Abstract
Phospho-beta-galactosidase (P-beta-gal), the enzyme which catalyzes the first step in the metabolism of intracellular lactose phosphate, occurred at high specific activity in the cytoplasm in 12 of 13 strains of streptococcus mutans grown on lactose but not other carbon sources. The P-beta-gal from S. mutans SL1 was purified 13-fold using diethylaminoethyl-cellulose ion exchange and agarose A--0.5 M molecular exclusion column chromatography. The molecualr weight of the enzyme was estimated to be 40,000, and its pH optimum was 6.5 in three different buffer systems. P-beta-gal activity was inhibited by Co2+, Zn2+, and Cu2+, but other cations, ethylenediaminetetraacetic acid, orthophosphate, and fluoride had no effect upon enzyme activity. The kinetic response of P-beta-gal to a model substrate, o-nitrophenyl-beta-D-galactopyranoside-6-phosphate, obeyed Michaelis-Menten kinetics, and the Km for this substrate was 0.19 mM. In addition to being under genetic control, P-beta-gal activity was regulated by a number of biologically active metabolites. Enzyme activity was inhibited in a sigmoidal fashion by phosphoenolpyruvate. The M 0.5 V value for phosphoenolpyruvate was 2.8 mM, and the Hill coefficient (n) was 3. In addition, P-beta-gal