Abstract
Aspartate transcarbamoylase (EC 2.1.3.2) is extensively studied as a model for cooperativity and allostery. This enzyme shows cooperativity between the catalytic sites, and its activity is feedback inhibited by CTP and activated by ATP. These regulatory processes involve several interfaces between catalytic and regulatory chains as well as between domains within these two types of chains. As far as the regulatory chain is concerned, its two domains are in contact through a hydrophobic interface, in which a tyrosine residue is inserted in a pocket involving two leucine residues of the allosteric domain and a valine and a leucine residue of the zinc domain. To probe the possible implication of this hydrophobic core in the CTP and ATP regulatory effect, the tyrosine was replaced by a phenylalanine through oligonucleotide-directed mutagenesis. Interestingly, the resulting mutant shows a complete inversion of the ATP effect; it is now inhibited by ATP instead of being activated by this nucleotide triphosphate. This mutant remains normally sensitive to the feedback inhibitor CTP. This result shows that the hydrophobic interface between the two domains of the regulatory chain plays an important role in the discrimination between the regulatory signals promoted by the two allosteric effectors.
Full text
PDF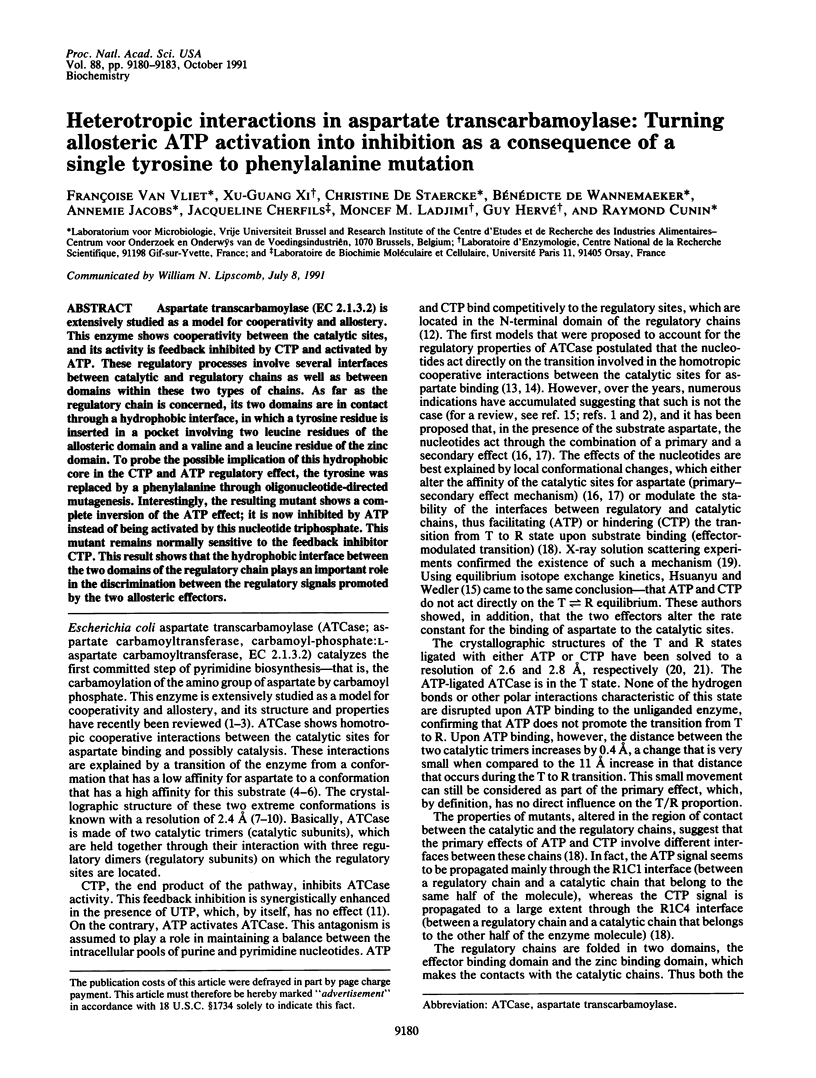
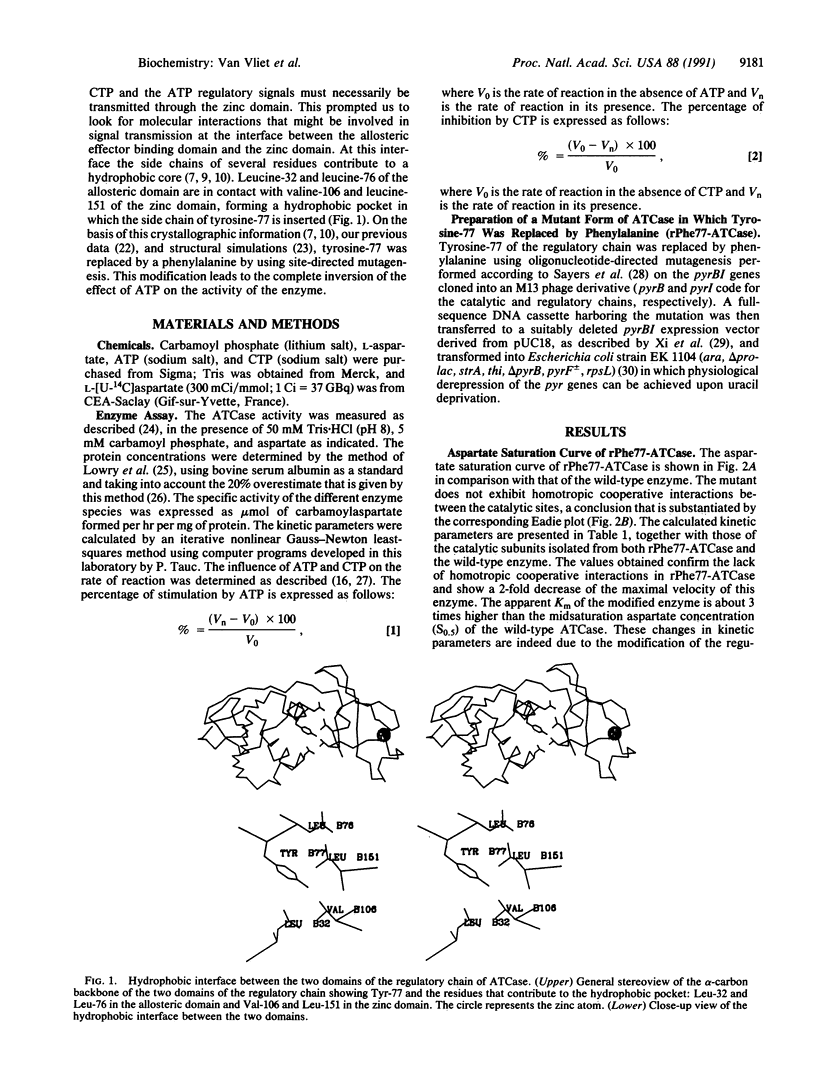
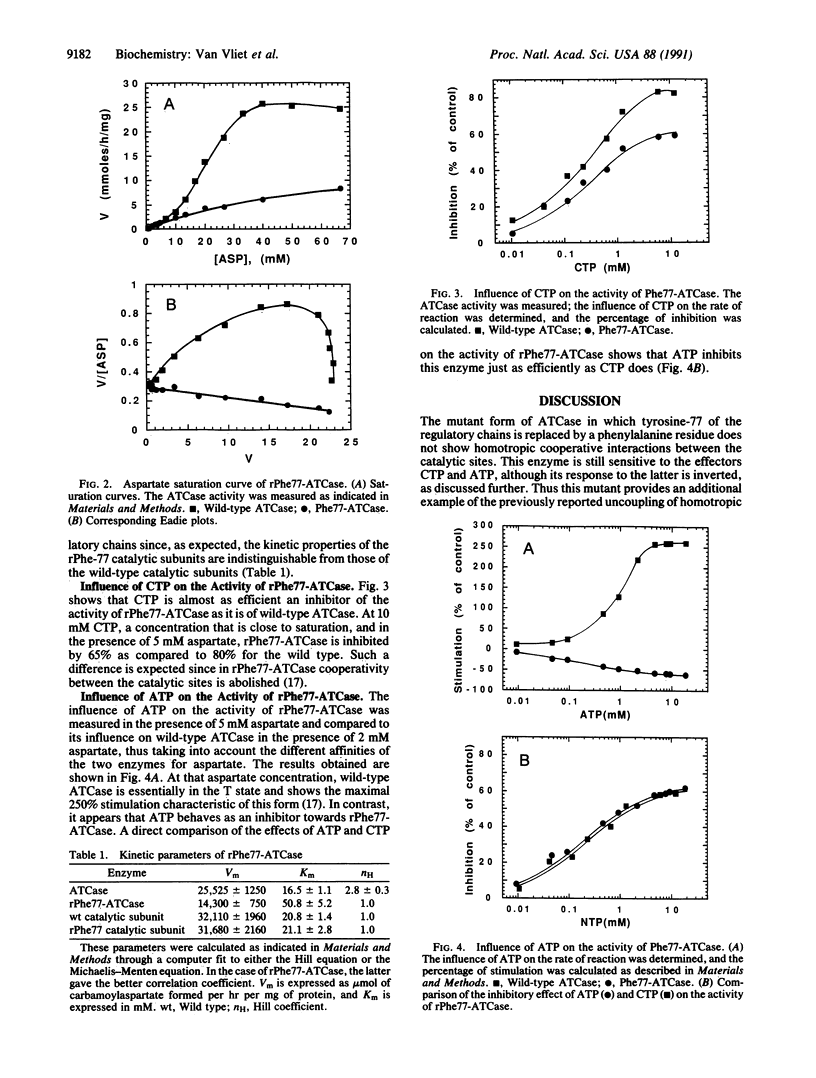
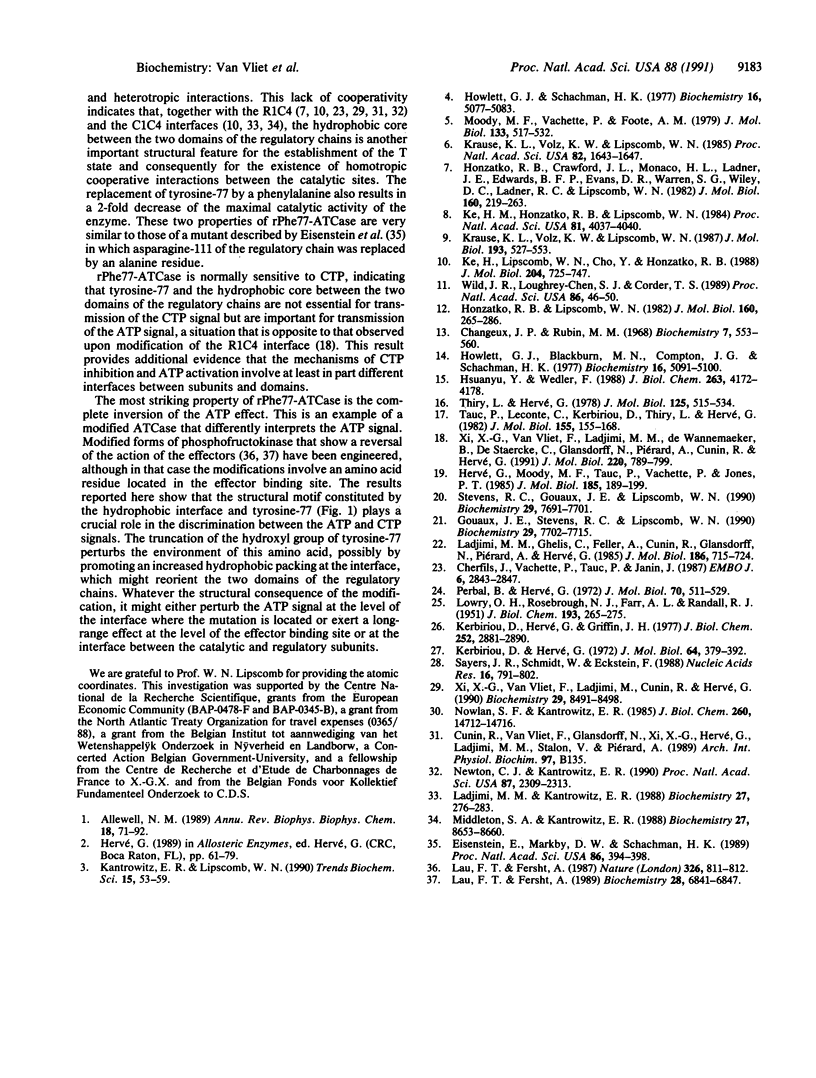
Selected References
These references are in PubMed. This may not be the complete list of references from this article.
