Abstract
A minimal model for the mitotic oscillator is presented. The model, built on recent experimental advances, is based on the cascade of post-translational modification that modulates the activity of cdc2 kinase during the cell cycle. The model pertains to the situation encountered in early amphibian embryos, where the accumulation of cyclin suffices to trigger the onset of mitosis. In the first cycle of the bicyclic cascade model, cyclin promotes the activation of cdc2 kinase through reversible dephosphorylation, and in the second cycle, cdc2 kinase activates a cyclin protease by reversible phosphorylation. That cyclin activates cdc2 kinase while the kinase triggers the degradation of cyclin has suggested that oscillations may originate from such a negative feedback loop [Félix, M. A., Labbé, J. C., Dorée, M., Hunt, T. & Karsenti, E. (1990) Nature (London) 346, 379-382]. This conjecture is corroborated by the model, which indicates that sustained oscillations of the limit cycle type can arise in the cascade, provided that a threshold exists in the activation of cdc2 kinase by cyclin and in the activation of cyclin proteolysis by cdc2 kinase. The analysis shows how miototic oscillations may readily arise from time lags associated with these thresholds and from the delayed negative feedback provided by cdc2-induced cyclin degradation. A mechanism for the origin of the thresholds is proposed in terms of the phenomenon of zero-order ultrasensitivity previously described for biochemical systems regulated by covalent modification.
Full text
PDF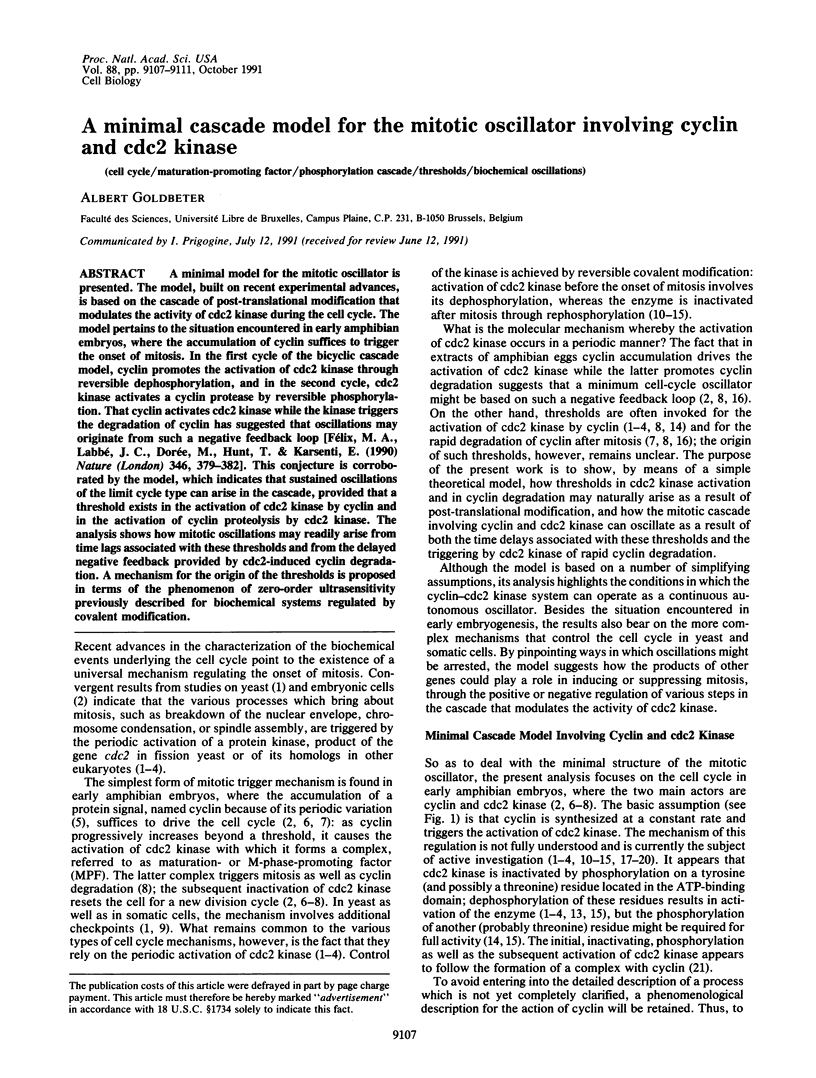
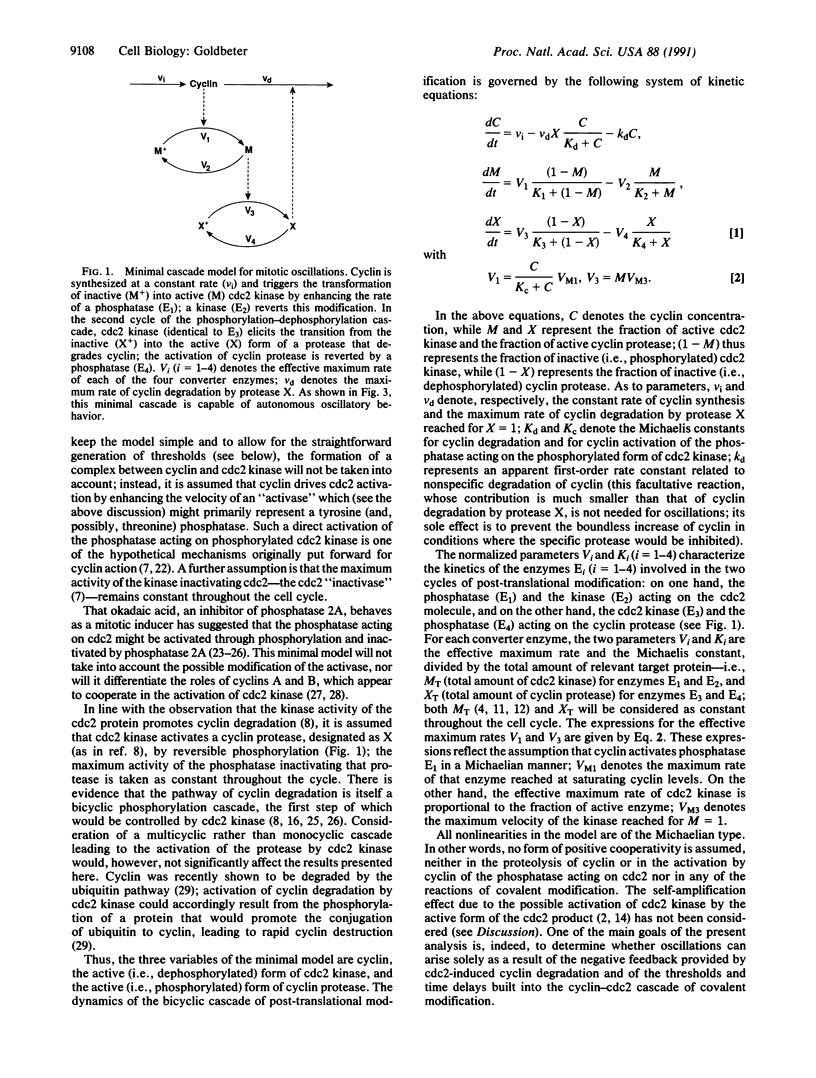
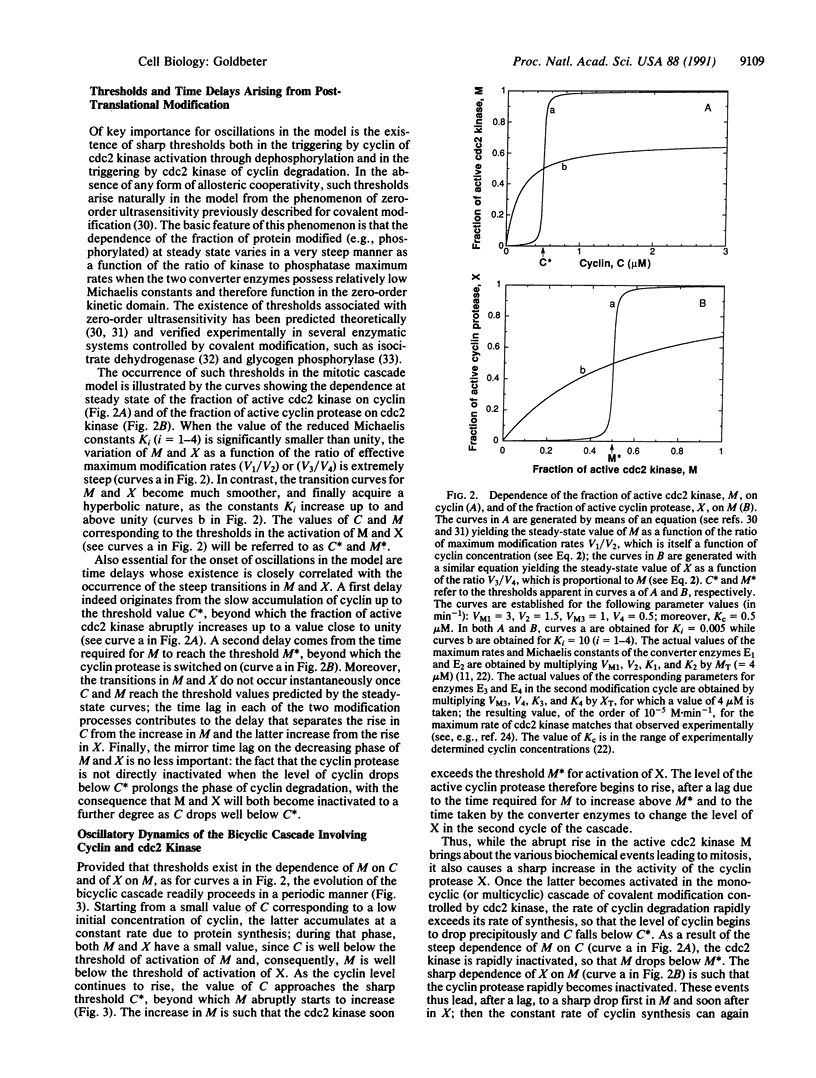
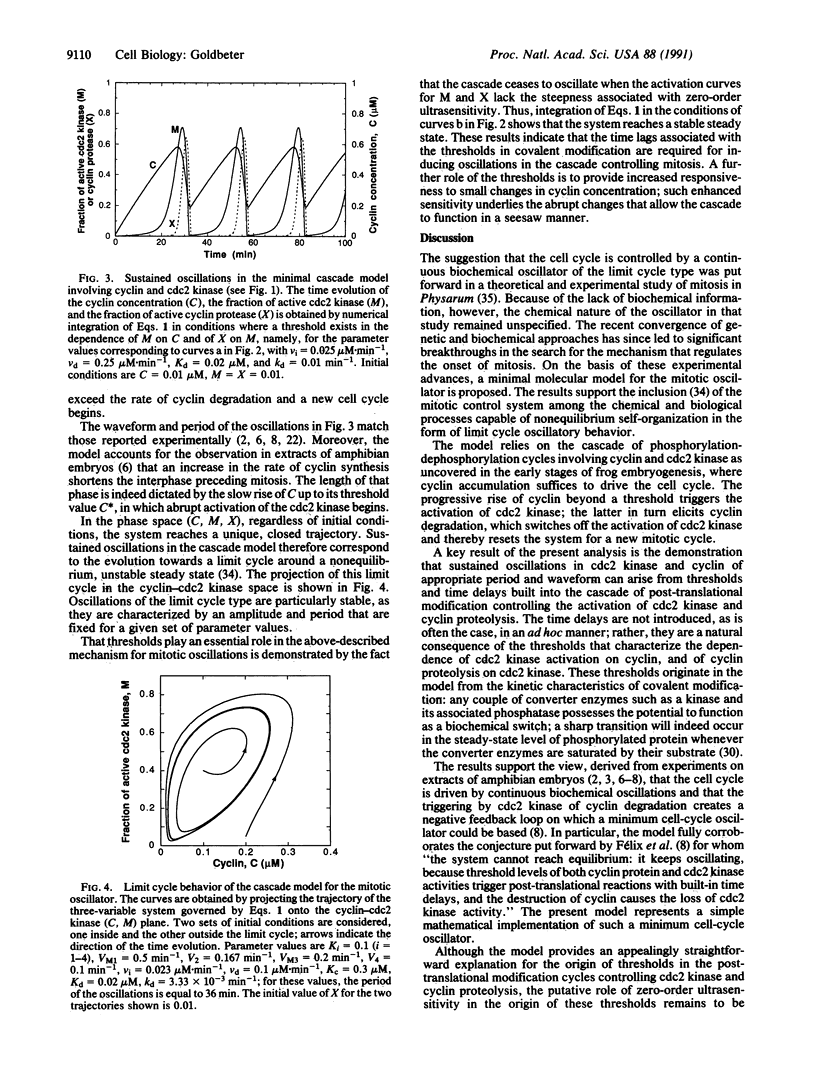
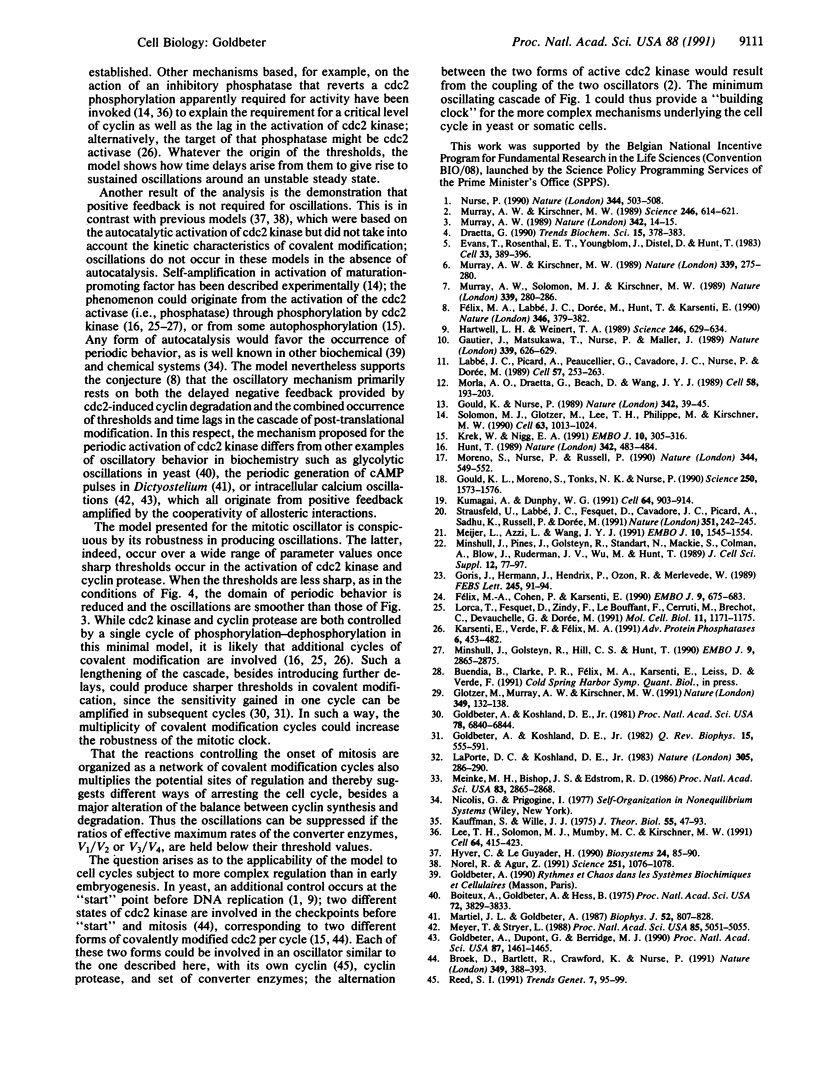
Selected References
These references are in PubMed. This may not be the complete list of references from this article.
