Abstract
Different alleles undergoing strong symmetric balancing selection show a simple genealogical structure (allelic genealogy), similar to the gene genealogy described by the coalescence process for a sample of neutral genes randomly drawn from a panmictic population at equilibrium. The only difference between the two genealogies lies in the different time scales. An approximate scaling factor for allelic genealogy relative to that of neutral gene genealogy is [square root of S/(2M)].[In[S/(16 pi M2)]]-3/2, where M = Nu and S = 2Ns (N, effective population size; u, mutation rate to selected alleles per locus per generation; s, selection coefficient). The larger the value of square root of S/M (greater than or equal to 100), the larger the scaling factor. These findings, supported by simulation results, allow one to apply the theoretical results of the coalescence process directly to the allelic genealogy. Combined with the trans-species evolution of the major histocompatibility complex polymorphism for which balancing selection is believed to be responsible, allelic genealogy predicts that the number of breeding individuals in the human population could not be as small as 50-100 at any time of its evolutionary history. The analysis appears to contradict the founder principle as being important in recent mammalian evolution.
Full text
PDF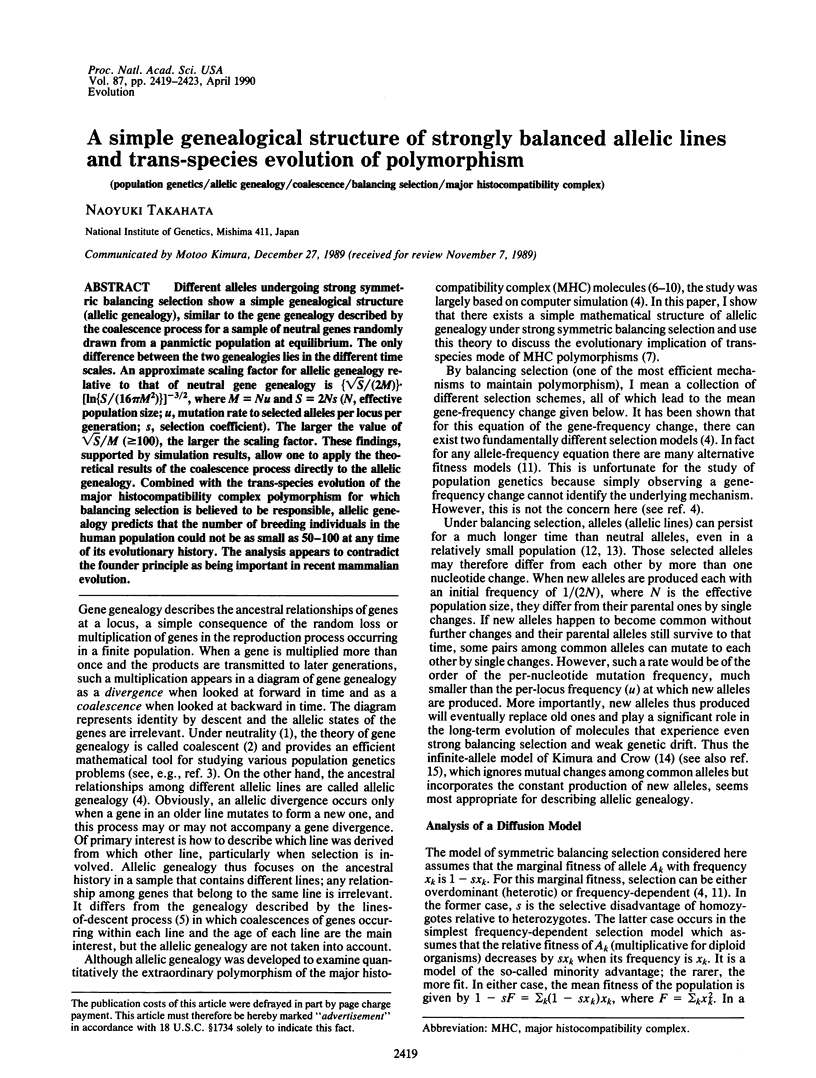
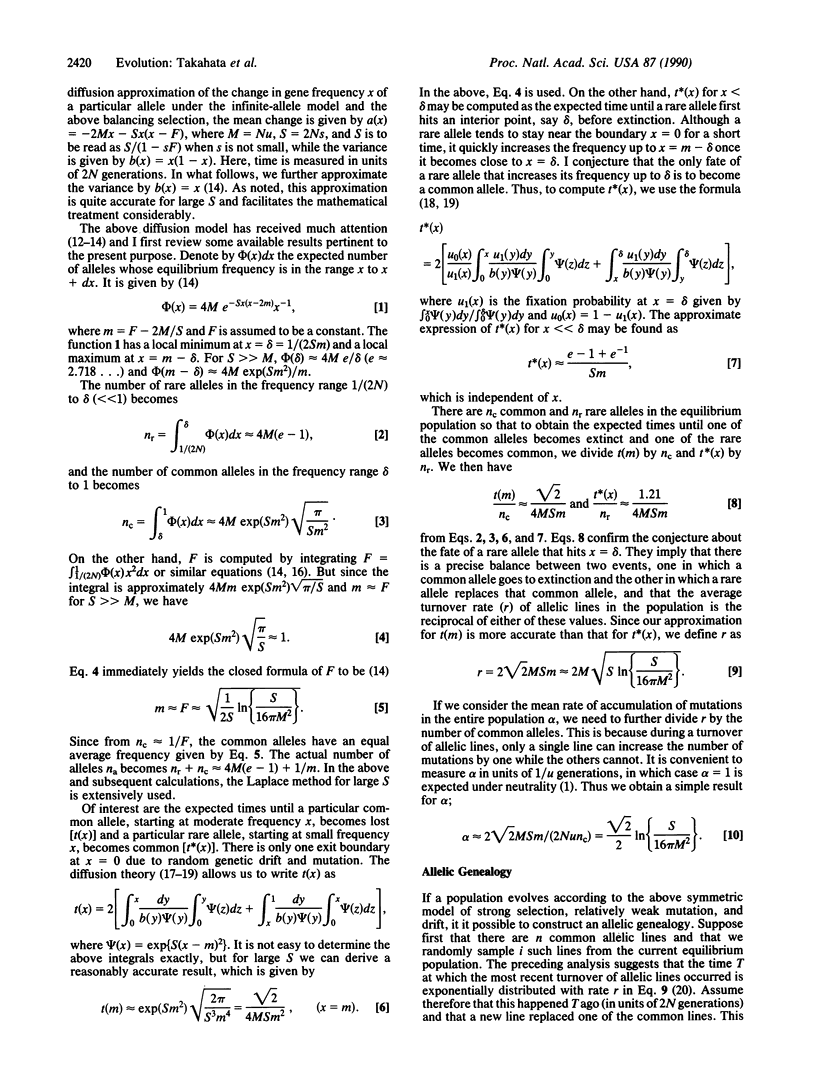
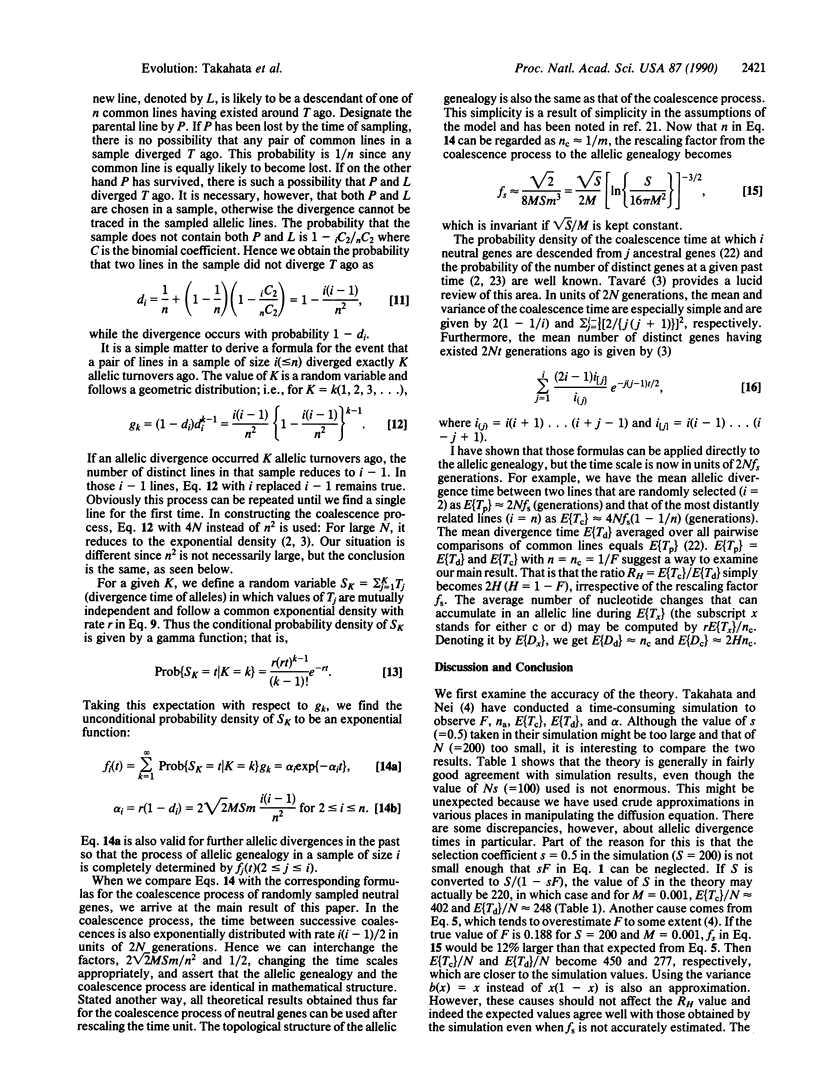
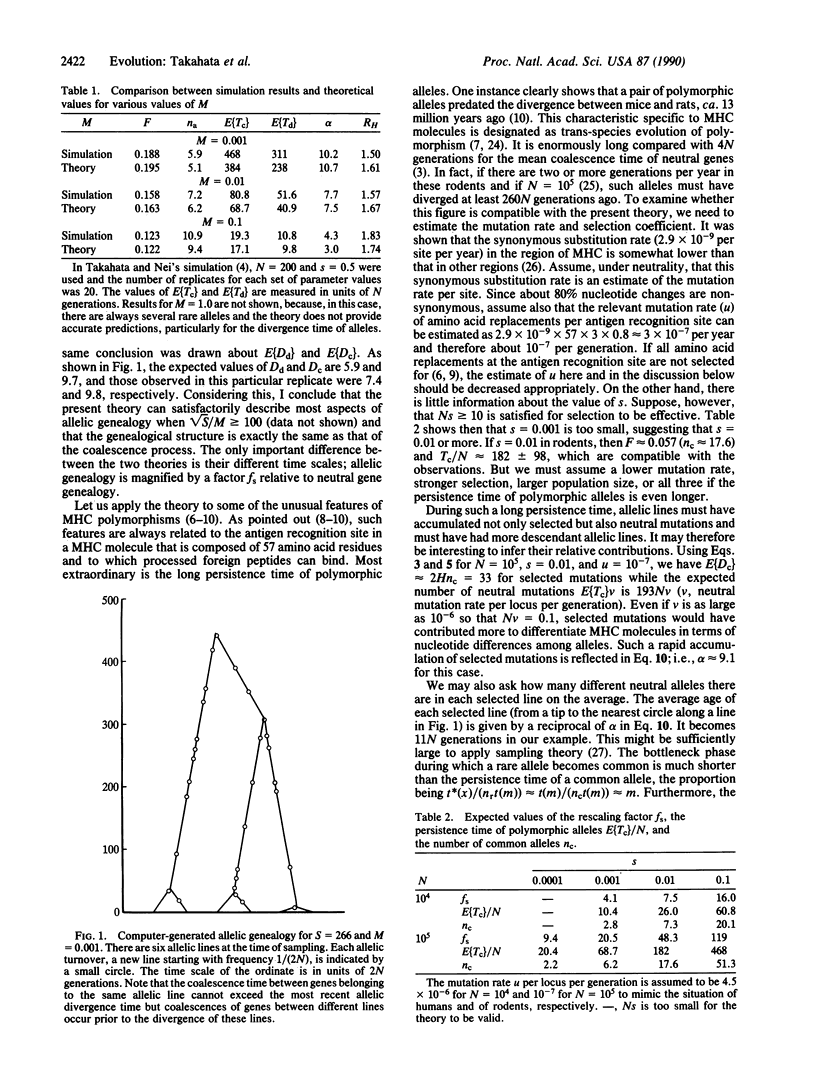
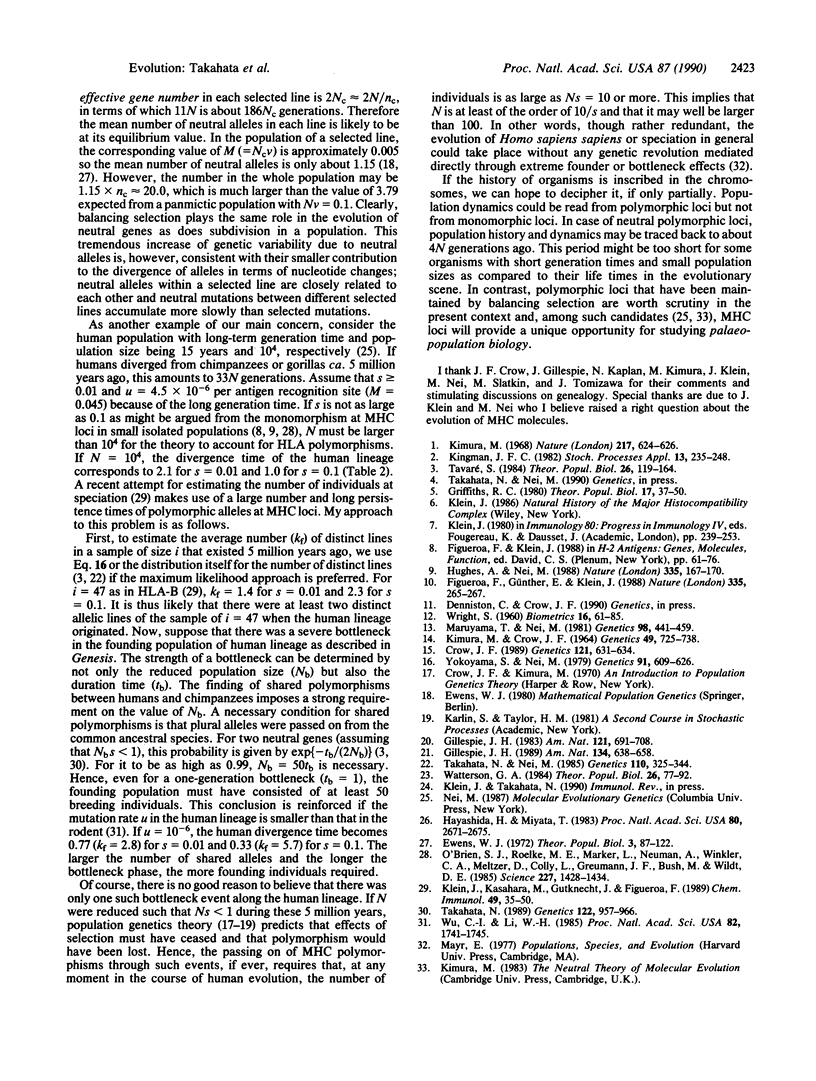
Selected References
These references are in PubMed. This may not be the complete list of references from this article.