exhibited strong inhibition by ATP, galactose-6-phosphate, and glucose-6-phosphate. In contrast to inhibition of P-beta-gal activity by phosphoenolpyruvate, the inhibition exerted by ATP, galactose-6-phosphate, and glucose-6-phosphate obeyed classical Michaelis-Menten kinetics; the Ki values for these inhibitors were 0.55, 1.6, and 4.0 mM, respectively.
Full text
PDF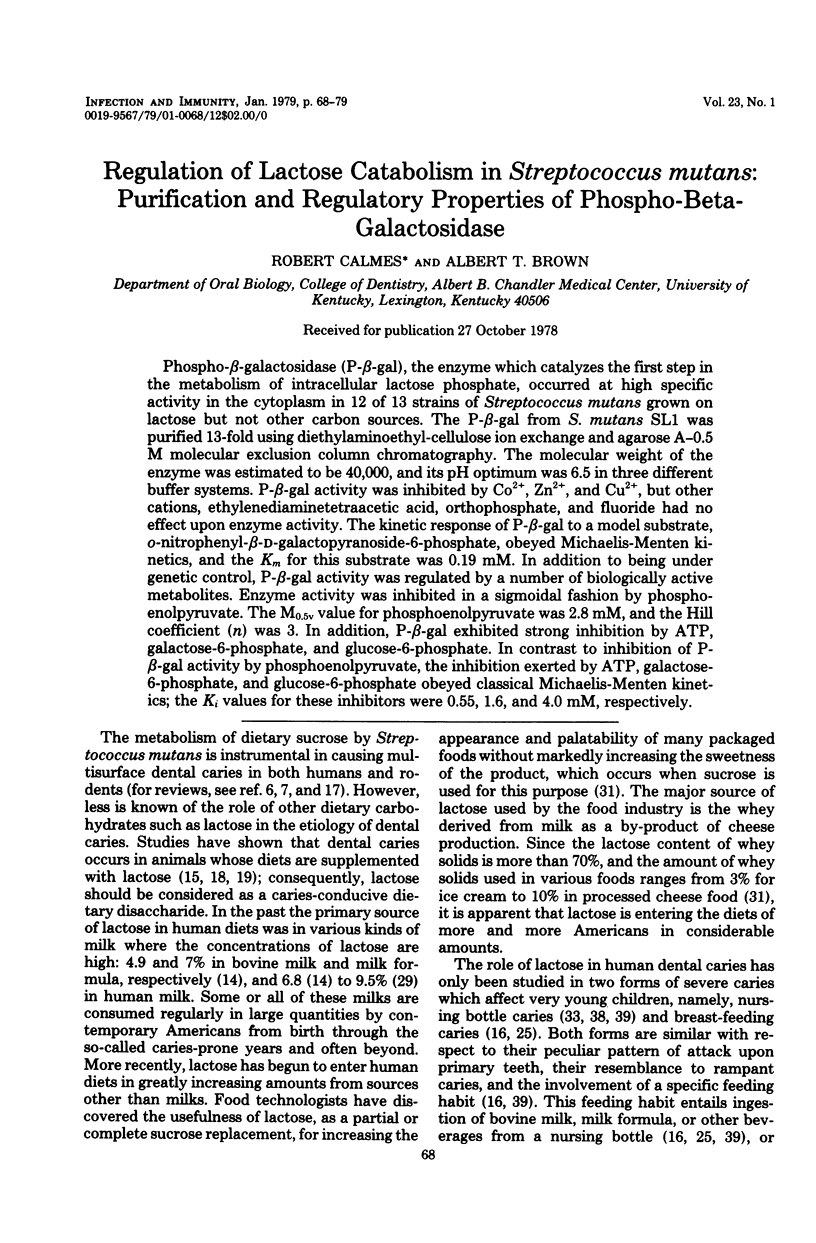
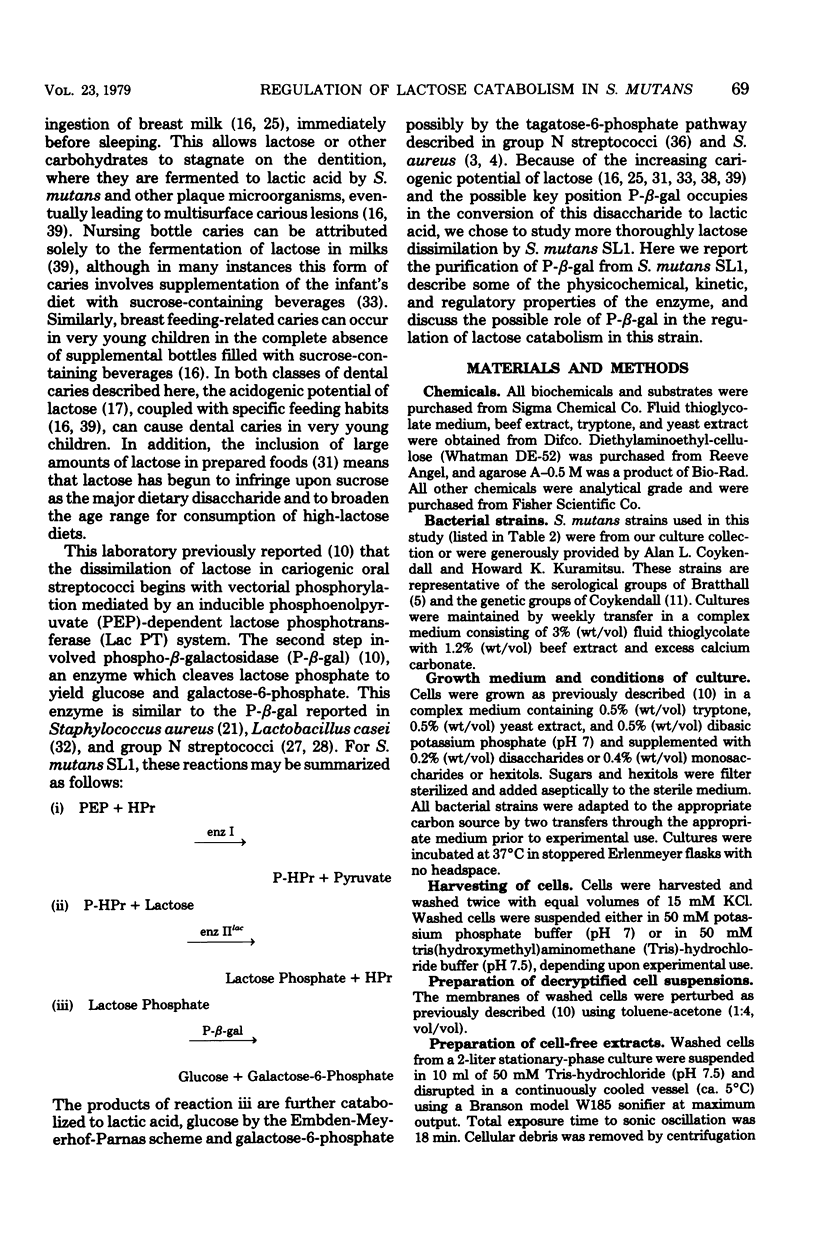
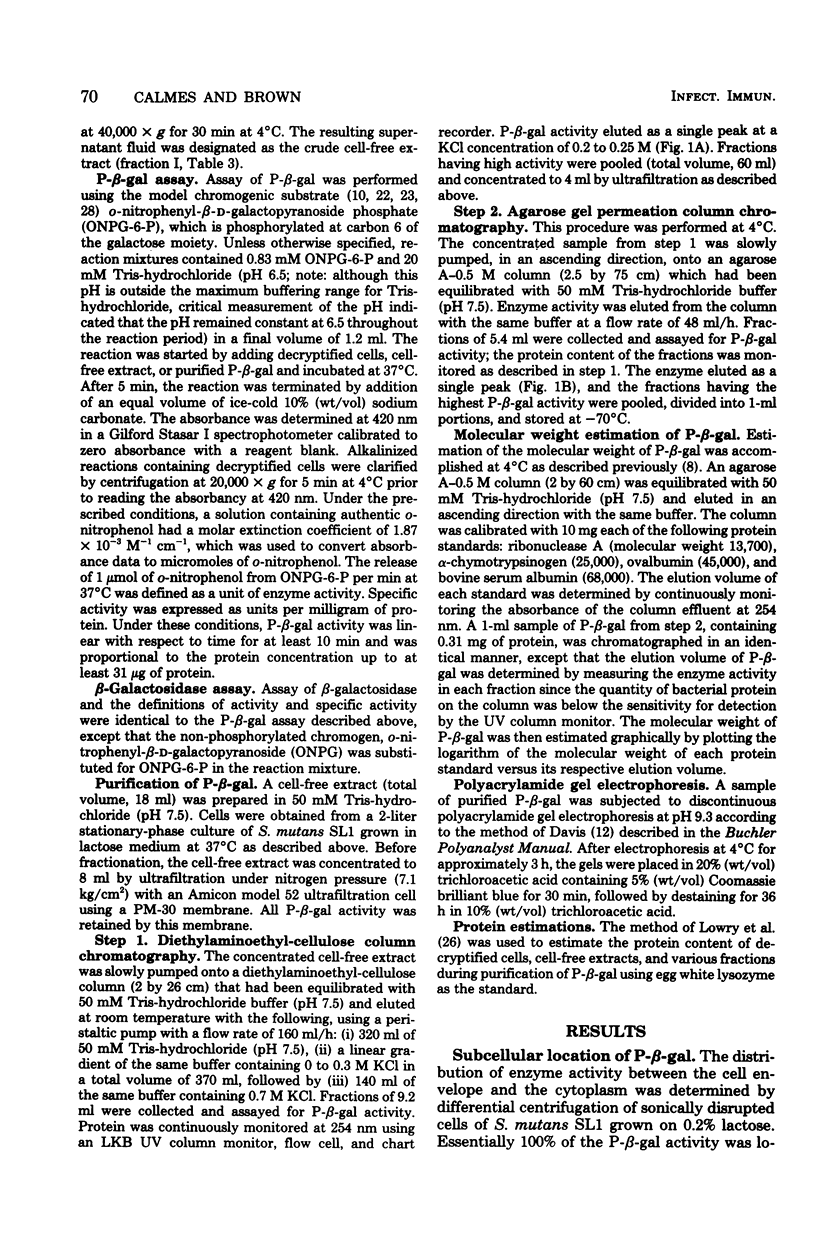
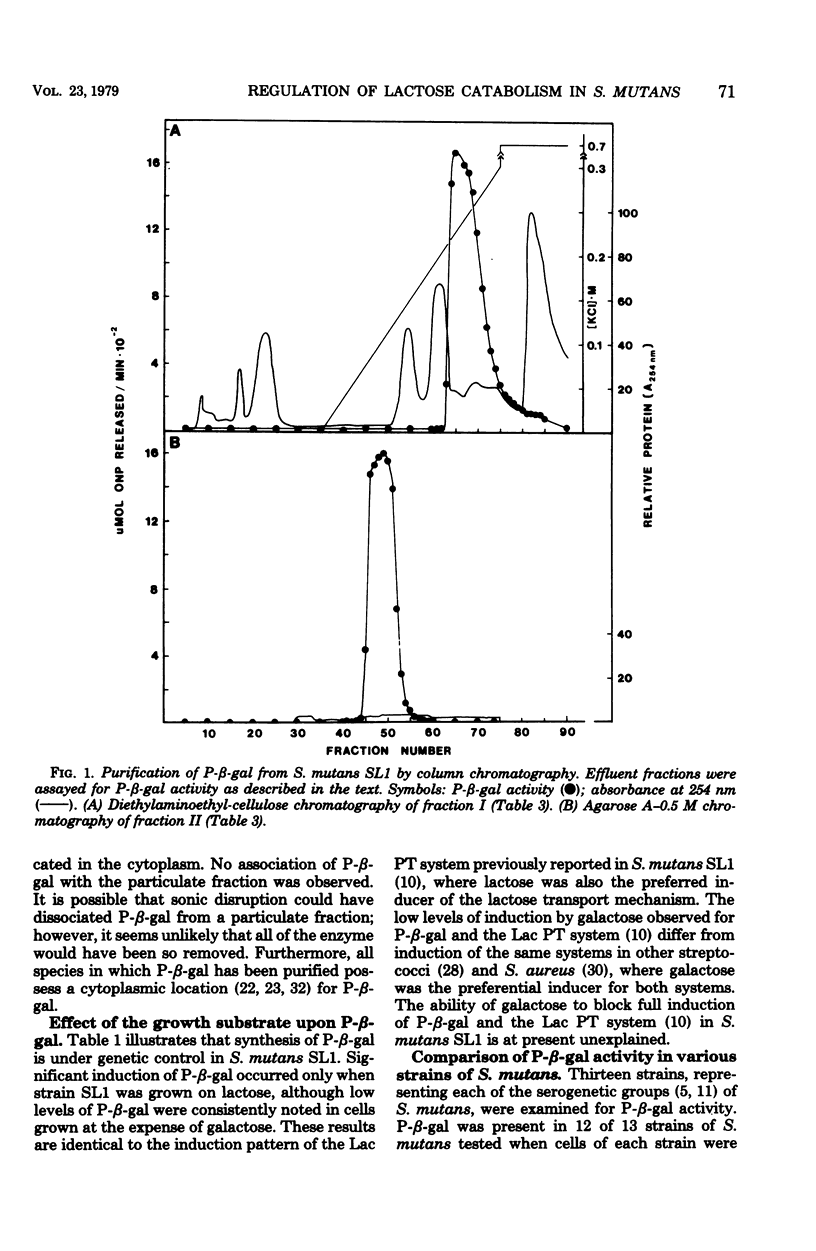
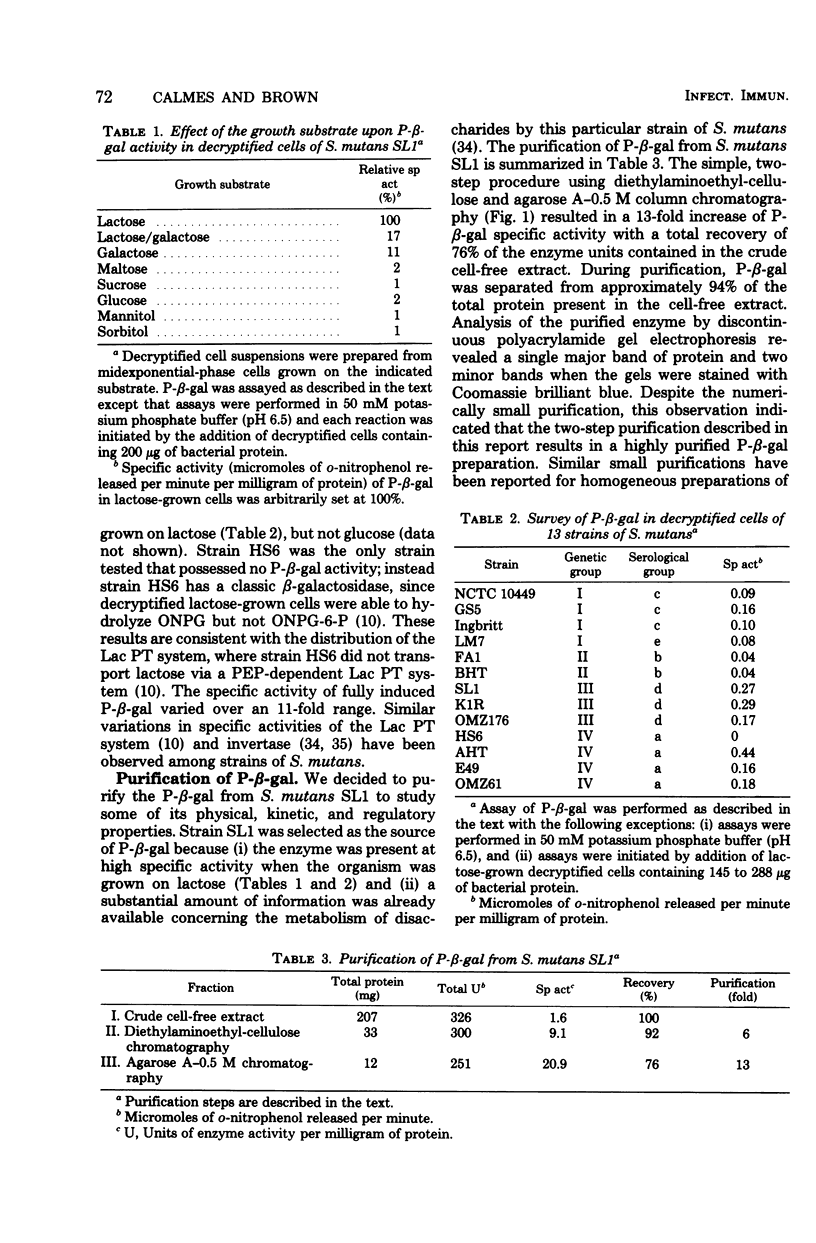
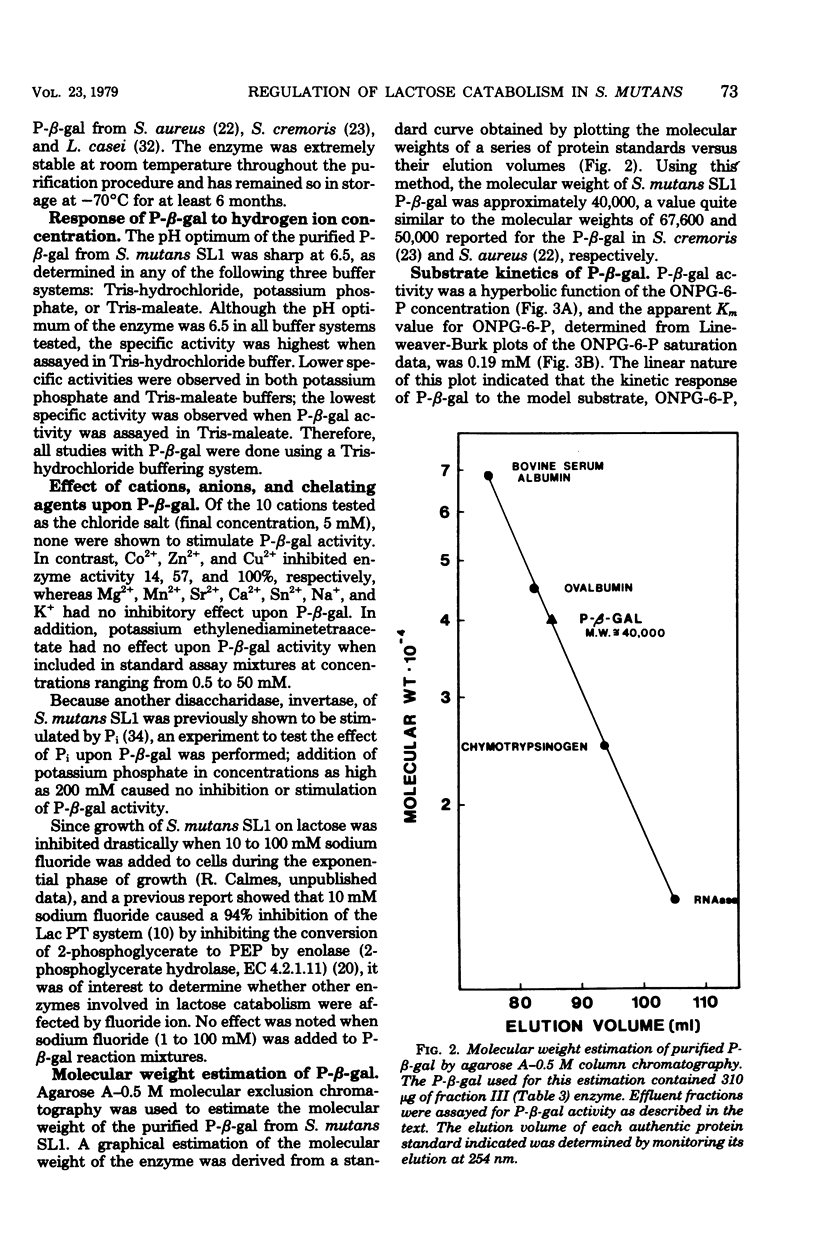
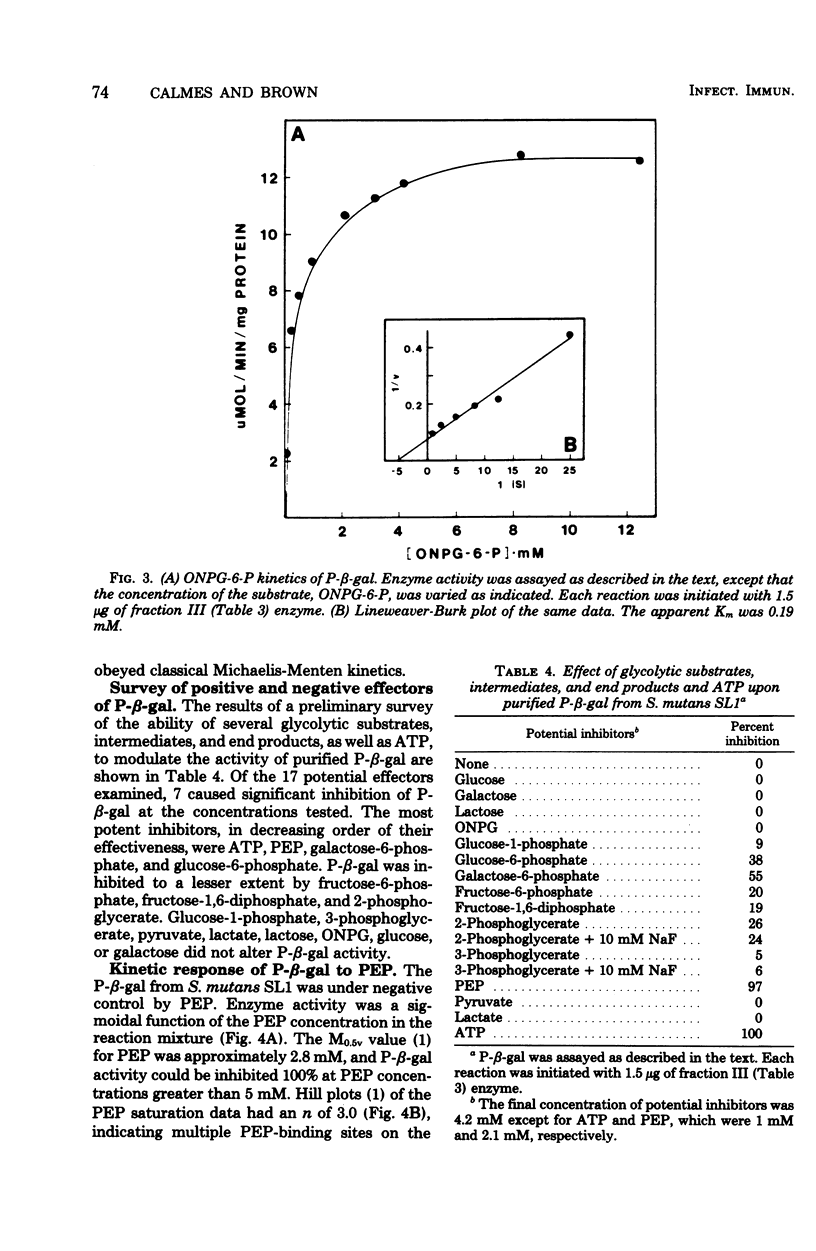
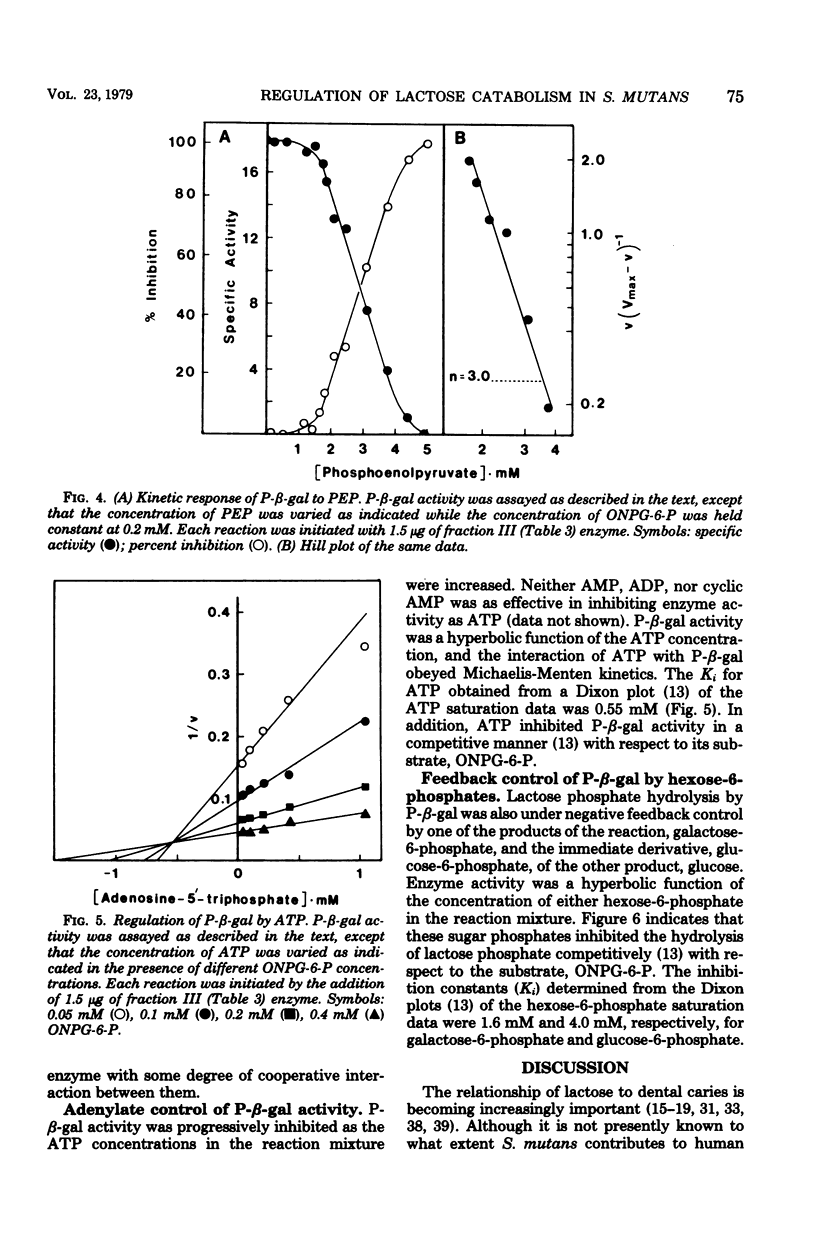
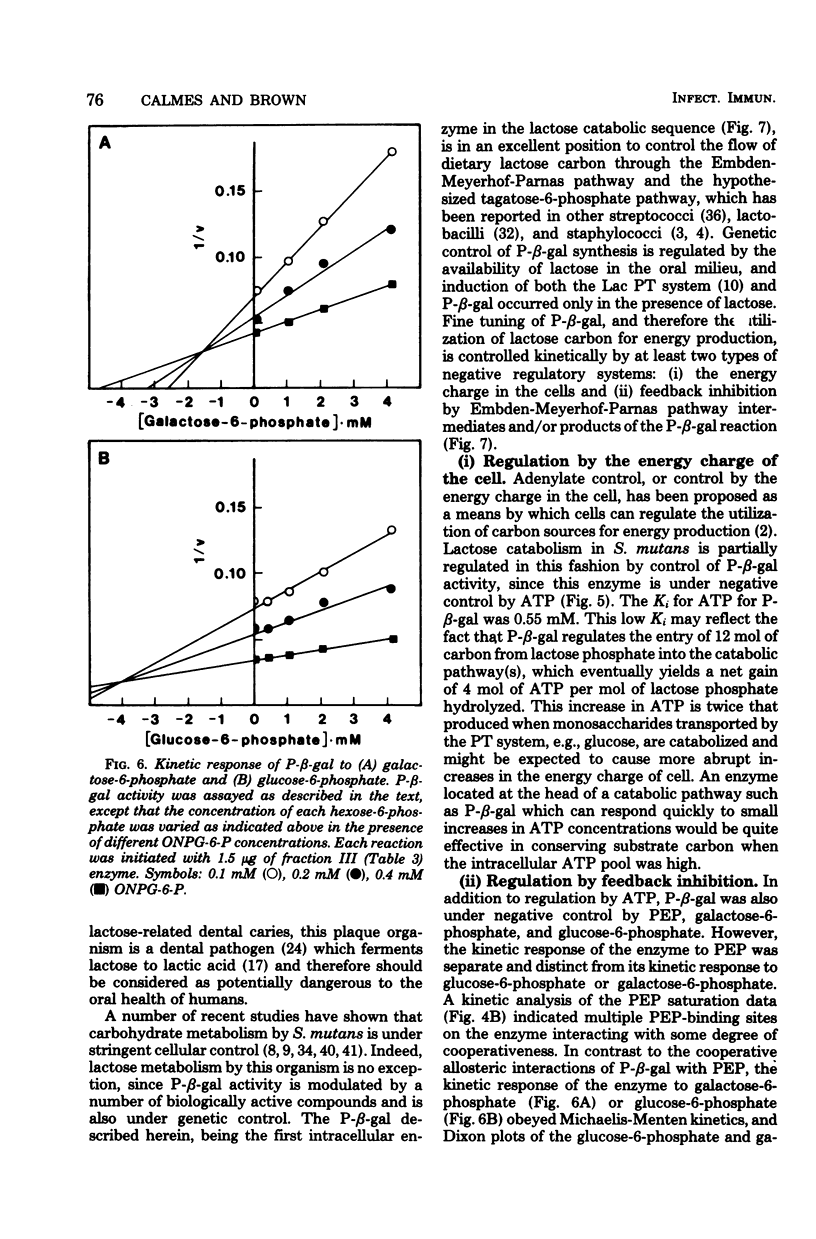
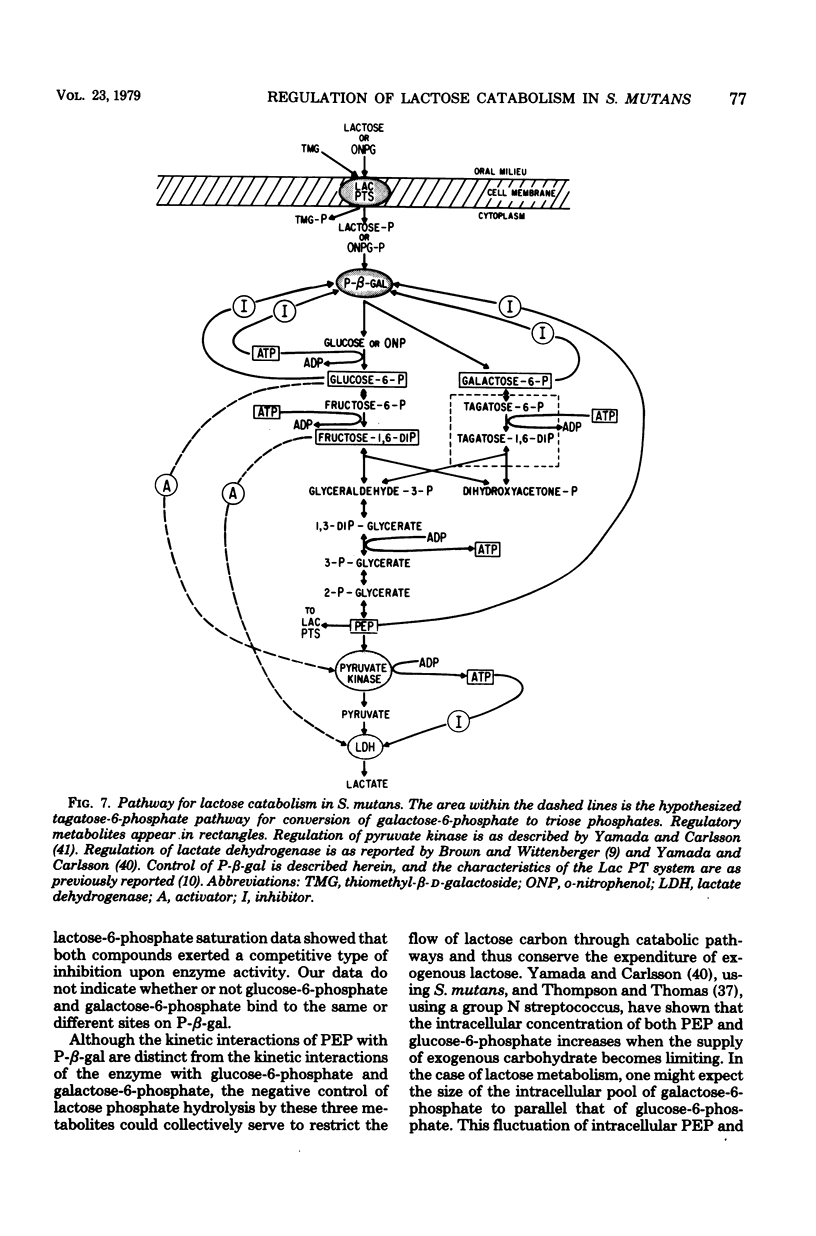
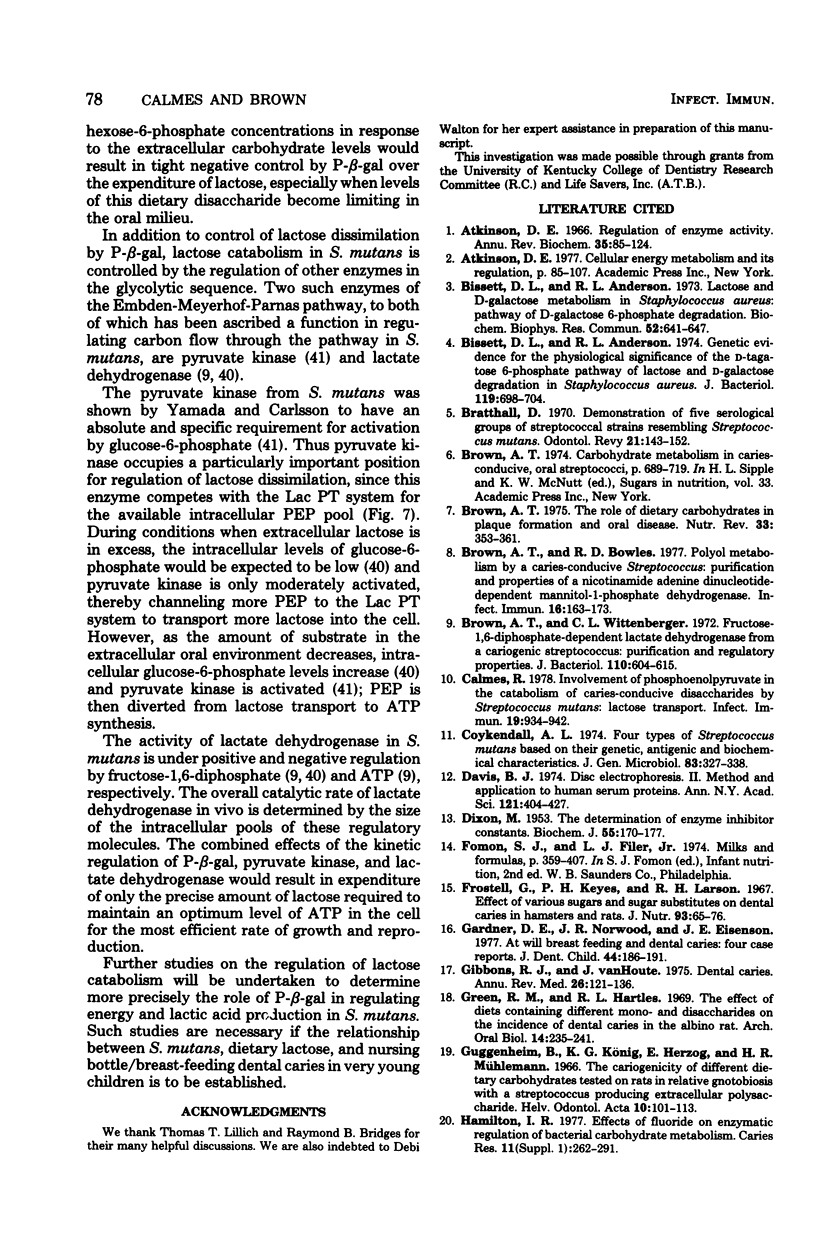
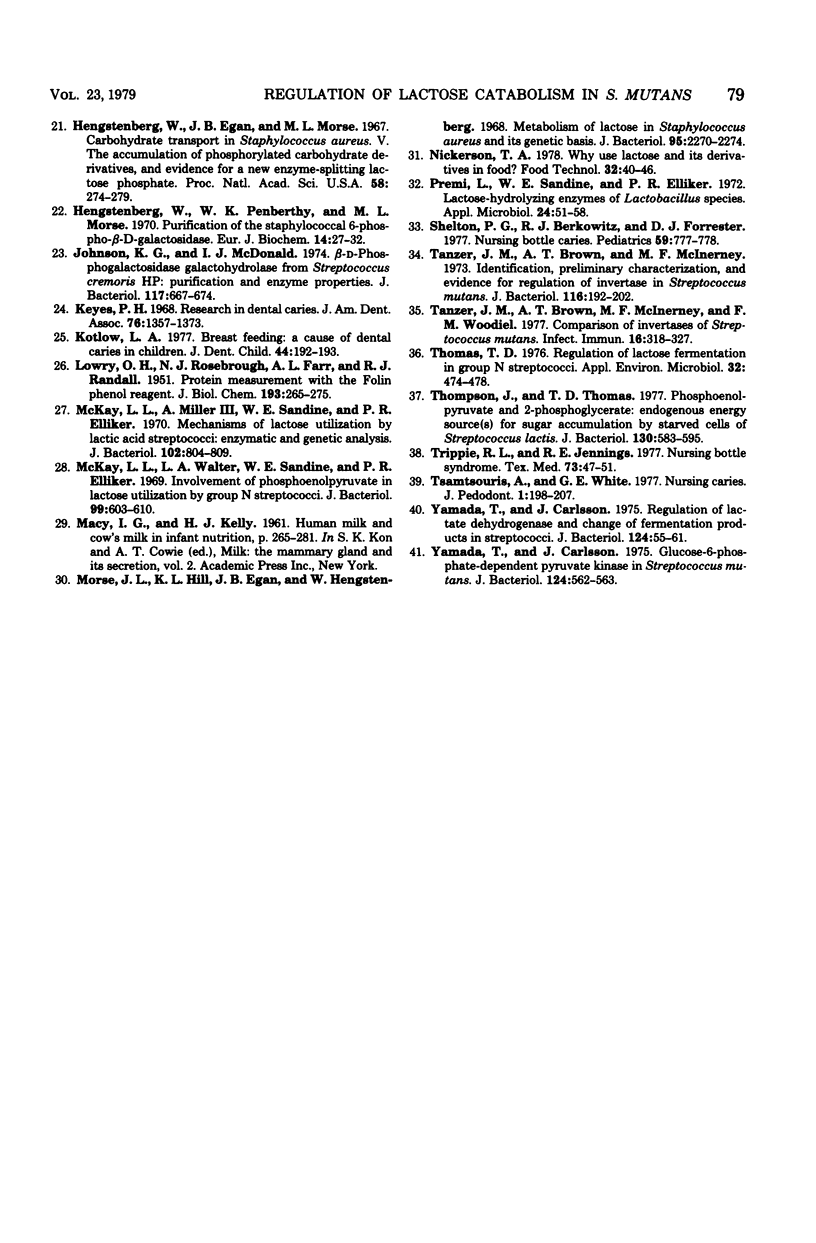
Selected References
These references are in PubMed. This may not be the complete list of references from this article.
- Bissett D. L., Anderson R. L. Genetic evidence for the physiological significance of the D-tagatose 6-phosphate pathway of lactose and D-galactose degradation in staphylococcus aureus. J Bacteriol. 1974 Sep;119(3):698–704. doi: 10.1128/jb.119.3.698-704.1974. [DOI] [PMC free article] [PubMed] [Google Scholar]