- Boiteux A., Goldbeter A., Hess B. Control of oscillating glycolysis of yeast by stochastic, periodic, and steady source of substrate: a model and experimental study. Proc Natl Acad Sci U S A. 1975 Oct;72(10):3829–3833. doi: 10.1073/pnas.72.10.3829. [DOI] [PMC free article] [PubMed] [Google Scholar]
- Broek D., Bartlett R., Crawford K., Nurse P. Involvement of p34cdc2 in establishing the dependency of S phase on mitosis. Nature. 1991 Jan 31;349(6308):388–393. doi: 10.1038/349388a0. [DOI] [PubMed] [Google Scholar]
- Draetta G. Cell cycle control in eukaryotes: molecular mechanisms of cdc2 activation. Trends Biochem Sci. 1990 Oct;15(10):378–383. doi: 10.1016/0968-0004(90)90235-4. [DOI] [PubMed] [Google Scholar]
- Evans T., Rosenthal E. T., Youngblom J., Distel D., Hunt T. Cyclin: a protein specified by maternal mRNA in sea urchin eggs that is destroyed at each cleavage division. Cell. 1983 Jun;33(2):389–396. doi: 10.1016/0092-8674(83)90420-8. [DOI] [PubMed] [Google Scholar]
- Félix M. A., Cohen P., Karsenti E. Cdc2 H1 kinase is negatively regulated by a type 2A phosphatase in the Xenopus early embryonic cell cycle: evidence from the effects of okadaic acid. EMBO J. 1990 Mar;9(3):675–683. doi: 10.1002/j.1460-2075.1990.tb08159.x. [DOI] [PMC free article] [PubMed] [Google Scholar]
- Félix M. A., Labbé J. C., Dorée M., Hunt T., Karsenti E. Triggering of cyclin degradation in interphase extracts of amphibian eggs by cdc2 kinase. Nature. 1990 Jul 26;346(6282):379–382. doi: 10.1038/346379a0. [DOI] [PubMed] [Google Scholar]
- Gautier J., Matsukawa T., Nurse P., Maller J. Dephosphorylation and activation of Xenopus p34cdc2 protein kinase during the cell cycle. Nature. 1989 Jun 22;339(6226):626–629. doi: 10.1038/339626a0. [DOI] [PubMed] [Google Scholar]
- Glotzer M., Murray A. W., Kirschner M. W. Cyclin is degraded by the ubiquitin pathway. Nature. 1991 Jan 10;349(6305):132–138. doi: 10.1038/349132a0. [DOI] [PubMed] [Google Scholar]
- Goldbeter A., Dupont G., Berridge M. J. Minimal model for signal-induced Ca2+ oscillations and for their frequency encoding through protein phosphorylation. Proc Natl Acad Sci U S A. 1990 Feb;87(4):1461–1465. doi: 10.1073/pnas.87.4.1461. [DOI] [PMC free article] [PubMed] [Google Scholar]
- Goldbeter A., Koshland D. E., Jr An amplified sensitivity arising from covalent modification in biological systems. Proc Natl Acad Sci U S A. 1981 Nov;78(11):6840–6844. doi: 10.1073/pnas.78.11.6840. [DOI] [PMC free article] [PubMed] [Google Scholar]
- Goldbeter A., Koshland D. E., Jr Sensitivity amplification in biochemical systems. Q Rev Biophys. 1982 Aug;15(3):555–591. doi: 10.1017/s0033583500003449. [DOI] [PubMed] [Google Scholar]
- Goris J., Hermann J., Hendrix P., Ozon R., Merlevede W. Okadaic acid, a specific protein phosphatase inhibitor, induces maturation and MPF formation in Xenopus laevis oocytes. FEBS Lett. 1989 Mar 13;245(1-2):91–94. doi: 10.1016/0014-5793(89)80198-x. [DOI] [PubMed] [Google Scholar]
- Gould K. L., Moreno S., Tonks N. K., Nurse P. Complementation of the mitotic activator, p80cdc25, by a human protein-tyrosine phosphatase. Science. 1990 Dec 14;250(4987):1573–1576. doi: 10.1126/science.1703321. [DOI] [PubMed] [Google Scholar]
