Abstract
An attempt was made to evaluate Münch's hypothesis of osmotically generated pressure flow in soybean (Glycine max L.) sieve tubes from velocity measurements and calculations of pressure potentials and sieve tube resistances. Pressure potential was estimated from values for water potentials and osmotic potential. Leaf water potential measurements were made by isopiestic thermocouple psychrometry, while the water potential of the nutrient solution was made with a vapor pressure osmometer. Osmotic potential was measured by first bringing the sucrose pools in the entire plant to the same specific radioactivity by steady-state-labeling of the shoot with constant specific radioactivity 14CO2 for 5 to 8 hours. Sucrose concentrations in sieve tubes were calculated from the disintegration rate per unit volume in sieve elements as measured by absolute quantitative microautoradiography of freeze-substituted, Eponembedded source (leaf) and sink (root) tissues.
Conductivity of the sieve tubes was calculated from measurements of their dimensions in the petiole, stem, and root. The total pressure drop required for pressure flow at the observed velocities was calculated from the conductivity, velocity, and path length.
In all experiments, the calculated sucrose concentration in source sieve tubes was greater than that in sink sieve tubes, with an average ratio (source to sink) of 1.79:1. However, the absolute sucrose concentrations (average values of 46.4 mg cm-3 in the source and 23.9 mg cm-3 in the sink) would have been insufficient to maintain positive turgor in the sieve elements, and the expected pressure differences would not have accounted for movement at the