- Crow J. F. Twenty-five years ago in genetics: the infinite allele model. Genetics. 1989 Apr;121(4):631–634. doi: 10.1093/genetics/121.4.631. [DOI] [PMC free article] [PubMed] [Google Scholar]
- Ewens W. J. The sampling theory of selectively neutral alleles. Theor Popul Biol. 1972 Mar;3(1):87–112. doi: 10.1016/0040-5809(72)90035-4. [DOI] [PubMed] [Google Scholar]
- Figueroa F., Günther E., Klein J. MHC polymorphism pre-dating speciation. Nature. 1988 Sep 15;335(6187):265–267. doi: 10.1038/335265a0. [DOI] [PubMed] [Google Scholar]
- Griffiths R. C. Lines of descent in the diffusion approximation of neutral Wright-Fisher models. Theor Popul Biol. 1980 Feb;17(1):37–50. doi: 10.1016/0040-5809(80)90013-1. [DOI] [PubMed] [Google Scholar]
- Hayashida H., Miyata T. Unusual evolutionary conservation and frequent DNA segment exchange in class I genes of the major histocompatibility complex. Proc Natl Acad Sci U S A. 1983 May;80(9):2671–2675. doi: 10.1073/pnas.80.9.2671. [DOI] [PMC free article] [PubMed] [Google Scholar]
- Hughes A. L., Nei M. Pattern of nucleotide substitution at major histocompatibility complex class I loci reveals overdominant selection. Nature. 1988 Sep 8;335(6186):167–170. doi: 10.1038/335167a0. [DOI] [PubMed] [Google Scholar]
- KIMURA M., CROW J. F. THE NUMBER OF ALLELES THAT CAN BE MAINTAINED IN A FINITE POPULATION. Genetics. 1964 Apr;49:725–738. doi: 10.1093/genetics/49.4.725. [DOI] [PMC free article] [PubMed] [Google Scholar]
- Kimura M. Evolutionary rate at the molecular level. Nature. 1968 Feb 17;217(5129):624–626. doi: 10.1038/217624a0. [DOI] [PubMed] [Google Scholar]
- Klein J., Kasahara M., Gutknecht J., Figueroa F. Origin and function of Mhc polymorphism. Chem Immunol. 1990;49:35–50. [PubMed] [Google Scholar]
- Maruyama T., Nei M. Genetic variability maintained by mutation and overdominant selection in finite populations. Genetics. 1981 Jun;98(2):441–459. doi: 10.1093/genetics/98.2.441. [DOI] [PMC free article] [PubMed] [Google Scholar]
- O'Brien S. J., Roelke M. E., Marker L., Newman A., Winkler C. A., Meltzer D., Colly L., Evermann J. F., Bush M., Wildt D. E. Genetic basis for species vulnerability in the cheetah. Science. 1985 Mar 22;227(4693):1428–1434. doi: 10.1126/science.2983425. [DOI] [PubMed] [Google Scholar]
- Takahata N. Gene genealogy in three related populations: consistency probability between gene and population trees. Genetics. 1989 Aug;122(4):957–966. doi: 10.1093/genetics/122.4.957. [DOI] [PMC free article] [PubMed] [Google Scholar]
- Takahata N., Nei M. Gene genealogy and variance of interpopulational nucleotide differences. Genetics. 1985 Jun;110(2):325–344. doi: 10.1093/genetics/110.2.325. [DOI] [PMC free article] [PubMed] [Google Scholar]
- Tavaré S. Line-of-descent and genealogical processes, and their applications in population genetics models. Theor Popul Biol. 1984 Oct;26(2):119–164. doi: 10.1016/0040-5809(84)90027-3. [DOI] [PubMed] [Google Scholar]
- Wu C. I., Li W. H. Evidence for higher rates of nucleotide substitution in rodents than in man. Proc Natl Acad Sci U S A. 1985 Mar;82(6):1741–1745. doi: 10.1073/pnas.82.6.1741. [DOI] [PMC free article] [PubMed] [Google Scholar]
- Yokoyama S., Nei M. Population dynamics of sex-determining alleles in honey bees and self-incompatibility alleles in plants. Genetics. 1979 Mar;91(3):609–626. doi: 10.1093/genetics/91.3.609. [DOI] [PMC free article] [PubMed] [Google Scholar]