- Allewell N. M. Escherichia coli aspartate transcarbamoylase: structure, energetics, and catalytic and regulatory mechanisms. Annu Rev Biophys Biophys Chem. 1989;18:71–92. doi: 10.1146/annurev.bb.18.060189.000443. [DOI] [PubMed] [Google Scholar]
- Changeux J. P., Rubin M. M. Allosteric interactions in aspartate transcarbamylase. 3. Interpretation of experimental data in terms of the model of Monod, Wyman, and Changeux. Biochemistry. 1968 Feb;7(2):553–561. doi: 10.1021/bi00842a601. [DOI] [PubMed] [Google Scholar]
- Cherfils J., Vachette P., Tauc P., Janin J. The pAR5 mutation and the allosteric mechanism of Escherichia coli aspartate carbamoyltransferase. EMBO J. 1987 Sep;6(9):2843–2847. doi: 10.1002/j.1460-2075.1987.tb02581.x. [DOI] [PMC free article] [PubMed] [Google Scholar]
- Gouaux J. E., Stevens R. C., Lipscomb W. N. Crystal structures of aspartate carbamoyltransferase ligated with phosphonoacetamide, malonate, and CTP or ATP at 2.8-A resolution and neutral pH. Biochemistry. 1990 Aug 21;29(33):7702–7715. doi: 10.1021/bi00485a020. [DOI] [PubMed] [Google Scholar]
- Hervé G., Moody M. F., Tauc P., Vachette P., Jones P. T. Quaternary structure changes in aspartate transcarbamylase studied by X-ray solution scattering. Signal transmission following effector binding. J Mol Biol. 1985 Sep 5;185(1):189–199. doi: 10.1016/0022-2836(85)90190-1. [DOI] [PubMed] [Google Scholar]
- Honzatko R. B., Crawford J. L., Monaco H. L., Ladner J. E., Ewards B. F., Evans D. R., Warren S. G., Wiley D. C., Ladner R. C., Lipscomb W. N. Crystal and molecular structures of native and CTP-liganded aspartate carbamoyltransferase from Escherichia coli. J Mol Biol. 1982 Sep 15;160(2):219–263. doi: 10.1016/0022-2836(82)90175-9. [DOI] [PubMed] [Google Scholar]
- Honzatko R. B., Lipscomb W. N. Interactions of phosphate ligands with Escherichia coli aspartate carbamoyltransferase in the crystalline state. J Mol Biol. 1982 Sep 15;160(2):265–286. doi: 10.1016/0022-2836(82)90176-0. [DOI] [PubMed] [Google Scholar]
- Howlett G. J., Blackburn M. N., Compton J. G., Schachman H. K. Allosteric regulation of aspartate transcarbamoylase. Analysis of the structural and functional behavior in terms of a two-state model. Biochemistry. 1977 Nov 15;16(23):5091–5100. doi: 10.1021/bi00642a023. [DOI] [PubMed] [Google Scholar]
- Howlett G. J., Schachman H. K. Allosteric regulation of aspartate transcarbamoylase. Changes in the sedimentation coefficient promoted by the bisubstrate analogue N-(phosphonacetyl)-L-aspartate. Biochemistry. 1977 Nov 15;16(23):5077–5083. doi: 10.1021/bi00642a021. [DOI] [PubMed] [Google Scholar]
- Hsuanyu Y. C., Wedler F. C. Effectors of Escherichia coli aspartate transcarbamoylase differentially perturb aspartate binding rather than the T-R transition. J Biol Chem. 1988 Mar 25;263(9):4172–4181. [PubMed] [Google Scholar]
- Kantrowitz E. R., Lipscomb W. N. Escherichia coli aspartate transcarbamoylase: the molecular basis for a concerted allosteric transition. Trends Biochem Sci. 1990 Feb;15(2):53–59. doi: 10.1016/0968-0004(90)90176-c. [DOI] [PubMed] [Google Scholar]
- Ke H. M., Honzatko R. B., Lipscomb W. N. Structure of unligated aspartate carbamoyltransferase of Escherichia coli at 2.6-A resolution. Proc Natl Acad Sci U S A. 1984 Jul;81(13):4037–4040. doi: 10.1073/pnas.81.13.4037. [DOI] [PMC free article] [PubMed] [Google Scholar]