- Bissett D. L., Anderson R. L. Lactose and D0galactose metabolism in Staphylococcus aureus: pathway of D-galactose 6-phosphate degradation. Biochem Biophys Res Commun. 1973 May 15;52(2):641–647. doi: 10.1016/0006-291x(73)90761-4. [DOI] [PubMed] [Google Scholar]
- Bratthall D. Demonstration of five serological groups of streptococcal strains resembling Streptococcus mutans. Odontol Revy. 1970;21(2):143–152. [PubMed] [Google Scholar]
- Brown A. T., Bowles R. D. Polyol metabolism by a caries-conducive Streptococcus: purification and properties of a nicotinamide adenine dinucleotide-dependent mannitol-1-phosphate dehydrogenase. Infect Immun. 1977 Apr;16(1):163–173. doi: 10.1128/iai.16.1.163-173.1977. [DOI] [PMC free article] [PubMed] [Google Scholar]
- Brown A. T. The role of dietary carbohydrates in plaque formation and oral disease. Nutr Rev. 1975 Dec;33(12):353–361. doi: 10.1111/j.1753-4887.1975.tb05089.x. [DOI] [PubMed] [Google Scholar]
- Brown A. T., Wittenberger C. L. Fructose-1,6-diphosphate-dependent lactate dehydrogenase from a cariogenic streptococcus: purification and regulatory properties. J Bacteriol. 1972 May;110(2):604–615. doi: 10.1128/jb.110.2.604-615.1972. [DOI] [PMC free article] [PubMed] [Google Scholar]
