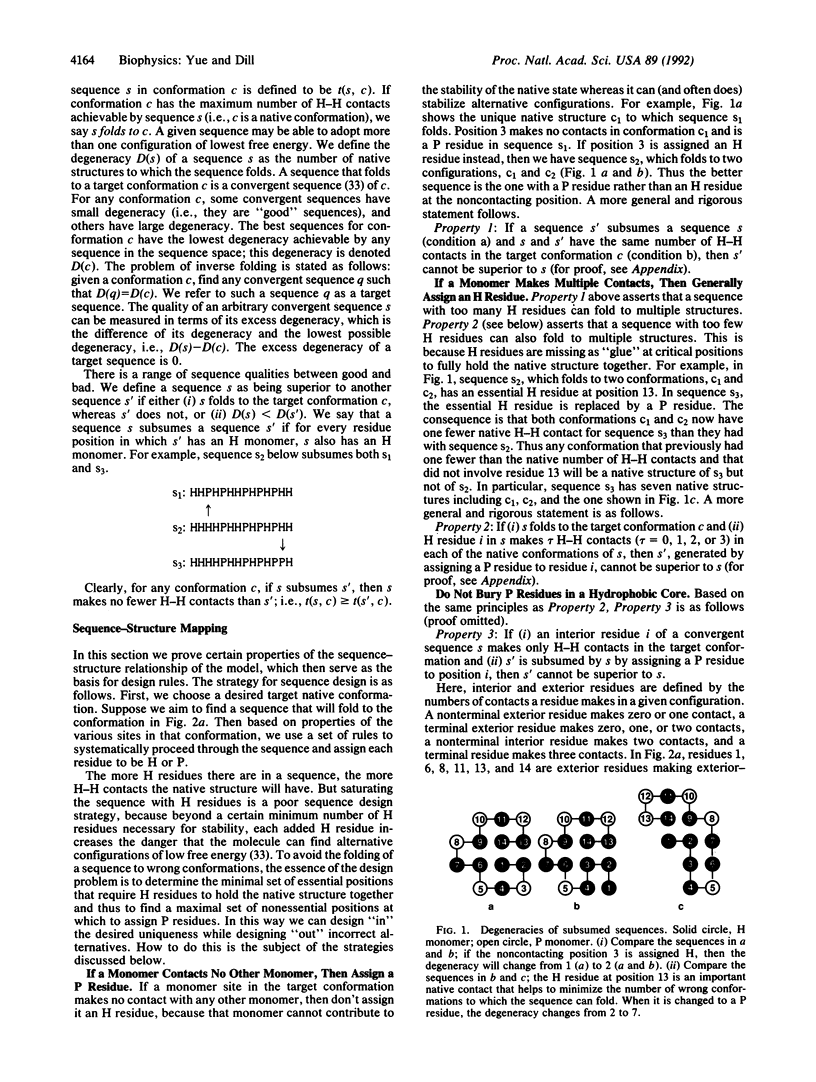
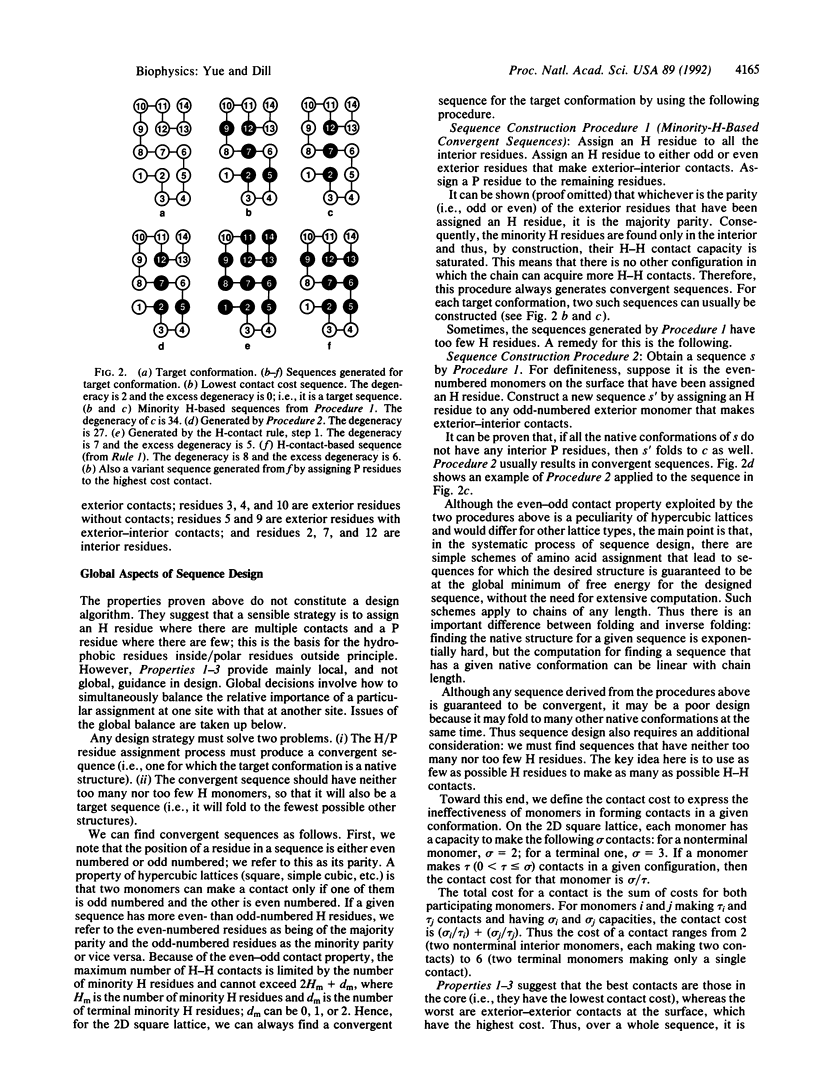
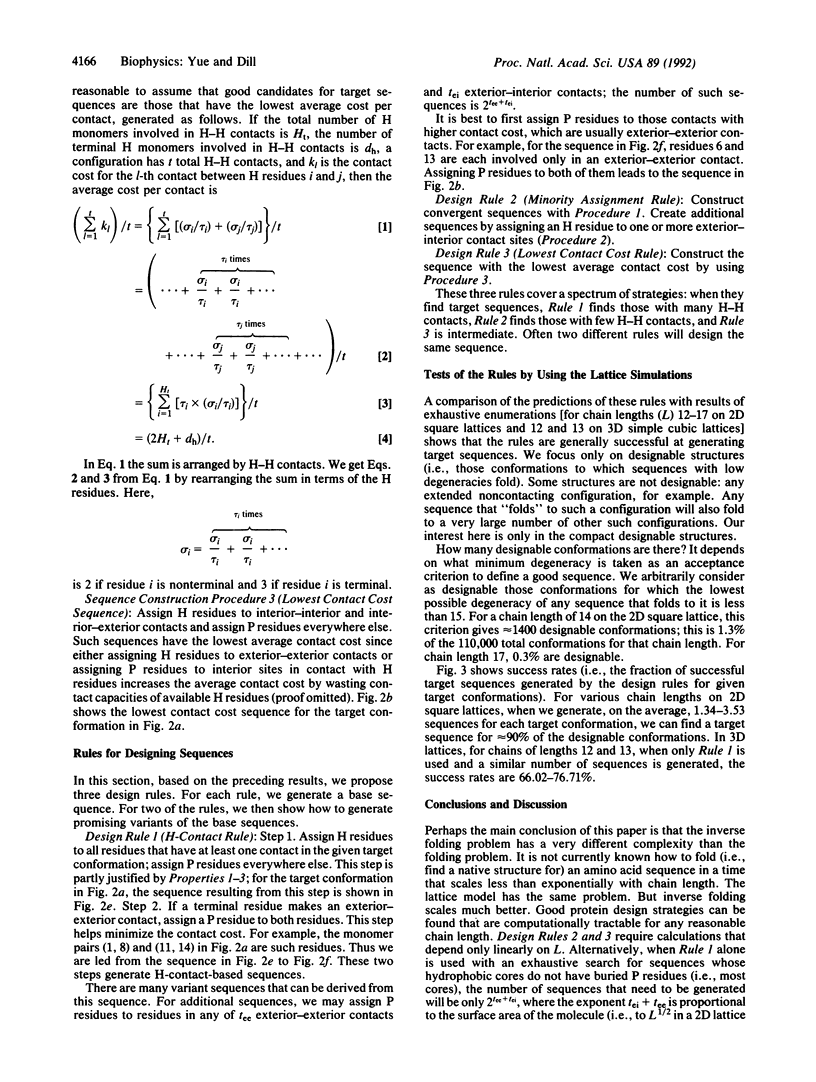
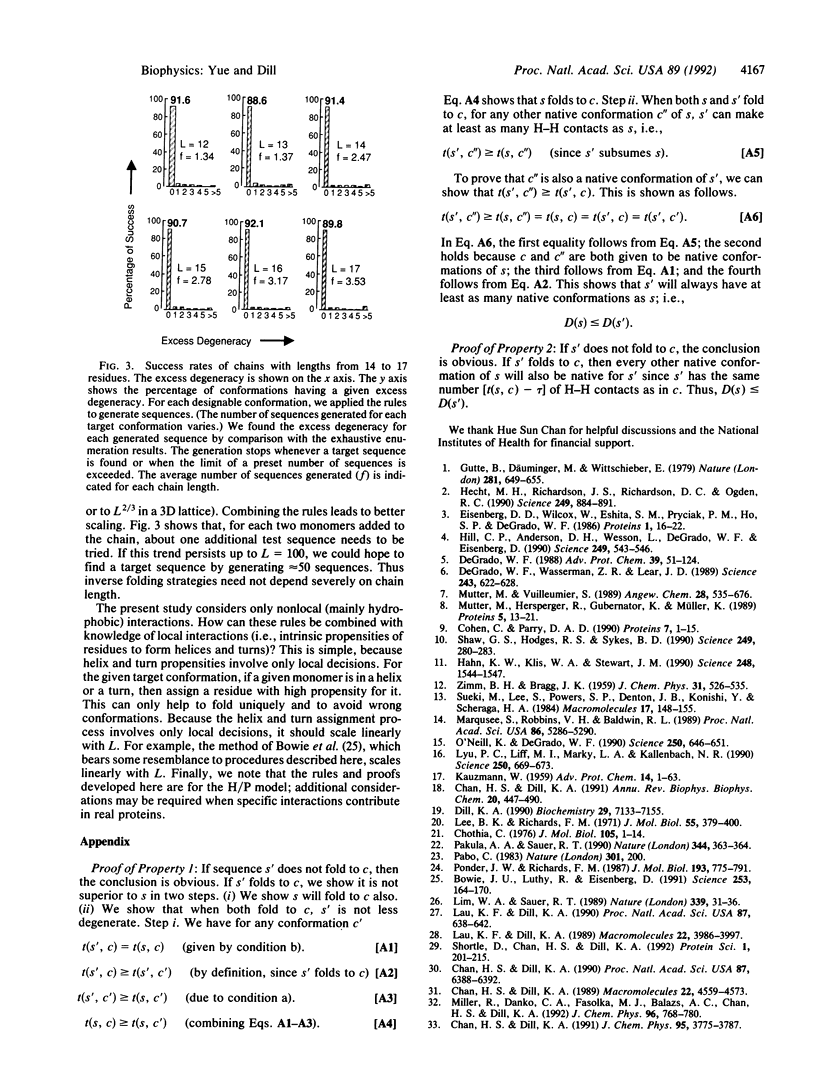
Selected References
These references are in PubMed. This may not be the complete list of references from this article.
- Bowie J. U., Lüthy R., Eisenberg D. A method to identify protein sequences that fold into a known three-dimensional structure. Science. 1991 Jul 12;253(5016):164–170. doi: 10.1126/science.1853201. [DOI] [PubMed] [Google Scholar]
- Chan H. S., Dill K. A. Origins of structure in globular proteins. Proc Natl Acad Sci U S A. 1990 Aug;87(16):6388–6392. doi: 10.1073/pnas.87.16.6388. [DOI] [PMC free article] [PubMed] [Google Scholar]
- Chan H. S., Dill K. A. Polymer principles in protein structure and stability. Annu Rev Biophys Biophys Chem. 1991;20:447–490. doi: 10.1146/annurev.bb.20.060191.002311. [DOI] [PubMed] [Google Scholar]
- Chothia C. The nature of the accessible and buried surfaces in proteins. J Mol Biol. 1976 Jul 25;105(1):1–12. doi: 10.1016/0022-2836(76)90191-1. [DOI] [PubMed] [Google Scholar]
- Cohen C., Parry D. A. Alpha-helical coiled coils and bundles: how to design an alpha-helical protein. Proteins. 1990;7(1):1–15. doi: 10.1002/prot.340070102. [DOI] [PubMed] [Google Scholar]
- DeGrado W. F., Wasserman Z. R., Lear J. D. Protein design, a minimalist approach. Science. 1989 Feb 3;243(4891):622–628. doi: 10.1126/science.2464850. [DOI] [PubMed] [Google Scholar]