- Ke H. M., Lipscomb W. N., Cho Y. J., Honzatko R. B. Complex of N-phosphonacetyl-L-aspartate with aspartate carbamoyltransferase. X-ray refinement, analysis of conformational changes and catalytic and allosteric mechanisms. J Mol Biol. 1988 Dec 5;204(3):725–747. doi: 10.1016/0022-2836(88)90365-8. [DOI] [PubMed] [Google Scholar]
- Kerbiriou D., Hervé G. An aspartate transcarbamylase lacking catalytic subunit interactions. Study of conformational changes by ultraviolet absorbance and circular dichroism spectroscopy. J Biol Chem. 1977 May 10;252(9):2881–2890. [PubMed] [Google Scholar]
- Kerbiriou D., Hervé G. Biosynthesis of an aspartate transcarbamylase lacking co-operative interactions. I. Disconnection of homotropic and heterotropic interactions under the influence of 2-thiouracil. J Mol Biol. 1972 Mar 14;64(2):379–392. doi: 10.1016/0022-2836(72)90505-0. [DOI] [PubMed] [Google Scholar]
- Krause K. L., Volz K. W., Lipscomb W. N. 2.5 A structure of aspartate carbamoyltransferase complexed with the bisubstrate analog N-(phosphonacetyl)-L-aspartate. J Mol Biol. 1987 Feb 5;193(3):527–553. doi: 10.1016/0022-2836(87)90265-8. [DOI] [PubMed] [Google Scholar]
- Krause K. L., Volz K. W., Lipscomb W. N. Structure at 2.9-A resolution of aspartate carbamoyltransferase complexed with the bisubstrate analogue N-(phosphonacetyl)-L-aspartate. Proc Natl Acad Sci U S A. 1985 Mar;82(6):1643–1647. doi: 10.1073/pnas.82.6.1643. [DOI] [PMC free article] [PubMed] [Google Scholar]
- LOWRY O. H., ROSEBROUGH N. J., FARR A. L., RANDALL R. J. Protein measurement with the Folin phenol reagent. J Biol Chem. 1951 Nov;193(1):265–275. [PubMed] [Google Scholar]
- Ladjimi M. M., Ghellis C., Feller A., Cunin R., Glansdorff N., Piérard A., Hervé G. Structure-function relationship in allosteric aspartate carbamoyltransferase from Escherichia coli. II. Involvement of the C-terminal region of the regulatory chain in homotropic and heterotropic interactions. J Mol Biol. 1985 Dec 20;186(4):715–724. doi: 10.1016/0022-2836(85)90391-2. [DOI] [PubMed] [Google Scholar]
- Ladjimi M. M., Kantrowitz E. R. A possible model for the concerted allosteric transition in Escherichia coli aspartate transcarbamylase as deduced from site-directed mutagenesis studies. Biochemistry. 1988 Jan 12;27(1):276–283. doi: 10.1021/bi00401a042. [DOI] [PubMed] [Google Scholar]
- Lau F. T., Fersht A. R. Conversion of allosteric inhibition to activation in phosphofructokinase by protein engineering. Nature. 1987 Apr 23;326(6115):811–812. doi: 10.1038/326811a0. [DOI] [PubMed] [Google Scholar]
- Lau F. T., Fersht A. R. Dissection of the effector-binding site and complementation studies of Escherichia coli phosphofructokinase using site-directed mutagenesis. Biochemistry. 1989 Aug 22;28(17):6841–6847. doi: 10.1021/bi00443a010. [DOI] [PubMed] [Google Scholar]
- Middleton S. A., Kantrowitz E. R. Function of arginine-234 and aspartic acid-271 in domain closure, cooperativity, and catalysis in Escherichia coli aspartate transcarbamylase. Biochemistry. 1988 Nov 15;27(23):8653–8660. doi: 10.1021/bi00423a022. [DOI] [PubMed] [Google Scholar]