- Calmes R. Involvement of phosphoenolpyruvate in the catabolism of caries-conducive disaccharides by Streptococcus mutans: lactose transport. Infect Immun. 1978 Mar;19(3):934–942. doi: 10.1128/iai.19.3.934-942.1978. [DOI] [PMC free article] [PubMed] [Google Scholar]
- Coykendall A. L. Four types of Streptococcus mutans based on their genetic, antigenic and biochemical characteristics. J Gen Microbiol. 1974 Aug;83(2):327–338. doi: 10.1099/00221287-83-2-327. [DOI] [PubMed] [Google Scholar]
- DAVIS B. J. DISC ELECTROPHORESIS. II. METHOD AND APPLICATION TO HUMAN SERUM PROTEINS. Ann N Y Acad Sci. 1964 Dec 28;121:404–427. doi: 10.1111/j.1749-6632.1964.tb14213.x. [DOI] [PubMed] [Google Scholar]
- DIXON M. The determination of enzyme inhibitor constants. Biochem J. 1953 Aug;55(1):170–171. doi: 10.1042/bj0550170. [DOI] [PMC free article] [PubMed] [Google Scholar]
- Frostell G., Keyes P. H., Larson R. H. Effect of various sugars and sugar substitutes on dental caries in hamsters and rats. J Nutr. 1967 Sep;93(1):65–76. doi: 10.1093/jn/93.1.65. [DOI] [PubMed] [Google Scholar]
- Gardner D. E., Norwood J. R., Eisenson J. E. At-will breast feeding and dental caries: four case reports. ASDC J Dent Child. 1977 May-Jun;44(3):186–191. [PubMed] [Google Scholar]