observed velocities. However, the low values for sucrose concentrations almost certainly were due to loss of sucrose during tissue preparation but, for technical reasons, such loss could not be accurately quantified.
Assuming a sucrose concentration sufficient to maintain zero turgor in the root sieve tubes, a xylem water potential gradient (ψw [sink] - ψw [source]) of 2 bars between source and sink, and the measured ratio of sucrose concentrations in source and sink (1.79:1), the average turgor gradient between source and sink (ψp [sink] - ψp [source]) would have been about -1.6 to -3.5 bars, which compares favorably with the -1.07 to -2.41 bars average gradient that would have been required to drive translocation at the observed velocities.
Full text
PDF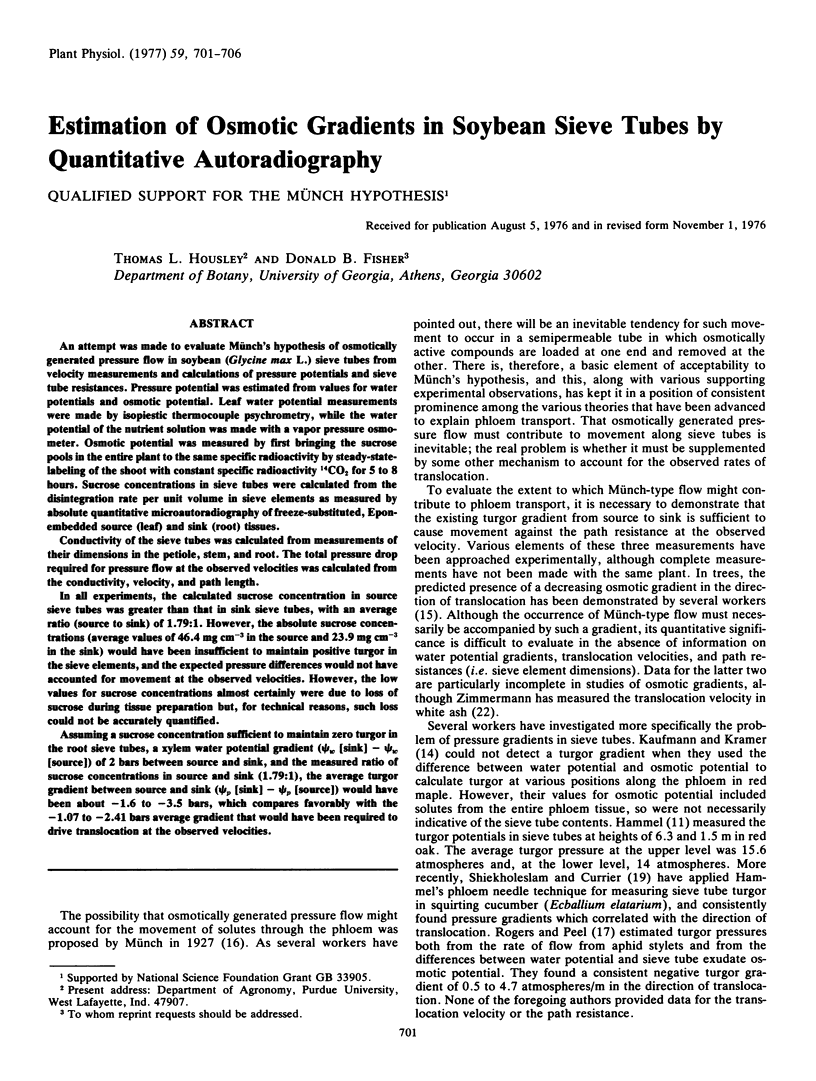
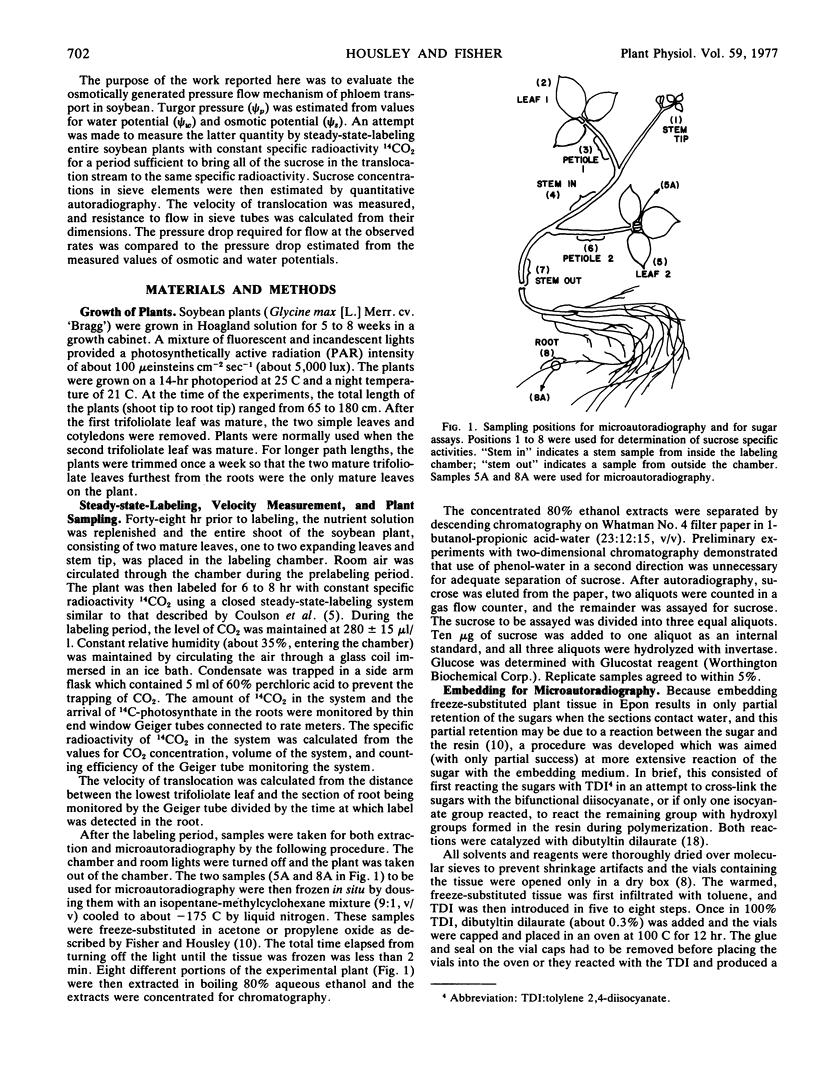
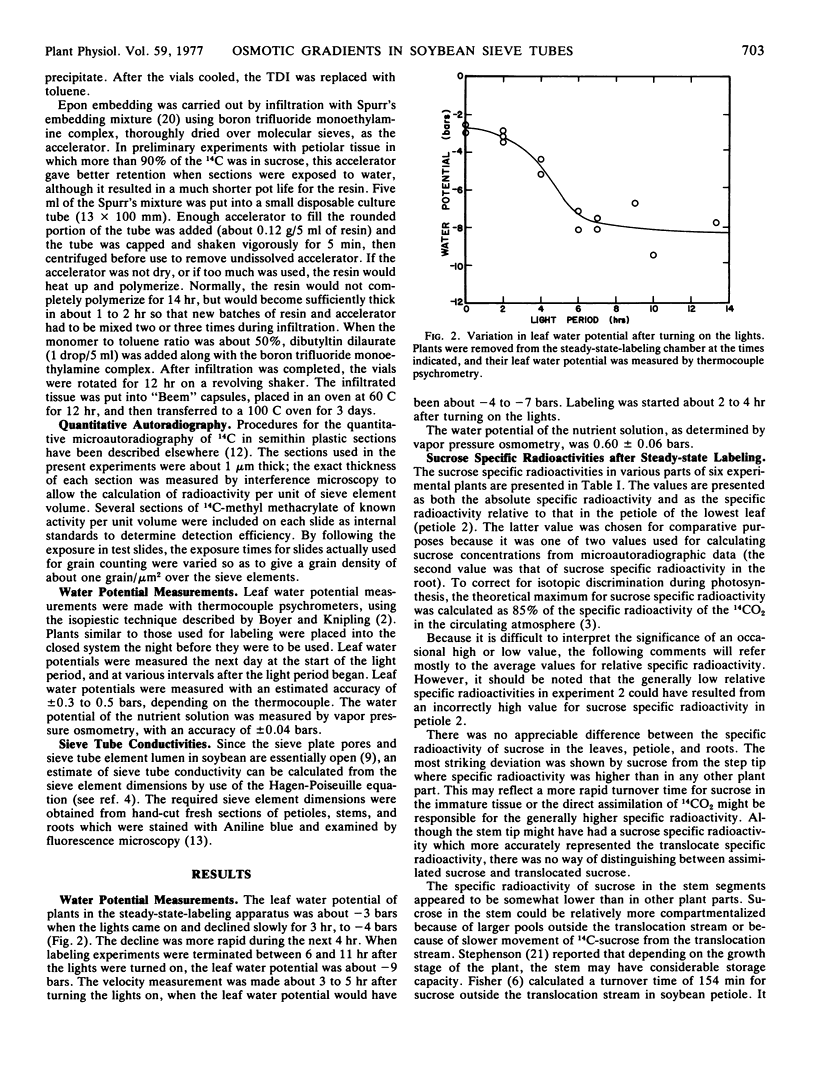
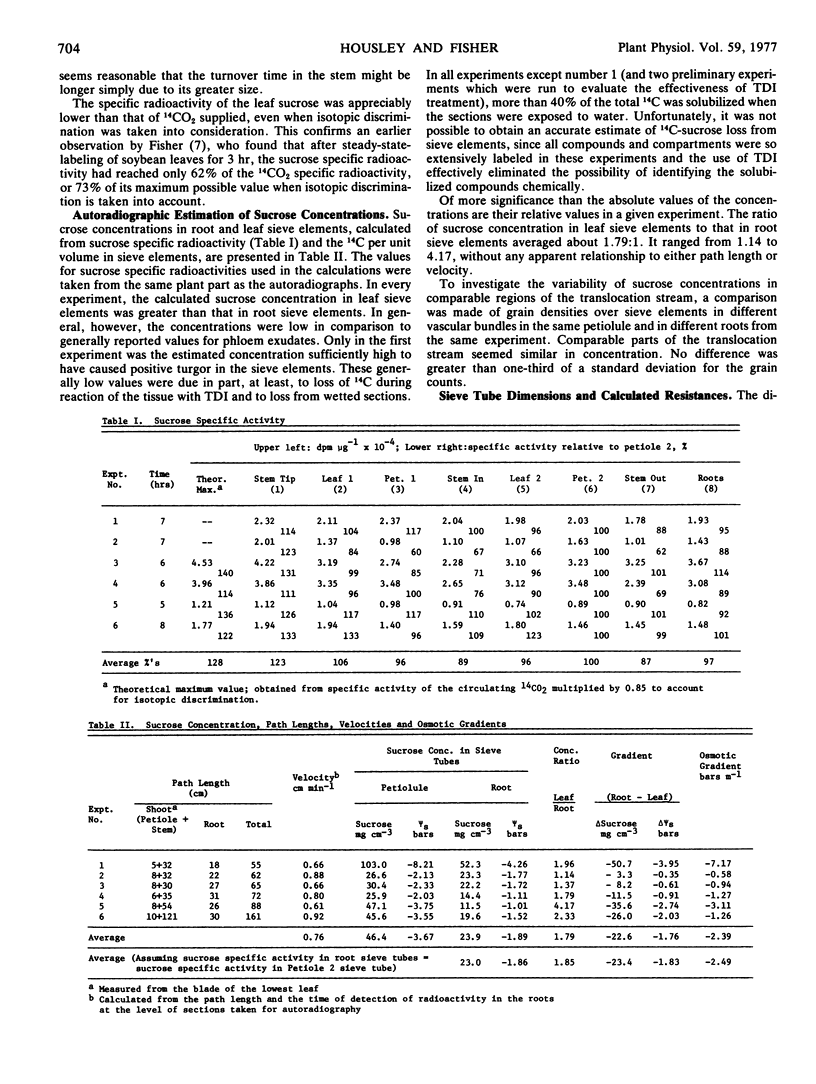
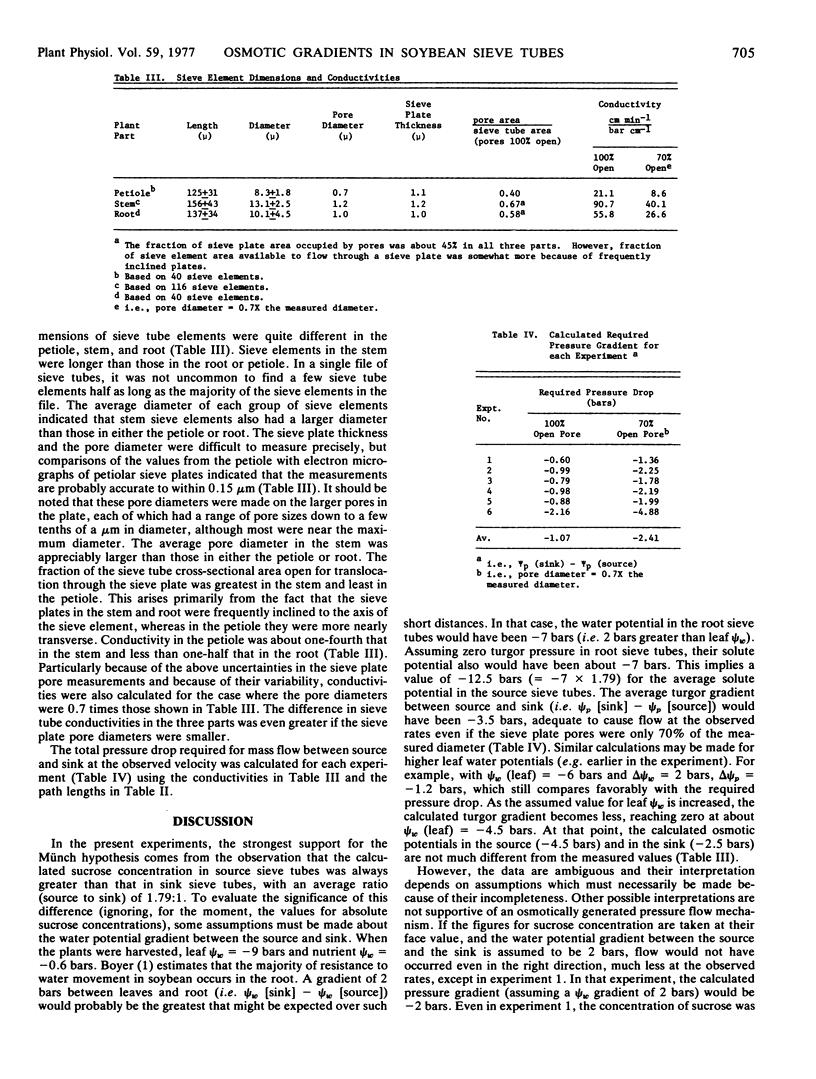
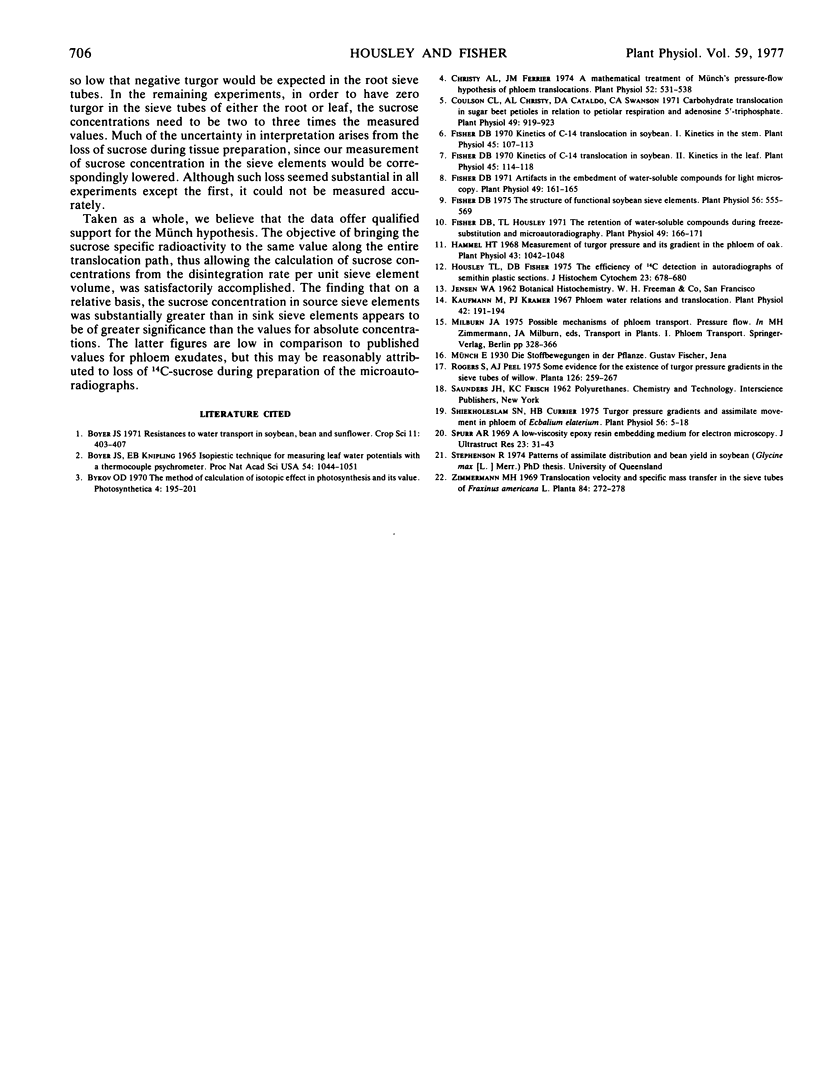
Selected References
These references are in PubMed. This may not be the complete list of references from this article.
