Abstract
The constitutive xanthine dehydrogenase and the inducible 2-furoyl-coenzyme A (CoA) dehydrogenase could be labeled with [185W]tungstate. This labeling was used as a reporter to purify both labile proteins. The radioactivity cochromatographed predominantly with the residual enzymatic activity of both enzymes during the first purification steps. Both radioactive proteins were separated and purified to homogeneity. Antibodies raised against the larger protein also exhibited cross-reactivity toward the second smaller protein and removed xanthine dehydrogenase and 2-furoyl-CoA dehydrogenase activity up to 80 and 60% from the supernatant of cell extracts, respectively. With use of cell extract, Western immunoblots showed only two bands which correlated exactly with the activity stains for both enzymes after native polyacrylamide gel electrophoresis. Molybdate was absolutely required for incorporation of 185W, formation of cross-reacting material, and enzymatic activity. The latter parameters showed a perfect correlation. This evidence proves that the radioactive proteins were actually xanthine dehydrogenase and 2-furoyl-CoA dehydrogenase. The apparent molecular weight of the native xanthine dehydrogenase was about 300,000, and that of 2-furoyl-CoA dehydrogenase was 150,000. Sodium dodecyl sulfate-polyacrylamide gel electrophoresis of both enzymes revealed two protein bands corresponding to molecular weights of 55,000 and 25,000. The xanthine dehydrogenase contained at least 1.6 mol of molybdenum, 0.9 ml of cytochrome b, 5.8 mol of iron, and 2.4 mol of labile sulfur per mol of enzyme. The composition of the 2-furoyl-CoA dehydrogenase