Abstract
The D-Ala-D-Ala carboxypeptidases/transpeptidases (penicillin-binding proteins, PBPs) share considerable structural homology with class A beta-lactamases (EC 3.5.2.6), although these beta-lactamases have no observable D-Ala-D-Ala carboxypeptidase activity. With the objective of recruiting such activity into a beta-lactamase background, we have prepared a chimeric protein by inserting a 28-amino acid segment of PBP-5 of Escherichia coli in place of the corresponding region of the RTEM-1 beta-lactamase. The segment thus inserted encompasses two residues conserved in both families: Ser-70, which forms the acyl-enzyme intermediate during beta-lactam hydrolysis, and Lys-73, whose presence has been shown to be necessary for catalysis. This chimera involves changes of 18 residues and gives a protein that differs at 7% of the residues from the parent. Whereas RTEM beta-lactamase has no D-Ala-D-Ala carboxypeptidase activity, that of the chimera is significant and is, in fact, about 1% the activity of PBP-5 on diacetyl-L-Lys-D-Ala-D-Ala; in terms of free energy of activation, the chimera stabilizes the transition state for the reaction to within about 2.7 kcal/mol of the stabilization achieved by PBP-5. Furthermore, the chimera catalyzes hydrolysis exclusively at the carboxyl-terminal amide bond which is the site of cleavage by D-Ala-D-Ala carboxypeptidase. Though containing all those residues that are conserved throughout class A beta-lactamases and are thought to be essential for beta-lactamase activity, the chimera has considerably reduced activity (approximately 10(-5) on penams such as penicillins and ampicillins