- Gibbons R. J., van Houte J. Dental caries. Annu Rev Med. 1975;26:121–136. doi: 10.1146/annurev.me.26.020175.001005. [DOI] [PubMed] [Google Scholar]
- Green R. M., Hartles R. L. The effect of diets contaning different mono- and disaccharides on the incidence of dental caries in the albino rat. Arch Oral Biol. 1969 Mar;14(3):235–241. doi: 10.1016/0003-9969(69)90225-8. [DOI] [PubMed] [Google Scholar]
- Guggenheim B., König K. G., Herzog E., Mühlemann H. R. The cariogenicity of different dietary carbohydrates tested on rats in relative gnotobiosis with a Streptococcus producing extracellular polysaccharide. Helv Odontol Acta. 1966 Oct;10(2):101–113. [PubMed] [Google Scholar]
- Hamilton I. R. Effects of fluoride on enzymatic regulation of bacterial carbohydrate metabolism. Caries Res. 1977;11 (Suppl 1):262–291. doi: 10.1159/000260304. [DOI] [PubMed] [Google Scholar]
- Hengstenberg W., Egan J. B., Morse M. L. Carbohydrate transport in Staphylococcus aureus. V. The accumulation of phosphorylated carbohydrate derivatives, and evidence for a new enzyme-splitting lactose phosphate. Proc Natl Acad Sci U S A. 1967 Jul;58(1):274–279. doi: 10.1073/pnas.58.1.274. [DOI] [PMC free article] [PubMed] [Google Scholar]