- Degrado W. F. Design of peptides and proteins. Adv Protein Chem. 1988;39:51–124. doi: 10.1016/s0065-3233(08)60375-7. [DOI] [PubMed] [Google Scholar]
- Dill K. A. Dominant forces in protein folding. Biochemistry. 1990 Aug 7;29(31):7133–7155. doi: 10.1021/bi00483a001. [DOI] [PubMed] [Google Scholar]
- Eisenberg D., Wilcox W., Eshita S. M., Pryciak P. M., Ho S. P., DeGrado W. F. The design, synthesis, and crystallization of an alpha-helical peptide. Proteins. 1986 Sep;1(1):16–22. doi: 10.1002/prot.340010105. [DOI] [PubMed] [Google Scholar]
- Gutte B., Däumigen M., Wittschieber E. Design, synthesis and characterisation of a 34-residue polypeptide that interacts with nucleic acids. Nature. 1979 Oct 25;281(5733):650–655. doi: 10.1038/281650a0. [DOI] [PubMed] [Google Scholar]
- Hahn K. W., Klis W. A., Stewart J. M. Design and synthesis of a peptide having chymotrypsin-like esterase activity. Science. 1990 Jun 22;248(4962):1544–1547. doi: 10.1126/science.2360048. [DOI] [PubMed] [Google Scholar]
- Hecht M. H., Richardson J. S., Richardson D. C., Ogden R. C. De novo design, expression, and characterization of Felix: a four-helix bundle protein of native-like sequence. Science. 1990 Aug 24;249(4971):884–891. doi: 10.1126/science.2392678. [DOI] [PubMed] [Google Scholar]
- Hill C. P., Anderson D. H., Wesson L., DeGrado W. F., Eisenberg D. Crystal structure of alpha 1: implications for protein design. Science. 1990 Aug 3;249(4968):543–546. doi: 10.1126/science.2382133. [DOI] [PubMed] [Google Scholar]