- Moody M. F., Vachette P., Foote A. M. Changes in the x-ray solution scattering of aspartate transcarbamylase following the allosteric transition. J Mol Biol. 1979 Oct 9;133(4):517–532. doi: 10.1016/0022-2836(79)90405-4. [DOI] [PubMed] [Google Scholar]
- Newton C. J., Kantrowitz E. R. The regulatory subunit of Escherichia coli aspartate carbamoyltransferase may influence homotropic cooperativity and heterotropic interactions by a direct interaction with the loop containing residues 230-245 of the catalytic chain. Proc Natl Acad Sci U S A. 1990 Mar;87(6):2309–2313. doi: 10.1073/pnas.87.6.2309. [DOI] [PMC free article] [PubMed] [Google Scholar]
- Nowlan S. F., Kantrowitz E. R. Superproduction and rapid purification of Escherichia coli aspartate transcarbamylase and its catalytic subunit under extreme derepression of the pyrimidine pathway. J Biol Chem. 1985 Nov 25;260(27):14712–14716. [PubMed] [Google Scholar]
- Perbal B., Hervé G. Biosynthesis of Escherichia coli aspartate transcarbamylase. I. Parameters of gene expression and sequential biosynthesis of the subunits. J Mol Biol. 1972 Oct 14;70(3):511–529. doi: 10.1016/0022-2836(72)90556-6. [DOI] [PubMed] [Google Scholar]
- Sayers J. R., Schmidt W., Eckstein F. 5'-3' exonucleases in phosphorothioate-based oligonucleotide-directed mutagenesis. Nucleic Acids Res. 1988 Feb 11;16(3):791–802. doi: 10.1093/nar/16.3.791. [DOI] [PMC free article] [PubMed] [Google Scholar]
- Stevens R. C., Gouaux J. E., Lipscomb W. N. Structural consequences of effector binding to the T state of aspartate carbamoyltransferase: crystal structures of the unligated and ATP- and CTP-complexed enzymes at 2.6-A resolution. Biochemistry. 1990 Aug 21;29(33):7691–7701. doi: 10.1021/bi00485a019. [DOI] [PubMed] [Google Scholar]
- Tauc P., Leconte C., Kerbiriou D., Thiry L., Hervé G. Coupling of homotropic and heterotropic interactions in Escherichia coli aspartate transcarbamylase. J Mol Biol. 1982 Feb 25;155(2):155–168. doi: 10.1016/0022-2836(82)90442-9. [DOI] [PubMed] [Google Scholar]
- Thiry L., Hervé G. The stimulation of Escherichia coli aspartate transcarbamylase activity by adenosine triphosphate. Relation with the other regulatory conformational changes; a model. J Mol Biol. 1978 Nov 15;125(4):515–534. doi: 10.1016/0022-2836(78)90314-5. [DOI] [PubMed] [Google Scholar]
- Wild J. R., Loughrey-Chen S. J., Corder T. S. In the presence of CTP, UTP becomes an allosteric inhibitor of aspartate transcarbamoylase. Proc Natl Acad Sci U S A. 1989 Jan;86(1):46–50. doi: 10.1073/pnas.86.1.46. [DOI] [PMC free article] [PubMed] [Google Scholar]
- Xi X. G., Van Vliet F., Ladjimi M. M., Cunin R., Hervé G. The catalytic site of Escherichia coli aspartate transcarbamylase: interaction between histidine 134 and the carbonyl group of the substrate carbamyl phosphate. Biochemistry. 1990 Sep 11;29(36):8491–8498. doi: 10.1021/bi00488a041. [DOI] [PubMed] [Google Scholar]
- Xi X. G., van Vliet F., Ladjimi M. M., de Wannemaeker B., de Staercke C., Glansdorff N., Piérard A., Cunin R., Hervé G. Heterotropic interactions in Escherichia coli aspartate transcarbamylase. Subunit interfaces involved in CTP inhibition and ATP activation. J Mol Biol. 1991 Aug 5;220(3):789–799. doi: 10.1016/0022-2836(91)90118-p. [DOI] [PubMed] [Google Scholar]