- Gould K. L., Nurse P. Tyrosine phosphorylation of the fission yeast cdc2+ protein kinase regulates entry into mitosis. Nature. 1989 Nov 2;342(6245):39–45. doi: 10.1038/342039a0. [DOI] [PubMed] [Google Scholar]
- Hartwell L. H., Weinert T. A. Checkpoints: controls that ensure the order of cell cycle events. Science. 1989 Nov 3;246(4930):629–634. doi: 10.1126/science.2683079. [DOI] [PubMed] [Google Scholar]
- Hunt T. Embryology. Under arrest in the cell cycle. Nature. 1989 Nov 30;342(6249):483–484. doi: 10.1038/342483a0. [DOI] [PubMed] [Google Scholar]
- Hyver C., Le Guyader H. MPF and cyclin: modelling of the cell cycle minimum oscillator. Biosystems. 1990;24(2):85–90. doi: 10.1016/0303-2647(90)90001-h. [DOI] [PubMed] [Google Scholar]
- Kauffman S., Wille J. J. The mitotic oscillator in Physarum polycephalum. J Theor Biol. 1975 Nov;55(1):47–93. doi: 10.1016/s0022-5193(75)80108-1. [DOI] [PubMed] [Google Scholar]
- Krek W., Nigg E. A. Differential phosphorylation of vertebrate p34cdc2 kinase at the G1/S and G2/M transitions of the cell cycle: identification of major phosphorylation sites. EMBO J. 1991 Feb;10(2):305–316. doi: 10.1002/j.1460-2075.1991.tb07951.x. [DOI] [PMC free article] [PubMed] [Google Scholar]
- Kumagai A., Dunphy W. G. The cdc25 protein controls tyrosine dephosphorylation of the cdc2 protein in a cell-free system. Cell. 1991 Mar 8;64(5):903–914. doi: 10.1016/0092-8674(91)90315-p. [DOI] [PubMed] [Google Scholar]
- LaPorte D. C., Koshland D. E., Jr Phosphorylation of isocitrate dehydrogenase as a demonstration of enhanced sensitivity in covalent regulation. Nature. 1983 Sep 22;305(5932):286–290. doi: 10.1038/305286a0. [DOI] [PubMed] [Google Scholar]
- Labbe J. C., Picard A., Peaucellier G., Cavadore J. C., Nurse P., Doree M. Purification of MPF from starfish: identification as the H1 histone kinase p34cdc2 and a possible mechanism for its periodic activation. Cell. 1989 Apr 21;57(2):253–263. doi: 10.1016/0092-8674(89)90963-x. [DOI] [PubMed] [Google Scholar]
- Lee T. H., Solomon M. J., Mumby M. C., Kirschner M. W. INH, a negative regulator of MPF, is a form of protein phosphatase 2A. Cell. 1991 Jan 25;64(2):415–423. doi: 10.1016/0092-8674(91)90649-j. [DOI] [PubMed] [Google Scholar]
- Lorca T., Fesquet D., Zindy F., Le Bouffant F., Cerruti M., Brechot C., Devauchelle G., Dorée M. An okadaic acid-sensitive phosphatase negatively controls the cyclin degradation pathway in amphibian eggs. Mol Cell Biol. 1991 Feb;11(2):1171–1175. doi: 10.1128/mcb.11.2.1171. [DOI] [PMC free article] [PubMed] [Google Scholar]
- Martiel J. L., Goldbeter A. A Model Based on Receptor Desensitization for Cyclic AMP Signaling in Dictyostelium Cells. Biophys J. 1987 Nov;52(5):807–828. doi: 10.1016/S0006-3495(87)83275-7. [DOI] [PMC free article] [PubMed] [Google Scholar]
- Meijer L., Azzi L., Wang J. Y. Cyclin B targets p34cdc2 for tyrosine phosphorylation. EMBO J. 1991 Jun;10(6):1545–1554. doi: 10.1002/j.1460-2075.1991.tb07674.x. [DOI] [PMC free article] [PubMed] [Google Scholar]
- Meinke M. H., Bishop J. S., Edstrom R. D. Zero-order ultrasensitivity in the regulation of glycogen phosphorylase. Proc Natl Acad Sci U S A. 1986 May;83(9):2865–2868. doi: 10.1073/pnas.83.9.2865. [DOI] [PMC free article] [PubMed] [Google Scholar]