- Hengstenberg W., Penberthy W. K., Morse M. L. Purification of the staphylococcal 6-phospho-beta-D-- galactosidase. Eur J Biochem. 1970 May 1;14(1):27–32. doi: 10.1111/j.1432-1033.1970.tb00256.x. [DOI] [PubMed] [Google Scholar]
- Johnson K. G., McDonald I. J. Beta-D-phosphogalactoside galactohydrolase from Streptococcus cremoris HP: purification and enzyme properties. J Bacteriol. 1974 Feb;117(2):667–674. doi: 10.1128/jb.117.2.667-674.1974. [DOI] [PMC free article] [PubMed] [Google Scholar]
- Keyes P. H. Research in dental caries. J Am Dent Assoc. 1968 Jun;76(6):1357–1373. doi: 10.14219/jada.archive.1968.0186. [DOI] [PubMed] [Google Scholar]
- Kotlow L. A. Breast feeding: a cause of dental caries in children. ASDC J Dent Child. 1977 May-Jun;44(3):192–193. [PubMed] [Google Scholar]
- LOWRY O. H., ROSEBROUGH N. J., FARR A. L., RANDALL R. J. Protein measurement with the Folin phenol reagent. J Biol Chem. 1951 Nov;193(1):265–275. [PubMed] [Google Scholar]
- McKay L. L., Walter L. A., Sandine W. E., Elliker P. R. Involvement of phosphoenolpyruvate in lactose utilization by group N streptococci. J Bacteriol. 1969 Aug;99(2):603–610. doi: 10.1128/jb.99.2.603-610.1969. [DOI] [PMC free article] [PubMed] [Google Scholar]