- Christy A. L., Ferrier J. M. A Mathematical Treatment of Munch's Pressure-Flow Hypothesis of Phloem Translocation. Plant Physiol. 1973 Dec;52(6):531–538. doi: 10.1104/pp.52.6.531. [DOI] [PMC free article] [PubMed] [Google Scholar]
- Coulson C. L., Christy A. L., Cataldo D. A., Swanson C. A. Carbohydrate translocation in sugar beet petioles in relation to petiolar respiration and adenosine 5'-triphosphate. Plant Physiol. 1972 Jun;49(6):919–923. doi: 10.1104/pp.49.6.919. [DOI] [PMC free article] [PubMed] [Google Scholar]
- Fisher D. B. Artifacts in the Embedment of Water-soluble Compounds for Light Microscopy. Plant Physiol. 1972 Feb;49(2):161–165. doi: 10.1104/pp.49.2.161. [DOI] [PMC free article] [PubMed] [Google Scholar]
- Fisher D. B., Housley T. L. The Retention of Water-soluble Compounds during Freeze-Substitution and Microautoradiography. Plant Physiol. 1972 Feb;49(2):166–171. doi: 10.1104/pp.49.2.166. [DOI] [PMC free article] [PubMed] [Google Scholar]
- Fisher D. B. Kinetics of C-14 Translocation in Soybean: II. Kinetics in the Leaf. Plant Physiol. 1970 Feb;45(2):114–118. doi: 10.1104/pp.45.2.114. [DOI] [PMC free article] [PubMed] [Google Scholar]
- Fisher D. B. Kinetics of C-14 translocation in soybean: I. Kinetics in the stem. Plant Physiol. 1970 Feb;45(2):107–113. doi: 10.1104/pp.45.2.107. [DOI] [PMC free article] [PubMed] [Google Scholar]
- Fisher D. B. Structure of functional soybean sieve elements. Plant Physiol. 1975 Nov;56(5):555–569. doi: 10.1104/pp.56.5.555. [DOI] [PMC free article] [PubMed] [Google Scholar]
- Hammel H. T. Measurement of turgor pressure and its gradient in the Phloem of oak. Plant Physiol. 1968 Jul;43(7):1042–1048. doi: 10.1104/pp.43.7.1042. [DOI] [PMC free article] [PubMed] [Google Scholar]
- Housley T. L., Fisher D. B. The efficiency of 14C detection in autoradiographs of semithin plastic sections. J Histochem Cytochem. 1975 Sep;23(9):678–680. doi: 10.1177/23.9.1176761. [DOI] [PubMed] [Google Scholar]
- Kaufmann M. R., Kramer P. J. Phloem water relations and translocation. Plant Physiol. 1967 Feb;42(2):191–194. doi: 10.1104/pp.42.2.191. [DOI] [PMC free article] [PubMed] [Google Scholar]
- Spurr A. R. A low-viscosity epoxy resin embedding medium for electron microscopy. J Ultrastruct Res. 1969 Jan;26(1):31–43. doi: 10.1016/s0022-5320(69)90033-1. [DOI] [PubMed] [Google Scholar]
- Twente J. W., Twente J. A. Regulation of hibernating periods by temperature. Proc Natl Acad Sci U S A. 1965 Oct;54(4):1044–1051. [PMC free article] [PubMed] [Google Scholar]