seemed to be similar, although the stoichiometry was not determined. The oxidation of furfuryl alcohol to furfural and further to 2-furoic acid by Pseudomonas putida Fu1 was catalyzed by two different dehydrogenases.
Full text
PDF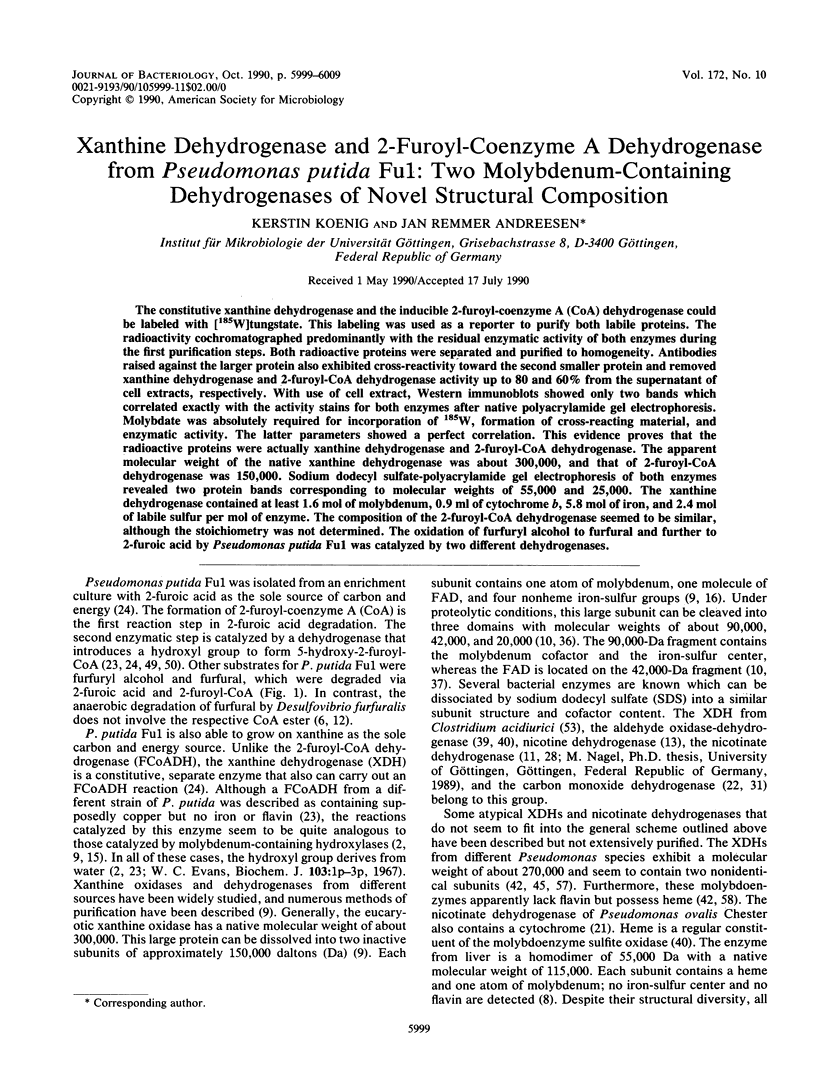
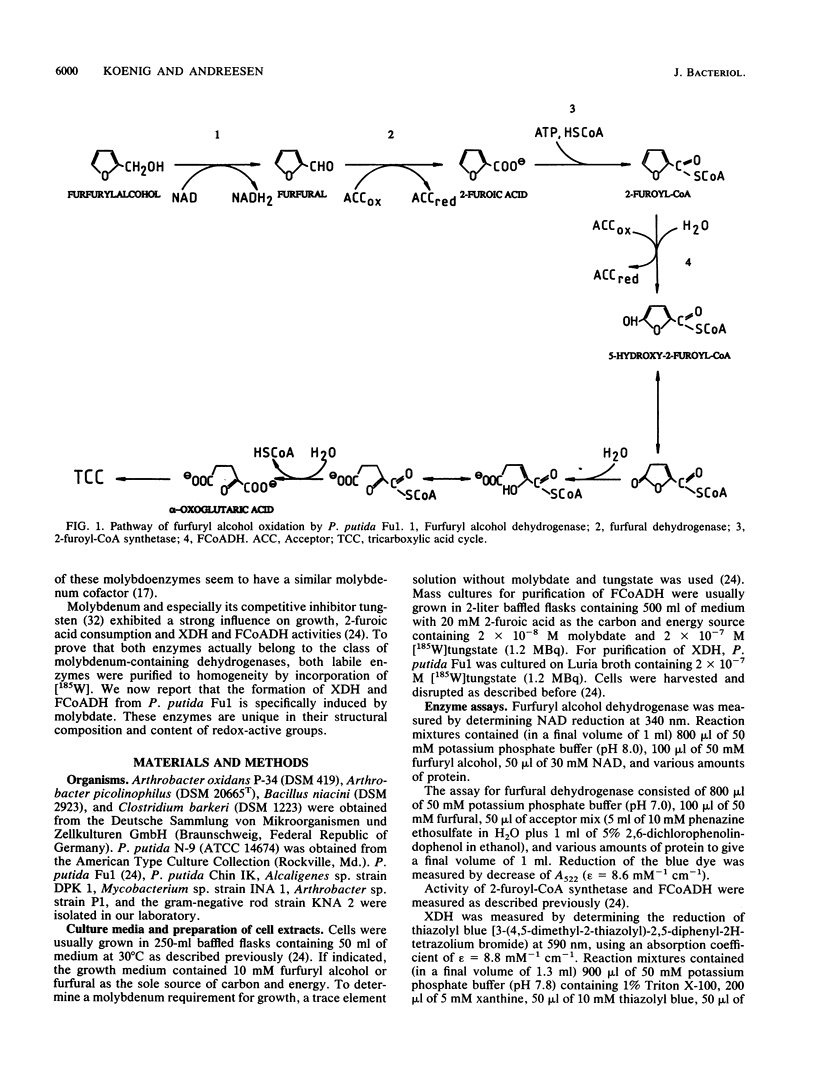
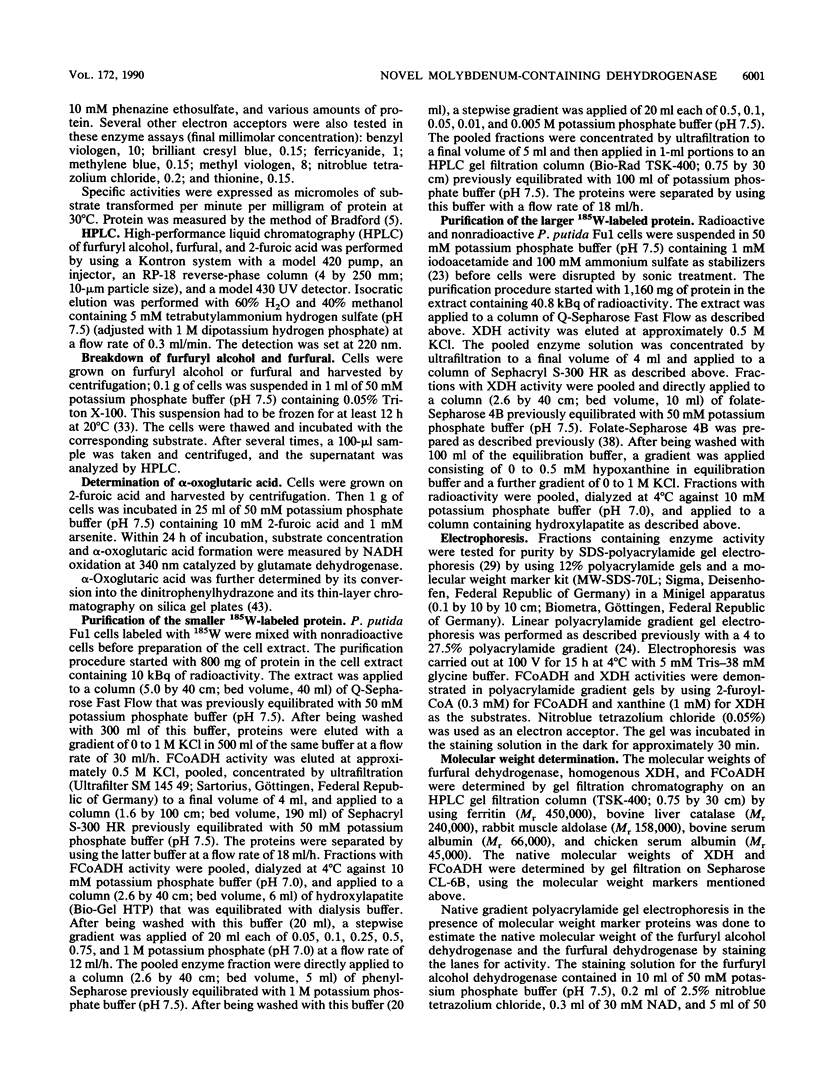
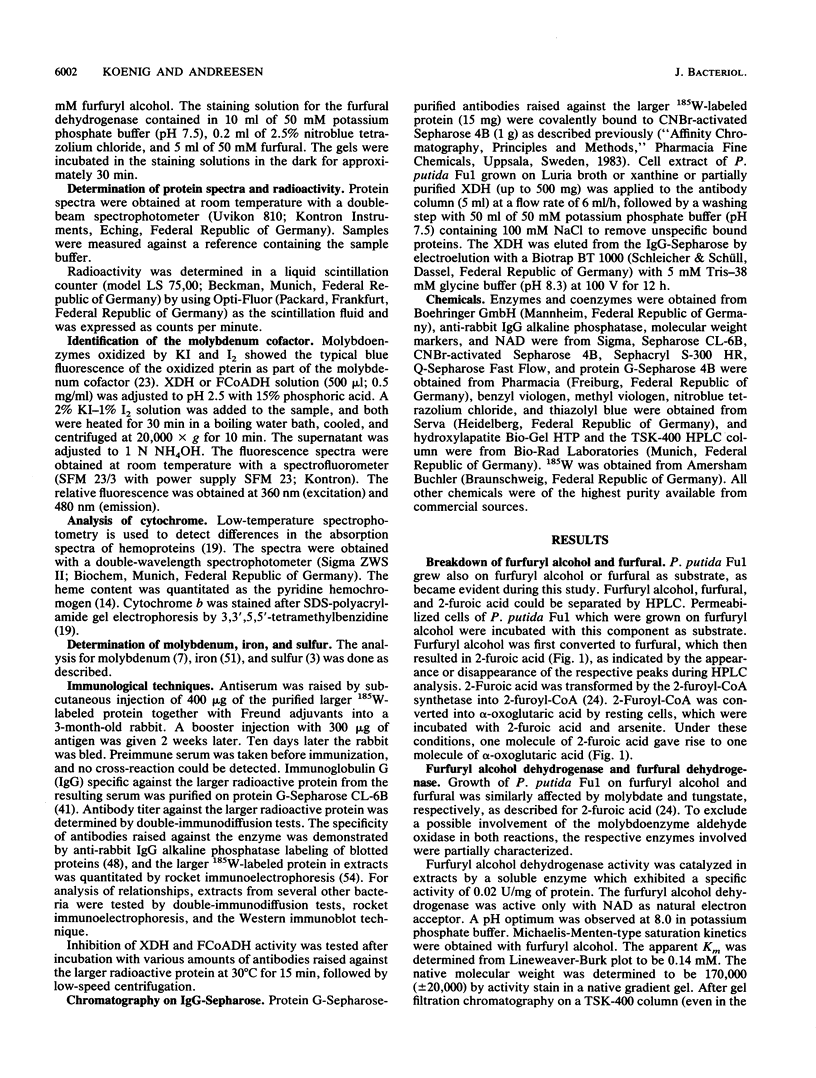