- McKay L., Miller A., 3rd, Sandine W. E., Elliker P. R. Mechanisms of lactose utilization by lactic acid streptococci: enzymatic and genetic analyses. J Bacteriol. 1970 Jun;102(3):804–809. doi: 10.1128/jb.102.3.804-809.1970. [DOI] [PMC free article] [PubMed] [Google Scholar]
- Morse M. L., Hill K. L., Egan J. B., Hengstenberg W. Metabolism of lactose by Staphylococcus aureus and its genetic basis. J Bacteriol. 1968 Jun;95(6):2270–2274. doi: 10.1128/jb.95.6.2270-2274.1968. [DOI] [PMC free article] [PubMed] [Google Scholar]
- Premi L., Sandine W. E., Elliker P. R. Lactose-hydrolyzing enzymes of Lactobacillus species. Appl Microbiol. 1972 Jul;24(1):51–57. doi: 10.1128/am.24.1.51-57.1972. [DOI] [PMC free article] [PubMed] [Google Scholar]
- Shelton P. G., Berkowitz R. J., Forrester D. J. Nursing bottle caries. Pediatrics. 1977 May;59(5):777–778. [PubMed] [Google Scholar]
- Tanzer J. M., Brown A. T., McInerney M. F. Identification, preliminary characterization, and evidence for regulation of invertase in Streptococcus mutans. J Bacteriol. 1973 Oct;116(1):192–202. doi: 10.1128/jb.116.1.192-202.1973. [DOI] [PMC free article] [PubMed] [Google Scholar]
- Tanzer J. M., Brown A. T., McInerney M. F., Woodiel F. N. Comparative study of invertases of Streptococcus mutans. Infect Immun. 1977 Apr;16(1):318–327. doi: 10.1128/iai.16.1.318-327.1977. [DOI] [PMC free article] [PubMed] [Google Scholar]