- KAUZMANN W. Some factors in the interpretation of protein denaturation. Adv Protein Chem. 1959;14:1–63. doi: 10.1016/s0065-3233(08)60608-7. [DOI] [PubMed] [Google Scholar]
- Lau K. F., Dill K. A. Theory for protein mutability and biogenesis. Proc Natl Acad Sci U S A. 1990 Jan;87(2):638–642. doi: 10.1073/pnas.87.2.638. [DOI] [PMC free article] [PubMed] [Google Scholar]
- Lee B., Richards F. M. The interpretation of protein structures: estimation of static accessibility. J Mol Biol. 1971 Feb 14;55(3):379–400. doi: 10.1016/0022-2836(71)90324-x. [DOI] [PubMed] [Google Scholar]
- Lim W. A., Sauer R. T. Alternative packing arrangements in the hydrophobic core of lambda repressor. Nature. 1989 May 4;339(6219):31–36. doi: 10.1038/339031a0. [DOI] [PubMed] [Google Scholar]
- Lyu P. C., Liff M. I., Marky L. A., Kallenbach N. R. Side chain contributions to the stability of alpha-helical structure in peptides. Science. 1990 Nov 2;250(4981):669–673. doi: 10.1126/science.2237416. [DOI] [PubMed] [Google Scholar]
- Marqusee S., Robbins V. H., Baldwin R. L. Unusually stable helix formation in short alanine-based peptides. Proc Natl Acad Sci U S A. 1989 Jul;86(14):5286–5290. doi: 10.1073/pnas.86.14.5286. [DOI] [PMC free article] [PubMed] [Google Scholar]
- Mutter M., Hersperger R., Gubernator K., Müller K. The construction of new proteins: V. A template-assembled synthetic protein (TASP) containing both a 4-helix bundle and beta-barrel-like structure. Proteins. 1989;5(1):13–21. doi: 10.1002/prot.340050104. [DOI] [PubMed] [Google Scholar]