as substrates. As a catalyst, the chimera shows an induction period of approximately 30 min, reflecting a slow conformational rearrangement from an inactive precursor to the active enzyme.
Full text
PDF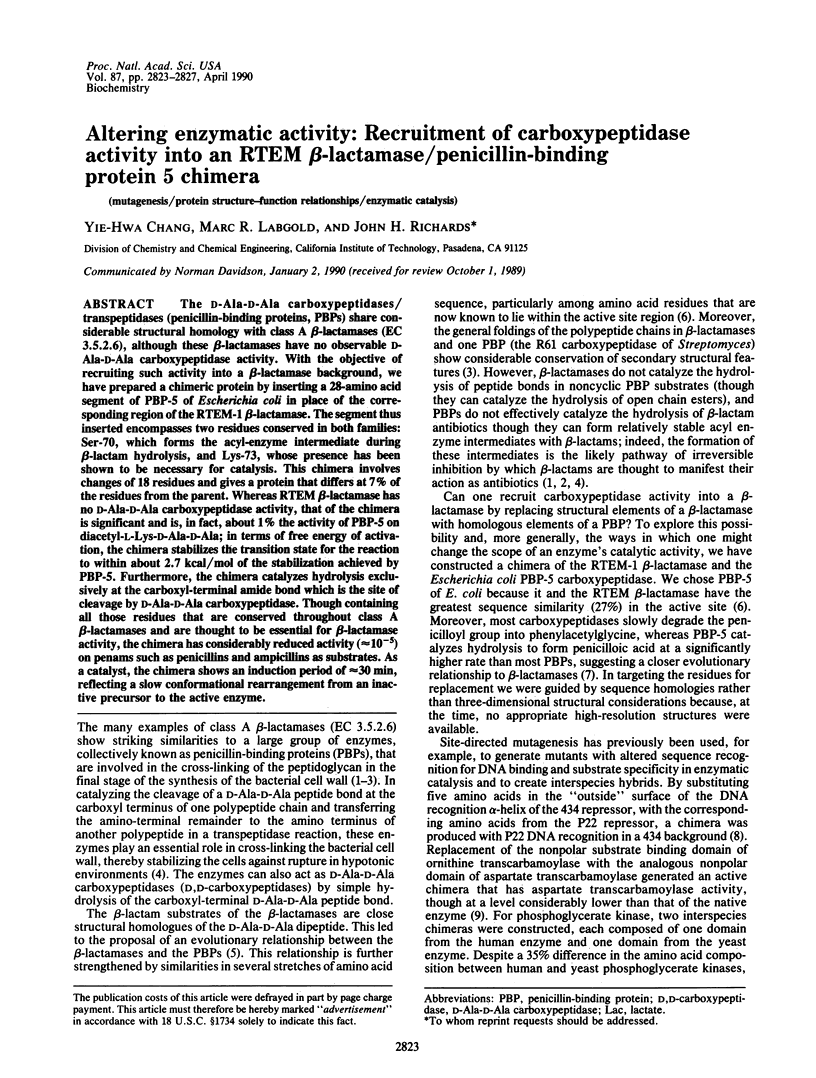
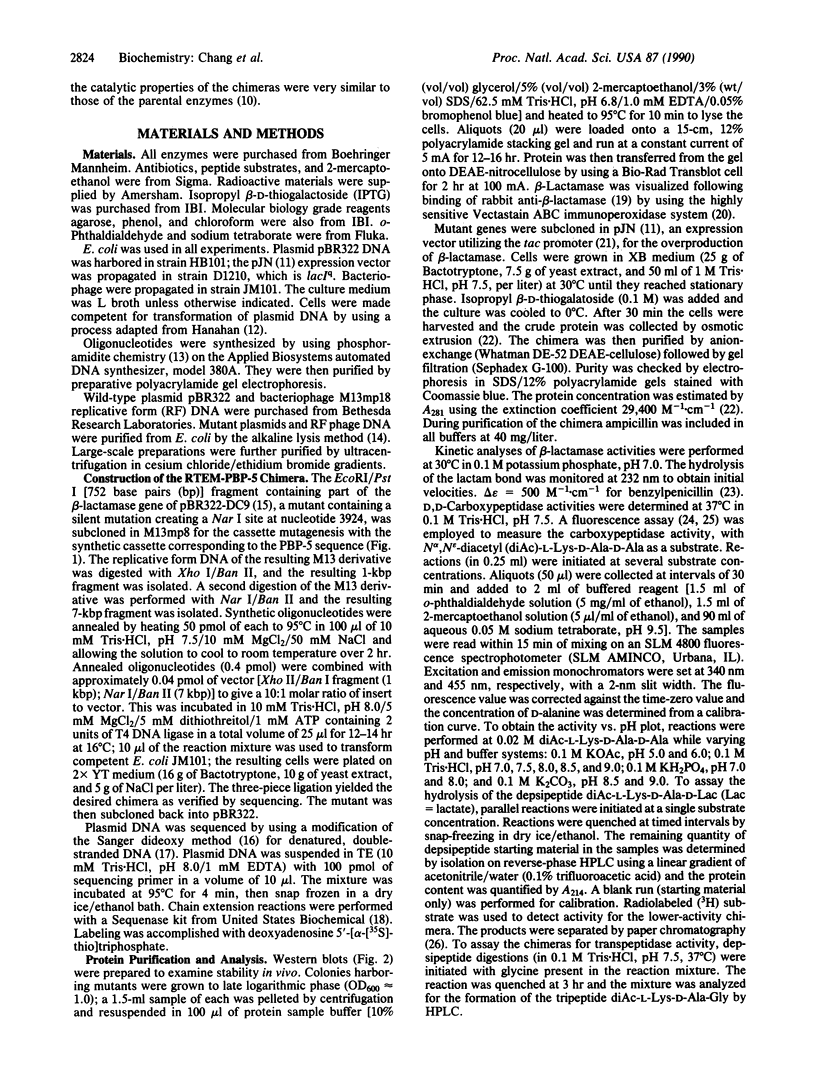
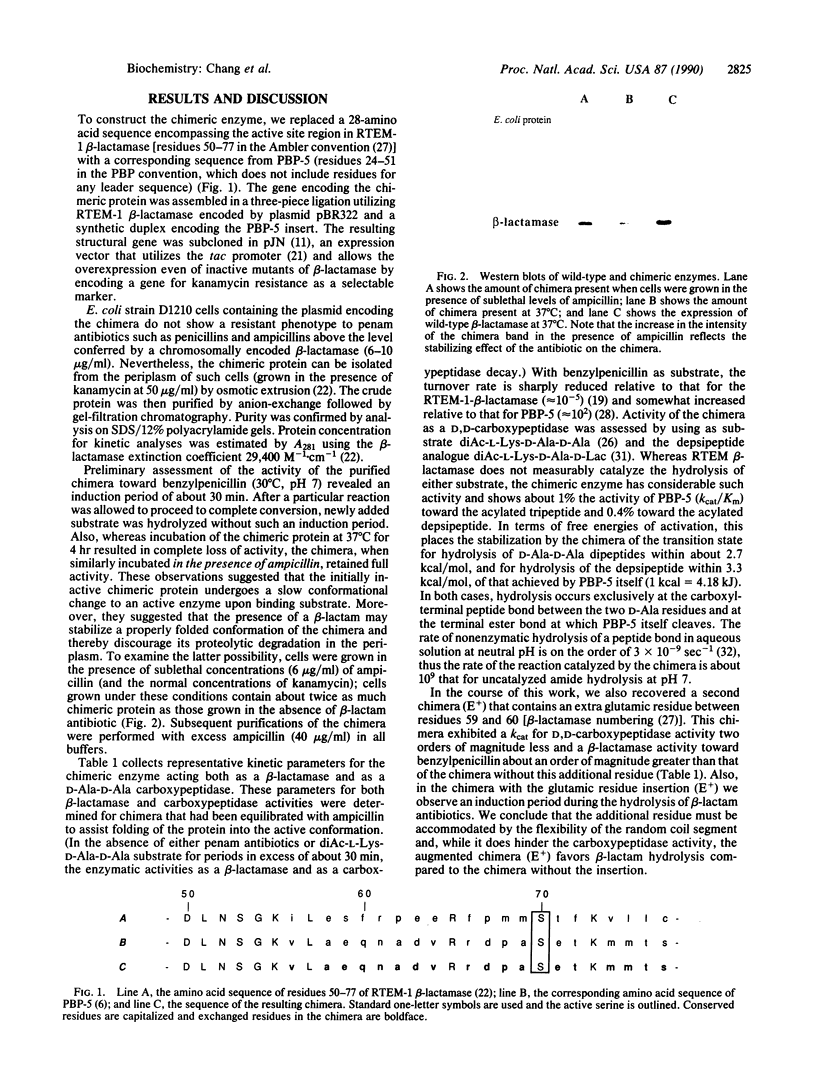
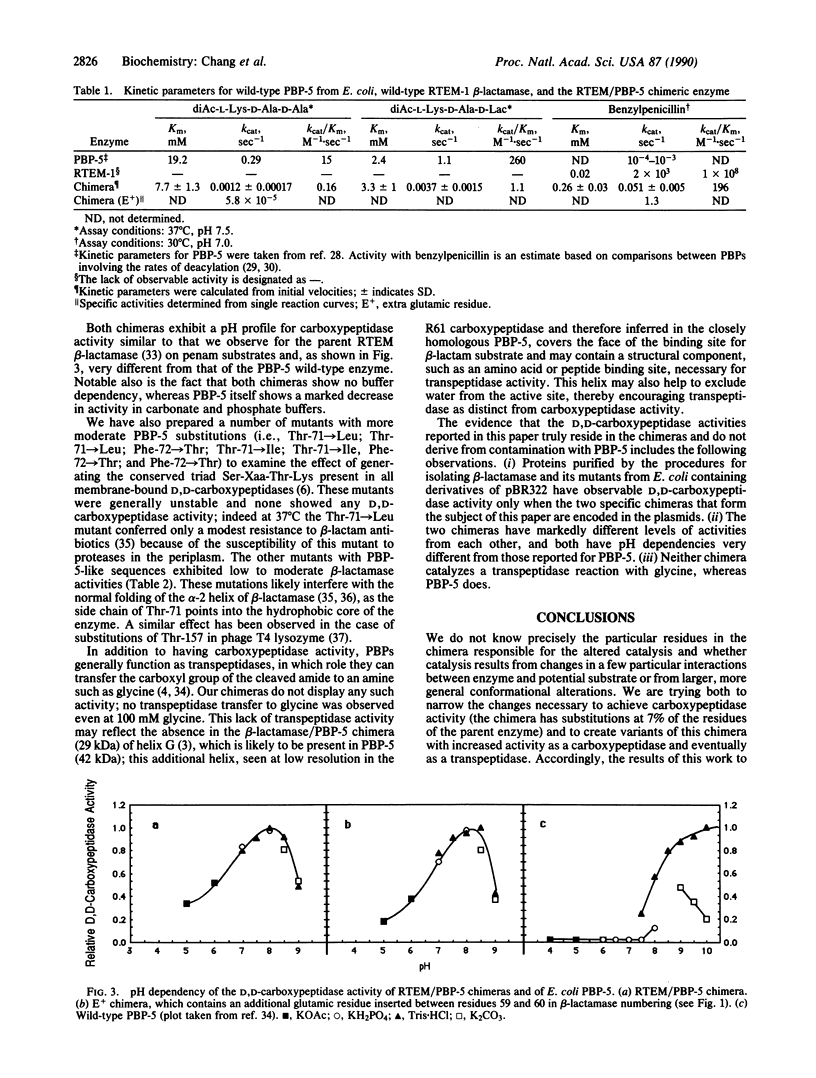
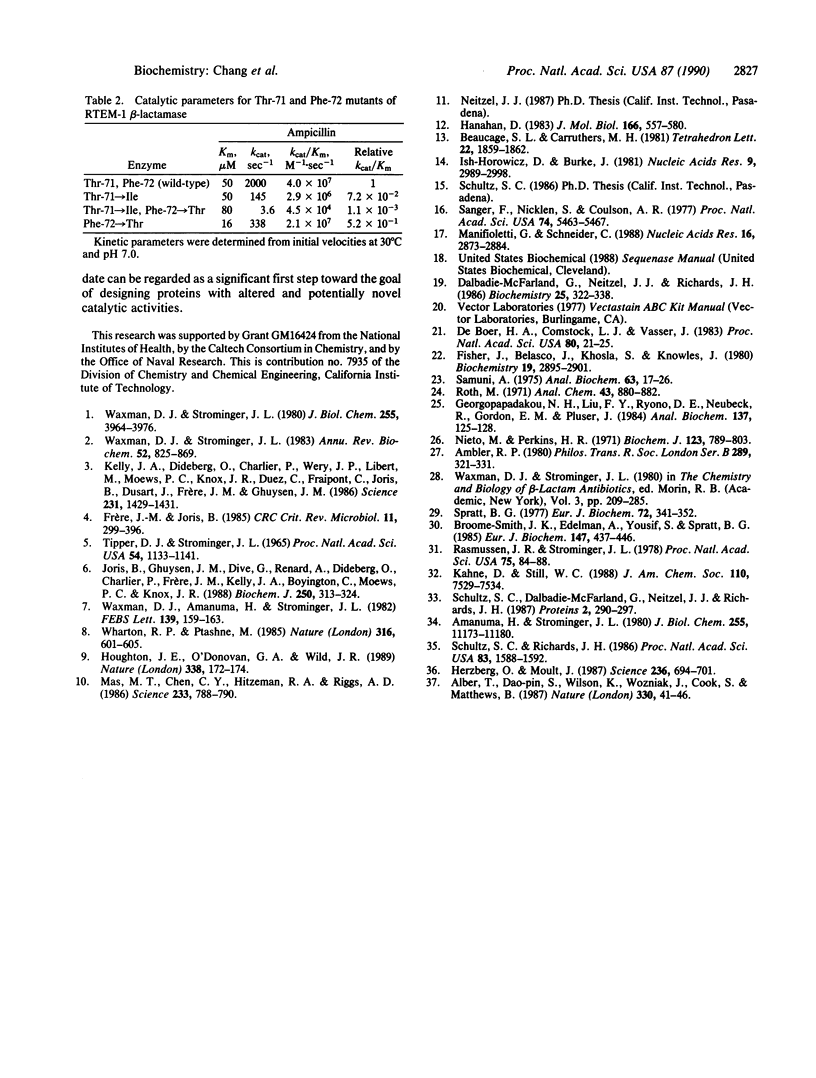
Images in this article
Selected References
These references are in PubMed. This may not be the complete list of references from this article.