- Thomas T. D. Regulation of lactose fermentation in group N streptococci. Appl Environ Microbiol. 1976 Oct;32(4):474–478. doi: 10.1128/aem.32.4.474-478.1976. [DOI] [PMC free article] [PubMed] [Google Scholar]
- Thompson J., Thomas T. D. Phosphoenolpyruvate and 2-phosphoglycerate: endogenous energy source(s) for sugar accumulation by starved cells of Streptococcus lactis. J Bacteriol. 1977 May;130(2):583–595. doi: 10.1128/jb.130.2.583-595.1977. [DOI] [PMC free article] [PubMed] [Google Scholar]
- Trippie R. L., Jennings R. E. Nursing bottle syndrome. Tex Med. 1977 Mar;73(3):47–51. [PubMed] [Google Scholar]
- Tsamtsouris A., White G. E. Nursing caries. J Pedod. 1977 Spring;1(3):198–207. [PubMed] [Google Scholar]
- Yamada T., Carlsson J. Glucose-6-phosphate-dependent pyruvate kinase in Streptococcus mutans. J Bacteriol. 1975 Oct;124(1):562–563. doi: 10.1128/jb.124.1.562-563.1975. [DOI] [PMC free article] [PubMed] [Google Scholar]
- Yamada T., Carlsson J. Regulation of lactate dehydrogenase and change of fermentation products in streptococci. J Bacteriol. 1975 Oct;124(1):55–61. doi: 10.1128/jb.124.1.55-61.1975. [DOI] [PMC free article] [PubMed] [Google Scholar]