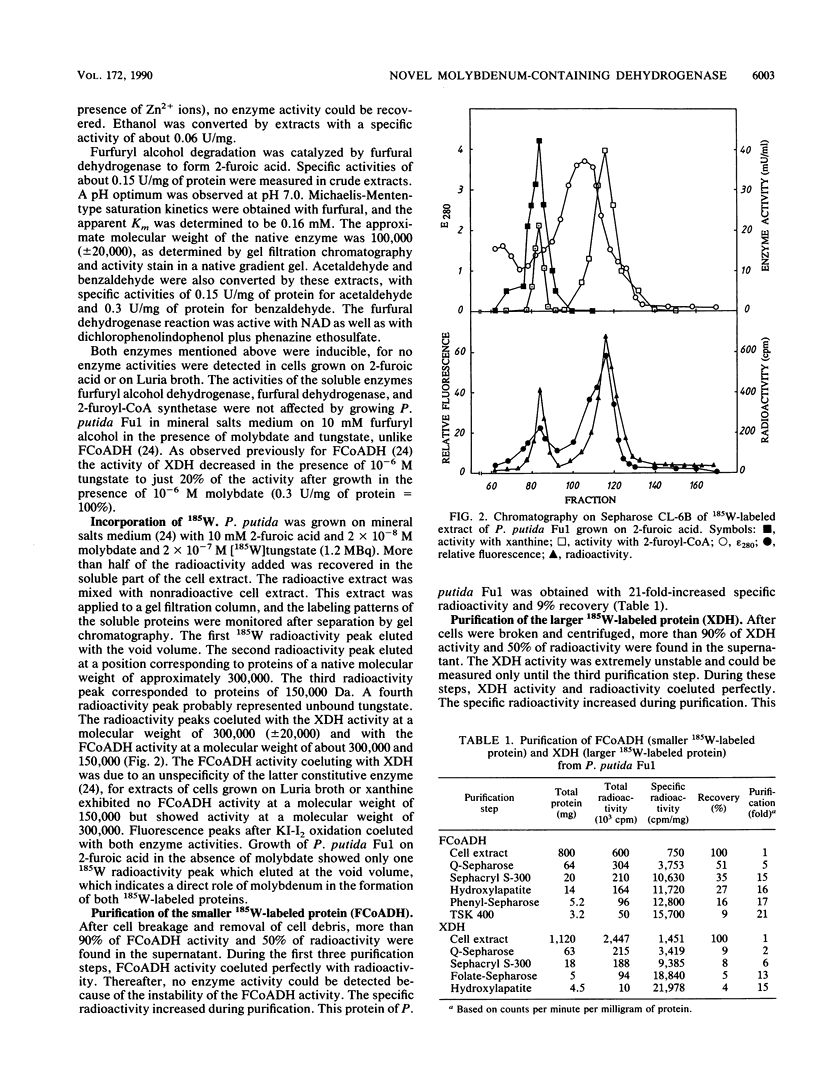
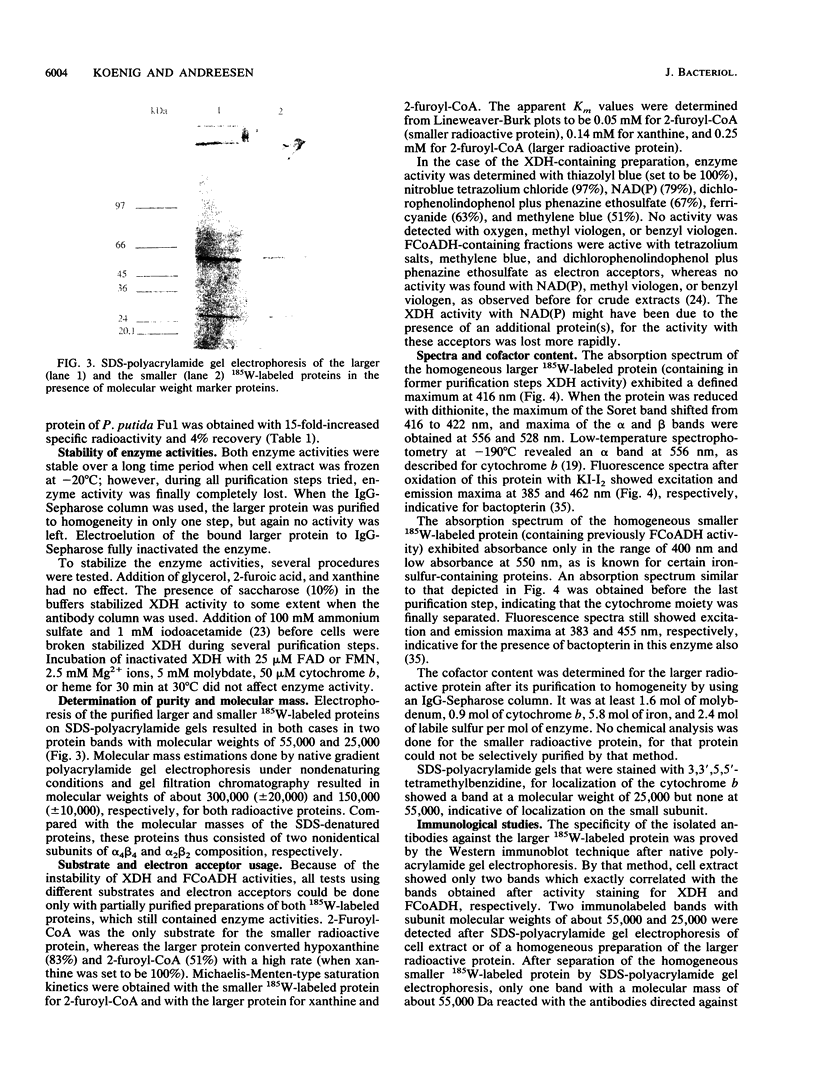
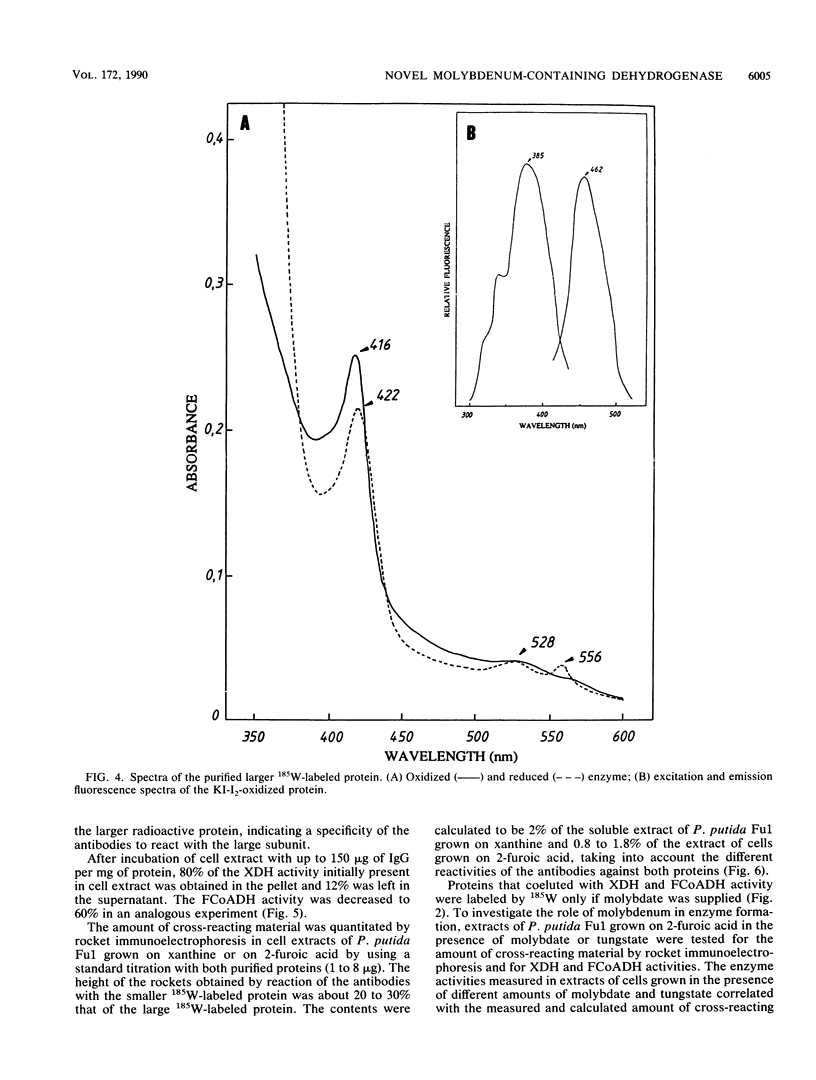
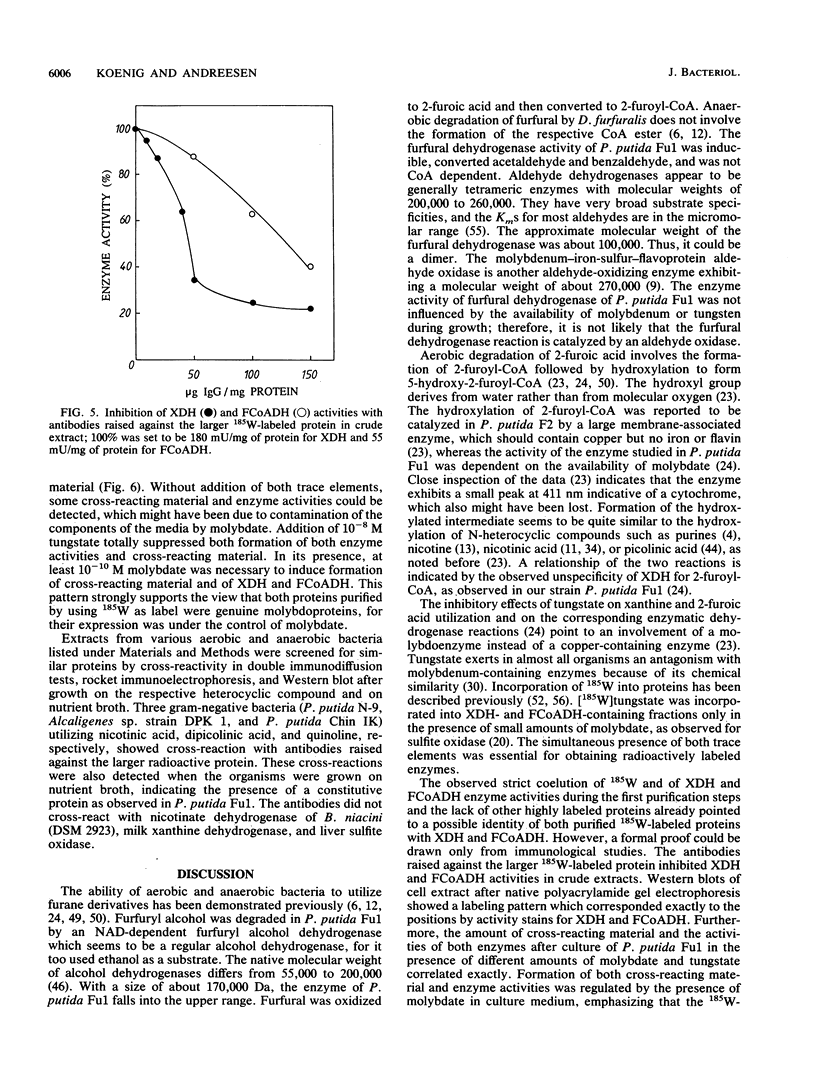
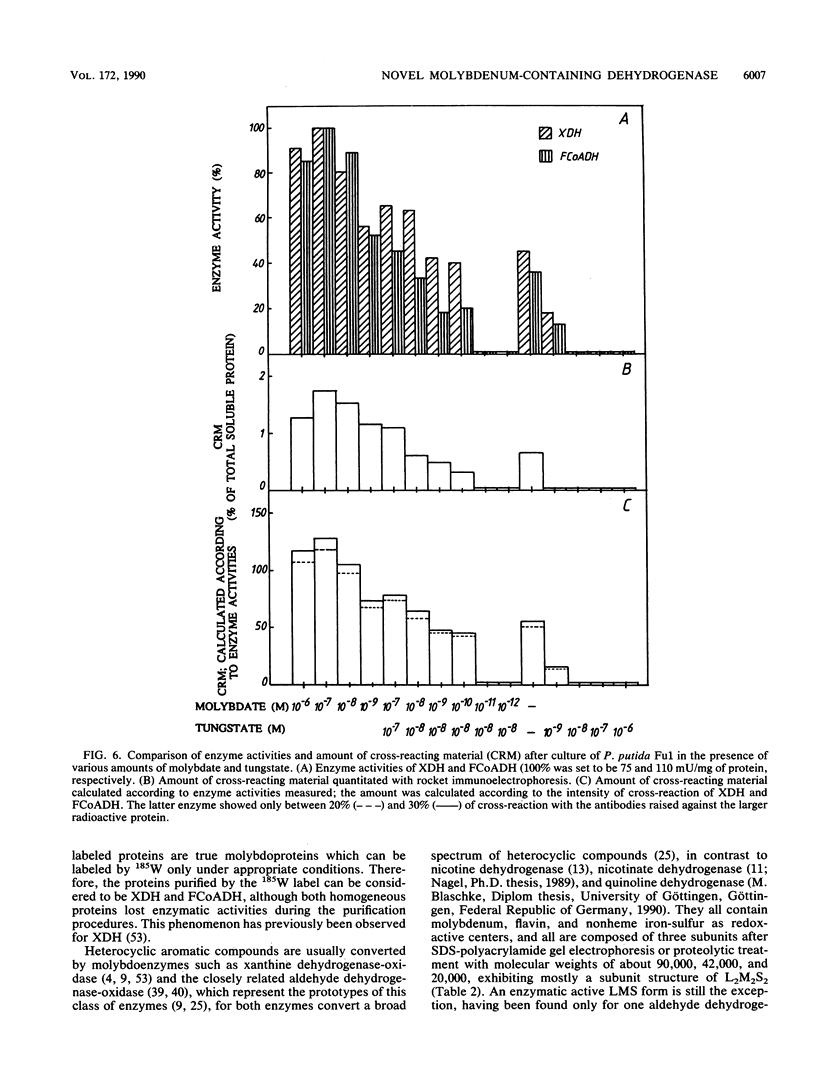
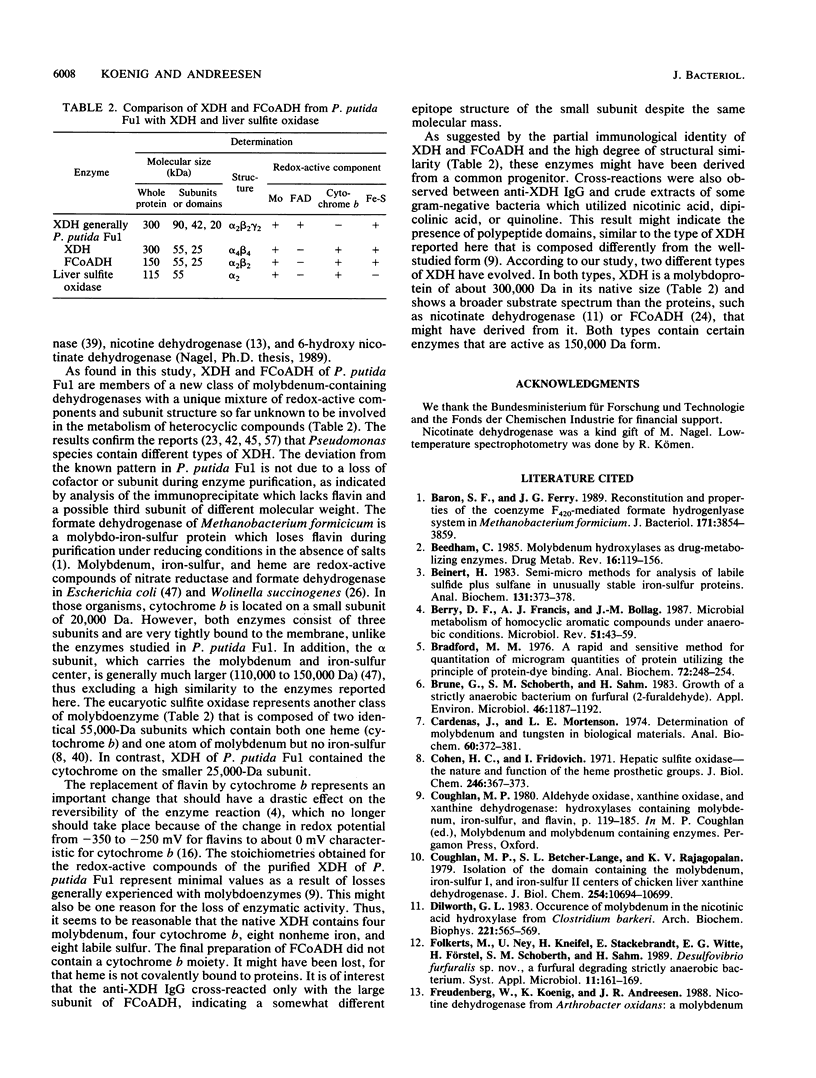
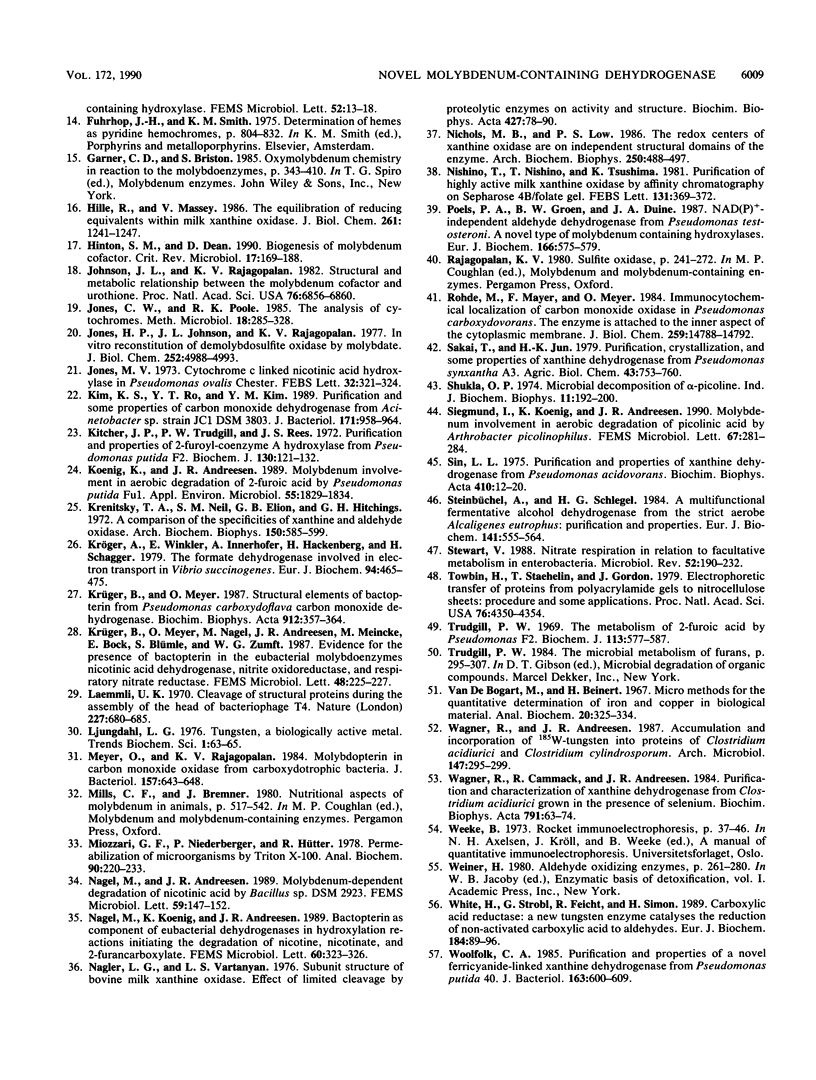
Images in this article
Selected References
These references are in PubMed. This may not be the complete list of references from this article.