- Alber T., Sun D. P., Wilson K., Wozniak J. A., Cook S. P., Matthews B. W. Contributions of hydrogen bonds of Thr 157 to the thermodynamic stability of phage T4 lysozyme. Nature. 1987 Nov 5;330(6143):41–46. doi: 10.1038/330041a0. [DOI] [PubMed] [Google Scholar]
- Amanuma H., Strominger J. L. Purification and properties of penicillin-binding proteins 5 and 6 from Escherichia coli membranes. J Biol Chem. 1980 Dec 10;255(23):11173–11180. [PubMed] [Google Scholar]
- Ambler R. P. The structure of beta-lactamases. Philos Trans R Soc Lond B Biol Sci. 1980 May 16;289(1036):321–331. doi: 10.1098/rstb.1980.0049. [DOI] [PubMed] [Google Scholar]
- Broome-Smith J. K., Edelman A., Yousif S., Spratt B. G. The nucleotide sequences of the ponA and ponB genes encoding penicillin-binding protein 1A and 1B of Escherichia coli K12. Eur J Biochem. 1985 Mar 1;147(2):437–446. doi: 10.1111/j.1432-1033.1985.tb08768.x. [DOI] [PubMed] [Google Scholar]
- Dalbadie-McFarland G., Neitzel J. J., Richards J. H. Active-site mutants of beta-lactamase: use of an inactive double mutant to study requirements for catalysis. Biochemistry. 1986 Jan 28;25(2):332–338. doi: 10.1021/bi00350a008. [DOI] [PubMed] [Google Scholar]
- Fisher J., Belasco J. G., Khosla S., Knowles J. R. beta-Lactamase proceeds via an acyl-enzyme intermediate. Interaction of the Escherichia coli RTEM enzyme with cefoxitin. Biochemistry. 1980 Jun 24;19(13):2895–2901. doi: 10.1021/bi00554a012. [DOI] [PubMed] [Google Scholar]
- Frère J. M., Joris B. Penicillin-sensitive enzymes in peptidoglycan biosynthesis. Crit Rev Microbiol. 1985;11(4):299–396. doi: 10.3109/10408418409105906. [DOI] [PubMed] [Google Scholar]
- Georgopapadakou N. H., Liu F. Y., Ryono D. E., Neubeck R., Gordon E. M., Pluscec J. Streptomyces R61 DD-carboxypeptidase: hydrolysis of X-D-alanyl-D-alanine peptides measured by a fluorometric assay. Anal Biochem. 1984 Feb;137(1):125–128. doi: 10.1016/0003-2697(84)90357-9. [DOI] [PubMed] [Google Scholar]
- Hanahan D. Studies on transformation of Escherichia coli with plasmids. J Mol Biol. 1983 Jun 5;166(4):557–580. doi: 10.1016/s0022-2836(83)80284-8. [DOI] [PubMed] [Google Scholar]
- Herzberg O., Moult J. Bacterial resistance to beta-lactam antibiotics: crystal structure of beta-lactamase from Staphylococcus aureus PC1 at 2.5 A resolution. Science. 1987 May 8;236(4802):694–701. doi: 10.1126/science.3107125. [DOI] [PubMed] [Google Scholar]
- Houghton J. E., O'Donovan G. A., Wild J. R. Reconstruction of an enzyme by domain substitution effectively switches substrate specificity. Nature. 1989 Mar 9;338(6211):172–174. doi: 10.1038/338172a0. [DOI] [PubMed] [Google Scholar]
- Ish-Horowicz D., Burke J. F. Rapid and efficient cosmid cloning. Nucleic Acids Res. 1981 Jul 10;9(13):2989–2998. doi: 10.1093/nar/9.13.2989. [DOI] [PMC free article] [PubMed] [Google Scholar]
- Joris B., Ghuysen J. M., Dive G., Renard A., Dideberg O., Charlier P., Frère J. M., Kelly J. A., Boyington J. C., Moews P. C. The active-site-serine penicillin-recognizing enzymes as members of the Streptomyces R61 DD-peptidase family. Biochem J. 1988 Mar 1;250(2):313–324. doi: 10.1042/bj2500313. [DOI] [PMC free article] [PubMed] [Google Scholar]
- Kelly J. A., Dideberg O., Charlier P., Wery J. P., Libert M., Moews P. C., Knox J. R., Duez C., Fraipont C., Joris B. On the origin of bacterial resistance to penicillin: comparison of a beta-lactamase and a penicillin target. Science. 1986 Mar 21;231(4744):1429–1431. doi: 10.1126/science.3082007. [DOI] [PubMed] [Google Scholar]
- Manfioletti G., Schneider C. A new and fast method for preparing high quality lambda DNA suitable for sequencing. Nucleic Acids Res. 1988 Apr 11;16(7):2873–2884. doi: 10.1093/nar/16.7.2873. [DOI] [PMC free article] [PubMed] [Google Scholar]