- Meyer T., Stryer L. Molecular model for receptor-stimulated calcium spiking. Proc Natl Acad Sci U S A. 1988 Jul;85(14):5051–5055. doi: 10.1073/pnas.85.14.5051. [DOI] [PMC free article] [PubMed] [Google Scholar]
- Minshull J., Golsteyn R., Hill C. S., Hunt T. The A- and B-type cyclin associated cdc2 kinases in Xenopus turn on and off at different times in the cell cycle. EMBO J. 1990 Sep;9(9):2865–2875. doi: 10.1002/j.1460-2075.1990.tb07476.x. [DOI] [PMC free article] [PubMed] [Google Scholar]
- Minshull J., Pines J., Golsteyn R., Standart N., Mackie S., Colman A., Blow J., Ruderman J. V., Wu M., Hunt T. The role of cyclin synthesis, modification and destruction in the control of cell division. J Cell Sci Suppl. 1989;12:77–97. doi: 10.1242/jcs.1989.supplement_12.8. [DOI] [PubMed] [Google Scholar]
- Moreno S., Nurse P., Russell P. Regulation of mitosis by cyclic accumulation of p80cdc25 mitotic inducer in fission yeast. Nature. 1990 Apr 5;344(6266):549–552. doi: 10.1038/344549a0. [DOI] [PubMed] [Google Scholar]
- Morla A. O., Draetta G., Beach D., Wang J. Y. Reversible tyrosine phosphorylation of cdc2: dephosphorylation accompanies activation during entry into mitosis. Cell. 1989 Jul 14;58(1):193–203. doi: 10.1016/0092-8674(89)90415-7. [DOI] [PubMed] [Google Scholar]
- Murray A. W. Cell biology: the cell cycle as a cdc2 cycle. Nature. 1989 Nov 2;342(6245):14–15. doi: 10.1038/342014a0. [DOI] [PubMed] [Google Scholar]
- Murray A. W., Kirschner M. W. Cyclin synthesis drives the early embryonic cell cycle. Nature. 1989 May 25;339(6222):275–280. doi: 10.1038/339275a0. [DOI] [PubMed] [Google Scholar]
- Murray A. W., Kirschner M. W. Dominoes and clocks: the union of two views of the cell cycle. Science. 1989 Nov 3;246(4930):614–621. doi: 10.1126/science.2683077. [DOI] [PubMed] [Google Scholar]
- Murray A. W., Solomon M. J., Kirschner M. W. The role of cyclin synthesis and degradation in the control of maturation promoting factor activity. Nature. 1989 May 25;339(6222):280–286. doi: 10.1038/339280a0. [DOI] [PubMed] [Google Scholar]
- Norel R., Agur Z. A model for the adjustment of the mitotic clock by cyclin and MPF levels. Science. 1991 Mar 1;251(4997):1076–1078. doi: 10.1126/science.1825521. [DOI] [PubMed] [Google Scholar]
- Nurse P. Universal control mechanism regulating onset of M-phase. Nature. 1990 Apr 5;344(6266):503–508. doi: 10.1038/344503a0. [DOI] [PubMed] [Google Scholar]
- Reed S. I. G1-specific cyclins: in search of an S-phase-promoting factor. Trends Genet. 1991 Mar;7(3):95–99. doi: 10.1016/0168-9525(91)90279-Y. [DOI] [PubMed] [Google Scholar]
- Solomon M. J., Glotzer M., Lee T. H., Philippe M., Kirschner M. W. Cyclin activation of p34cdc2. Cell. 1990 Nov 30;63(5):1013–1024. doi: 10.1016/0092-8674(90)90504-8. [DOI] [PubMed] [Google Scholar]
- Strausfeld U., Labbé J. C., Fesquet D., Cavadore J. C., Picard A., Sadhu K., Russell P., Dorée M. Dephosphorylation and activation of a p34cdc2/cyclin B complex in vitro by human CDC25 protein. Nature. 1991 May 16;351(6323):242–245. doi: 10.1038/351242a0. [DOI] [PubMed] [Google Scholar]