- O'Neil K. T., DeGrado W. F. A thermodynamic scale for the helix-forming tendencies of the commonly occurring amino acids. Science. 1990 Nov 2;250(4981):646–651. doi: 10.1126/science.2237415. [DOI] [PubMed] [Google Scholar]
- Pabo C. Molecular technology. Designing proteins and peptides. Nature. 1983 Jan 20;301(5897):200–200. doi: 10.1038/301200a0. [DOI] [PubMed] [Google Scholar]
- Pakula A. A., Sauer R. T. Reverse hydrophobic effects relieved by amino-acid substitutions at a protein surface. Nature. 1990 Mar 22;344(6264):363–364. doi: 10.1038/344363a0. [DOI] [PubMed] [Google Scholar]
- Ponder J. W., Richards F. M. Tertiary templates for proteins. Use of packing criteria in the enumeration of allowed sequences for different structural classes. J Mol Biol. 1987 Feb 20;193(4):775–791. doi: 10.1016/0022-2836(87)90358-5. [DOI] [PubMed] [Google Scholar]
- Shaw G. S., Hodges R. S., Sykes B. D. Calcium-induced peptide association to form an intact protein domain: 1H NMR structural evidence. Science. 1990 Jul 20;249(4966):280–283. doi: 10.1126/science.2374927. [DOI] [PubMed] [Google Scholar]
- Shortle D., Chan H. S., Dill K. A. Modeling the effects of mutations on the denatured states of proteins. Protein Sci. 1992 Feb;1(2):201–215. doi: 10.1002/pro.5560010202. [DOI] [PMC free article] [PubMed] [Google Scholar]