- Mas M. T., Chen C. Y., Hitzeman R. A., Riggs A. D. Active human-yeast chimeric phosphoglycerate kinases engineered by domain interchange. Science. 1986 Aug 15;233(4765):788–790. doi: 10.1126/science.3526552. [DOI] [PubMed] [Google Scholar]
- Nieto M., Perkins H. R. Modifications of the acyl-D-alanyl-D-alanine terminus affecting complex-formation with vancomycin. Biochem J. 1971 Aug;123(5):789–803. doi: 10.1042/bj1230789. [DOI] [PMC free article] [PubMed] [Google Scholar]
- Rasmussen J. R., Strominger J. L. Utilization of a depsipeptide substrate for trapping acyl-enzyme intermediates of penicillin-sensitive D-alanine carboxypeptidases. Proc Natl Acad Sci U S A. 1978 Jan;75(1):84–88. doi: 10.1073/pnas.75.1.84. [DOI] [PMC free article] [PubMed] [Google Scholar]
- Roth M. Fluorescence reaction for amino acids. Anal Chem. 1971 Jun;43(7):880–882. doi: 10.1021/ac60302a020. [DOI] [PubMed] [Google Scholar]
- Samuni A. A direct spectrophotometric assay and determination of Michaelis constants for the beta-lactamase reaction. Anal Biochem. 1975 Jan;63(1):17–26. doi: 10.1016/0003-2697(75)90185-2. [DOI] [PubMed] [Google Scholar]
- Sanger F., Nicklen S., Coulson A. R. DNA sequencing with chain-terminating inhibitors. Proc Natl Acad Sci U S A. 1977 Dec;74(12):5463–5467. doi: 10.1073/pnas.74.12.5463. [DOI] [PMC free article] [PubMed] [Google Scholar]
- Schultz S. C., Dalbadie-McFarland G., Neitzel J. J., Richards J. H. Stability of wild-type and mutant RTEM-1 beta-lactamases: effect of the disulfide bond. Proteins. 1987;2(4):290–297. doi: 10.1002/prot.340020405. [DOI] [PubMed] [Google Scholar]
- Schultz S. C., Richards J. H. Site-saturation studies of beta-lactamase: production and characterization of mutant beta-lactamases with all possible amino acid substitutions at residue 71. Proc Natl Acad Sci U S A. 1986 Mar;83(6):1588–1592. doi: 10.1073/pnas.83.6.1588. [DOI] [PMC free article] [PubMed] [Google Scholar]
- Spratt B. G. Properties of the penicillin-binding proteins of Escherichia coli K12,. Eur J Biochem. 1977 Jan;72(2):341–352. doi: 10.1111/j.1432-1033.1977.tb11258.x. [DOI] [PubMed] [Google Scholar]
- Tipper D. J., Strominger J. L. Mechanism of action of penicillins: a proposal based on their structural similarity to acyl-D-alanyl-D-alanine. Proc Natl Acad Sci U S A. 1965 Oct;54(4):1133–1141. doi: 10.1073/pnas.54.4.1133. [DOI] [PMC free article] [PubMed] [Google Scholar]
- Waxman D. J., Amanuma H., Strominger J. L. Amino acid sequence homologies between Escherichia coli penicillin-binding protein 5 and class A beta-lactamases. FEBS Lett. 1982 Mar 22;139(2):159–163. doi: 10.1016/0014-5793(82)80840-5. [DOI] [PubMed] [Google Scholar]
- Waxman D. J., Strominger J. L. Penicillin-binding proteins and the mechanism of action of beta-lactam antibiotics. Annu Rev Biochem. 1983;52:825–869. doi: 10.1146/annurev.bi.52.070183.004141. [DOI] [PubMed] [Google Scholar]
- Waxman D. J., Strominger J. L. Sequence of active site peptides from the penicillin-sensitive D-alanine carboxypeptidase of Bacillus subtilis. Mechanism of penicillin action and sequence homology to beta-lactamases. J Biol Chem. 1980 May 10;255(9):3964–3976. [PubMed] [Google Scholar]
- Wharton R. P., Ptashne M. Changing the binding specificity of a repressor by redesigning an alpha-helix. Nature. 1985 Aug 15;316(6029):601–605. doi: 10.1038/316601a0. [DOI] [PubMed] [Google Scholar]
- de Boer H. A., Comstock L. J., Vasser M. The tac promoter: a functional hybrid derived from the trp and lac promoters. Proc Natl Acad Sci U S A. 1983 Jan;80(1):21–25. doi: 10.1073/pnas.80.1.21. [DOI] [PMC free article] [PubMed] [Google Scholar]