- Baron S. F., Ferry J. G. Reconstitution and properties of a coenzyme F420-mediated formate hydrogenlyase system in Methanobacterium formicicum. J Bacteriol. 1989 Jul;171(7):3854–3859. doi: 10.1128/jb.171.7.3854-3859.1989. [DOI] [PMC free article] [PubMed] [Google Scholar]
- Beedham C. Molybdenum hydroxylases as drug-metabolizing enzymes. Drug Metab Rev. 1985;16(1-2):119–156. doi: 10.3109/03602538508991432. [DOI] [PubMed] [Google Scholar]
- Beinert H. Semi-micro methods for analysis of labile sulfide and of labile sulfide plus sulfane sulfur in unusually stable iron-sulfur proteins. Anal Biochem. 1983 Jun;131(2):373–378. doi: 10.1016/0003-2697(83)90186-0. [DOI] [PubMed] [Google Scholar]
- Berry D. F., Francis A. J., Bollag J. M. Microbial metabolism of homocyclic and heterocyclic aromatic compounds under anaerobic conditions. Microbiol Rev. 1987 Mar;51(1):43–59. doi: 10.1128/mr.51.1.43-59.1987. [DOI] [PMC free article] [PubMed] [Google Scholar]
- Bradford M. M. A rapid and sensitive method for the quantitation of microgram quantities of protein utilizing the principle of protein-dye binding. Anal Biochem. 1976 May 7;72:248–254. doi: 10.1016/0003-2697(76)90527-3. [DOI] [PubMed] [Google Scholar]
- Brune G., Schoberth S. M., Sahm H. Growth of a strictly anaerobic bacterium on furfural (2-furaldehyde). Appl Environ Microbiol. 1983 Nov;46(5):1187–1192. doi: 10.1128/aem.46.5.1187-1192.1983. [DOI] [PMC free article] [PubMed] [Google Scholar]
- Cardenas J., Mortenson L. E. Determination of molybdenum and tungsten in biological materials. Anal Biochem. 1974 Aug;60(2):372–381. doi: 10.1016/0003-2697(74)90244-9. [DOI] [PubMed] [Google Scholar]
- Cohen H. J., Fridovich I. Hepatic sulfite oxidase. The nature and function of the heme prosthetic groups. J Biol Chem. 1971 Jan 25;246(2):367–373. [PubMed] [Google Scholar]
- Coughlan M. P., Betcher-Lange S. L., Rajagopalan K. V. Isolation of the domain containing the molybdenum, iron-sulfur I, and iron-sulfur II centers of chicken liver xanthine dehydrogenase. J Biol Chem. 1979 Nov 10;254(21):10694–10699. [PubMed] [Google Scholar]
- Dilworth G. L. Occurrence of molybdenum in the nicotinic acid hydroxylase from Clostridium barkeri. Arch Biochem Biophys. 1983 Mar;221(2):565–569. doi: 10.1016/0003-9861(83)90176-5. [DOI] [PubMed] [Google Scholar]
- Hille R., Massey V. The equilibration of reducing equivalents within milk xanthine oxidase. J Biol Chem. 1986 Jan 25;261(3):1241–1247. [PubMed] [Google Scholar]
- Hinton S. M., Dean D. Biogenesis of molybdenum cofactors. Crit Rev Microbiol. 1990;17(3):169–188. doi: 10.3109/10408419009105724. [DOI] [PubMed] [Google Scholar]
- Johnson J. L., Rajagopalan K. V. Structural and metabolic relationship between the molybdenum cofactor and urothione. Proc Natl Acad Sci U S A. 1982 Nov;79(22):6856–6860. doi: 10.1073/pnas.79.22.6856. [DOI] [PMC free article] [PubMed] [Google Scholar]
- Jones H. P., Johnson J. L., Rajagopalan K. V. In vitro reconstitution of demolybdosulfite oxidase by molybdate. J Biol Chem. 1977 Jul 25;252(14):4988–4993. [PubMed] [Google Scholar]
- Jones M. V. Cytochrome c linked nicotinic acid hydroxylase in Pseudomonas ovalis Chester. FEBS Lett. 1973 Jun 1;32(2):321–324. doi: 10.1016/0014-5793(73)80864-6. [DOI] [PubMed] [Google Scholar]
- Kim K. S., Ro Y. T., Kim Y. M. Purification and some properties of carbon monoxide dehydrogenase from Acinetobacter sp. strain JC1 DSM 3803. J Bacteriol. 1989 Feb;171(2):958–964. doi: 10.1128/jb.171.2.958-964.1989. [DOI] [PMC free article] [PubMed] [Google Scholar]
- Kitcher J. P., Trudgill P. W., Rees J. S. Purification and properties of 2-furoyl-coenzyme A hydroxylase from Pseudomonas putida F2. Biochem J. 1972 Nov;130(1):121–132. doi: 10.1042/bj1300121. [DOI] [PMC free article] [PubMed] [Google Scholar]
- Koenig K., Andreesen J. R. Molybdenum Involvement in Aerobic Degradation of 2-Furoic Acid by Pseudomonas putida Fu1. Appl Environ Microbiol. 1989 Jul;55(7):1829–1834. doi: 10.1128/aem.55.7.1829-1834.1989. [DOI] [PMC free article] [PubMed] [Google Scholar]
- Krenitsky T. A., Neil S. M., Elion G. B., Hitchings G. H. A comparison of the specificities of xanthine oxidase and aldehyde oxidase. Arch Biochem Biophys. 1972 Jun;150(2):585–599. doi: 10.1016/0003-9861(72)90078-1. [DOI] [PubMed] [Google Scholar]
- Kröger A., Winkler E., Innerhofer A., Hackenberg H., Schägger H. The formate dehydrogenase involved in electron transport from formate to fumarate in Vibrio succinogenes. Eur J Biochem. 1979 Mar;94(2):465–475. doi: 10.1111/j.1432-1033.1979.tb12914.x. [DOI] [PubMed] [Google Scholar]
- Krüger B., Meyer O. Structural elements of bactopterin from Pseudomonas carboxydoflava carbon monoxide dehydrogenase. Biochim Biophys Acta. 1987 Apr 30;912(3):357–364. doi: 10.1016/0167-4838(87)90040-9. [DOI] [PubMed] [Google Scholar]
- Laemmli U. K. Cleavage of structural proteins during the assembly of the head of bacteriophage T4. Nature. 1970 Aug 15;227(5259):680–685. doi: 10.1038/227680a0. [DOI] [PubMed] [Google Scholar]
- Meyer O., Rajagopalan K. V. Molybdopterin in carbon monoxide oxidase from carboxydotrophic bacteria. J Bacteriol. 1984 Feb;157(2):643–648. doi: 10.1128/jb.157.2.643-648.1984. [DOI] [PMC free article] [PubMed] [Google Scholar]
- Miozzari G. F., Niederberger P., Hütter R. Permeabilization of microorganisms by Triton X-100. Anal Biochem. 1978 Oct 1;90(1):220–233. doi: 10.1016/0003-2697(78)90026-x. [DOI] [PubMed] [Google Scholar]
- Nagler L. G., Vartanyan L. S. Subunit structure of bovine milk xanthine oxidase. Effect of limited cleavage by proteolytic enzymes on activity and structure. Biochim Biophys Acta. 1976 Mar 18;427(1):78–90. doi: 10.1016/0005-2795(76)90287-7. [DOI] [PubMed] [Google Scholar]
- Nichols M. B., Low P. S. The redox centers of xanthine oxidase are on independent structural domains of the enzyme. Arch Biochem Biophys. 1986 Nov 1;250(2):488–497. doi: 10.1016/0003-9861(86)90753-8. [DOI] [PubMed] [Google Scholar]
- Nishino T., Nishino T., Tsushima K. Purification of highly active milk xanthine oxidase by affinity chromatography on Sepharose 4B/folate gel. FEBS Lett. 1981 Aug 31;131(2):369–372. doi: 10.1016/0014-5793(81)80406-1. [DOI] [PubMed] [Google Scholar]
- Poels P. A., Groen B. W., Duine J. A. NAD(P)+-independent aldehyde dehydrogenase from Pseudomonas testosteroni. A novel type of molybdenum-containing hydroxylase. Eur J Biochem. 1987 Aug 3;166(3):575–579. doi: 10.1111/j.1432-1033.1987.tb13552.x. [DOI] [PubMed] [Google Scholar]
- Rohde M., Mayer F., Meyer O. Immunocytochemical localization of carbon monoxide oxidase in Pseudomonas carboxydovorans. The enzyme is attached to the inner aspect of the cytoplasmic membrane. J Biol Chem. 1984 Dec 10;259(23):14788–14792. [PubMed] [Google Scholar]
- Shukla O. P. Microbial Decomposition of alpha-Picoline. Indian J Biochem Biophys. 1974 Sep;11(3):192–200. [PubMed] [Google Scholar]
- Sin I. L. Purification and properties of xanthine dehydrogenase from Pseudomonas acidovorans. Biochim Biophys Acta. 1975 Nov 20;410(1):12–20. doi: 10.1016/0005-2744(75)90203-x. [DOI] [PubMed] [Google Scholar]
- Steinbüchel A., Schlegel H. G. A multifunctional fermentative alcohol dehydrogenase from the strict aerobe Alcaligenes eutrophus: purification and properties. Eur J Biochem. 1984 Jun 15;141(3):555–564. doi: 10.1111/j.1432-1033.1984.tb08229.x. [DOI] [PubMed] [Google Scholar]
- Stewart V. Nitrate respiration in relation to facultative metabolism in enterobacteria. Microbiol Rev. 1988 Jun;52(2):190–232. doi: 10.1128/mr.52.2.190-232.1988. [DOI] [PMC free article] [PubMed] [Google Scholar]
- Towbin H., Staehelin T., Gordon J. Electrophoretic transfer of proteins from polyacrylamide gels to nitrocellulose sheets: procedure and some applications. Proc Natl Acad Sci U S A. 1979 Sep;76(9):4350–4354. doi: 10.1073/pnas.76.9.4350. [DOI] [PMC free article] [PubMed] [Google Scholar]
- Trudgill P. W. The metabolism of 2-furoic acid by Pseudomanas F2. Biochem J. 1969 Jul;113(4):577–587. doi: 10.1042/bj1130577. [DOI] [PMC free article] [PubMed] [Google Scholar]
- Van de Bogart M., Beinert H. Micro methods for the quantitative determination of iron and copper in biological material. Anal Biochem. 1967 Aug;20(2):325–334. doi: 10.1016/0003-2697(67)90038-3. [DOI] [PubMed] [Google Scholar]
- Weeke B. Rocket immunoelectrophoresis. Scand J Immunol Suppl. 1973;1:37–46. doi: 10.1111/j.1365-3083.1973.tb03777.x. [DOI] [PubMed] [Google Scholar]
- White H., Strobl G., Feicht R., Simon H. Carboxylic acid reductase: a new tungsten enzyme catalyses the reduction of non-activated carboxylic acids to aldehydes. Eur J Biochem. 1989 Sep 1;184(1):89–96. doi: 10.1111/j.1432-1033.1989.tb14993.x. [DOI] [PubMed] [Google Scholar]
- Woolfolk C. A. Purification and properties of a novel ferricyanide-linked xanthine dehydrogenase from Pseudomonas putida 40. J Bacteriol. 1985 Aug;163(2):600–609. doi: 10.1128/jb.163.2.600-609.1985. [DOI] [PMC free article] [PubMed] [Google Scholar]