Abstract
The three populations of synaptic vesicles in electromotor nerve terminals were analysed quantitatively. Empty vesicles (VP0), fully charged vesicles (VP1) and charged but smaller VP2-type vesicles are present in approximately equal amounts in the nerve terminal. The populations show differences in the kinetics of in vitro uptake of acetylcholine, ATP and Ca2+. VP0 and VP2 accumulate acetylcholine and ATP but no Ca2+, whereas VP1 shows negligible acetylcholine and ATP but high Ca2+ uptake. Thus the expression of uptake properties of this secretory organelle depend on the stage it has reached in its life cycle and might constitute a signal for processing. VP2 was found to contain much less core proteoglycan than VP0 and VP1 indicating that part of it has been lost by exocytosis. In synaptic extracellular matrix containing fractions an antigen is detectable that cross-reacts with an antiserum against the vesicle proteoglycan. This material elutes upon gel filtration in a position similar to a smaller form of proteoglycan found in vesicles. We conclude that the electromotor nerve terminal releases a proteoglycan by the regulated secretory pathway that is deposited in the extracellular matrix. It might have a function in keeping pre- and postsynaptic structures in alignment constituting a transsynaptic signal. Based on the findings described, a model of the vesicles' life-cycle is discussed, whereby the VP2 population is the major source of quantal release of acetylcholine.
Full text
PDF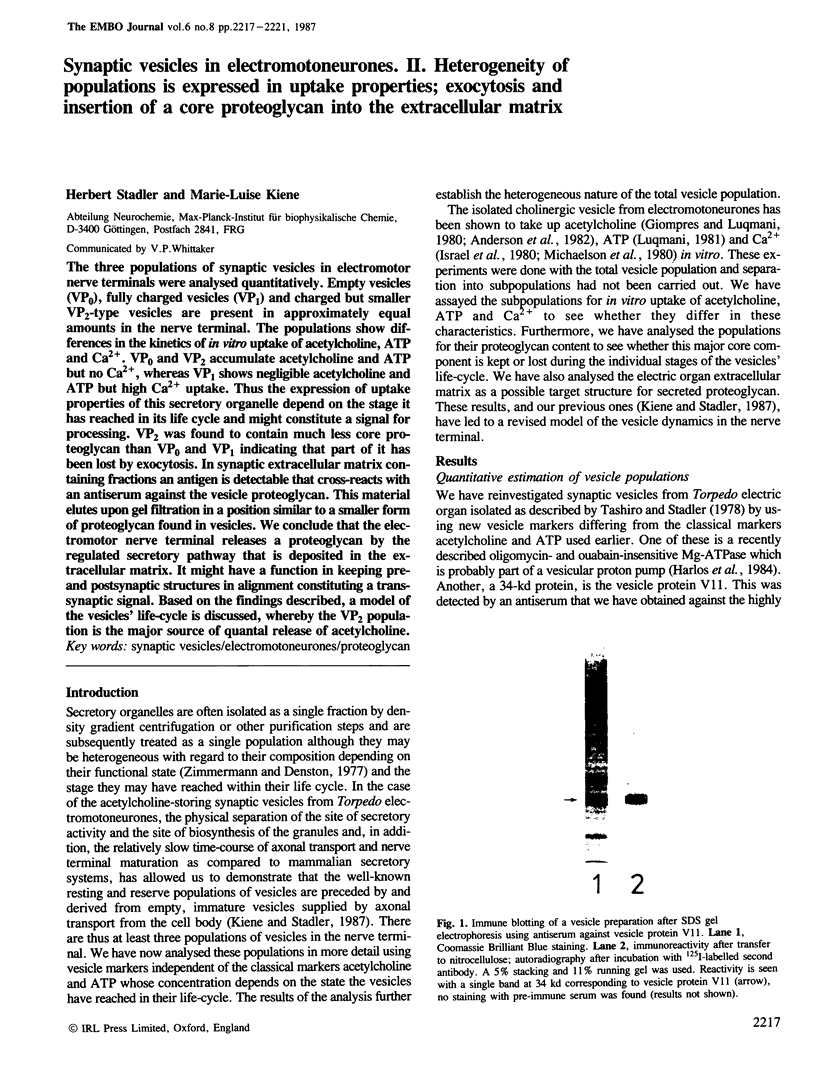
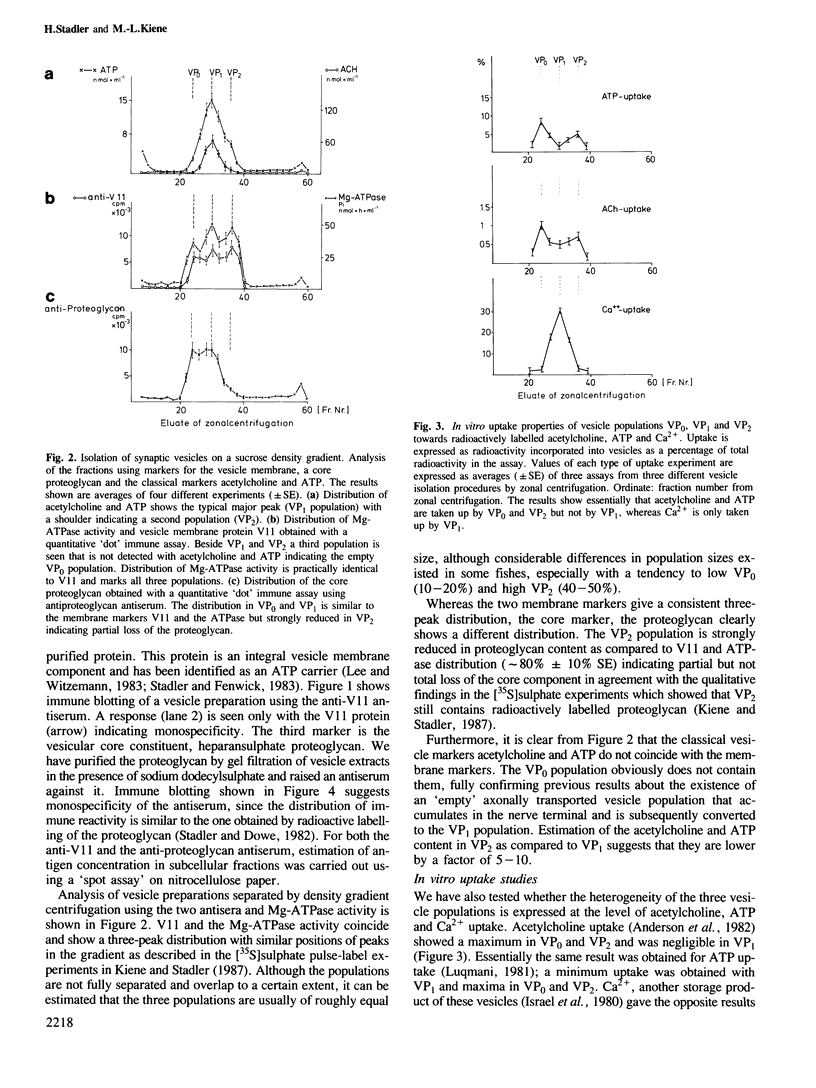
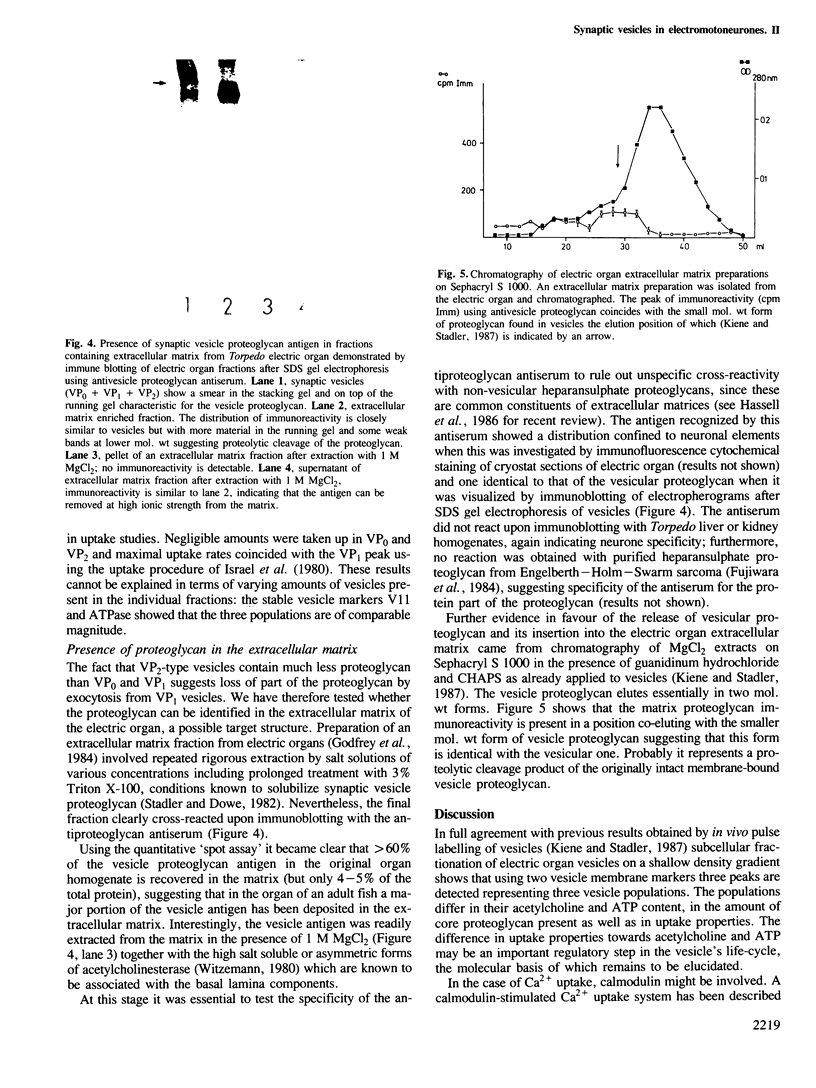
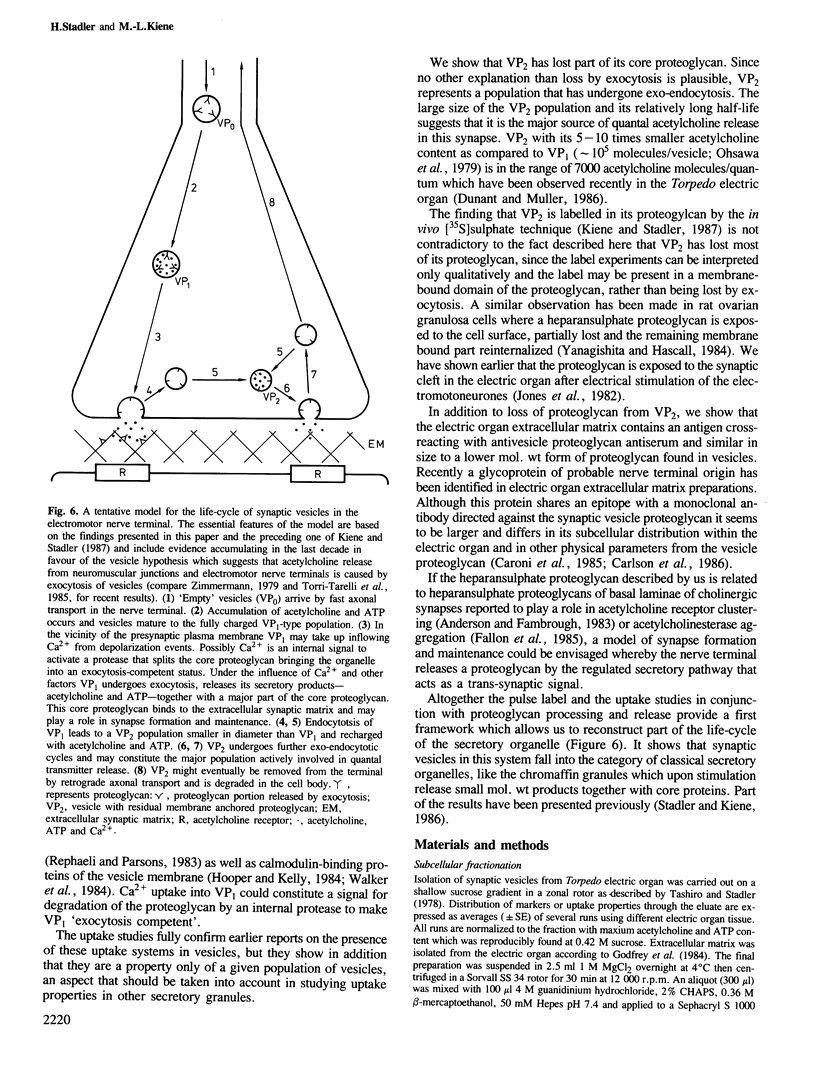
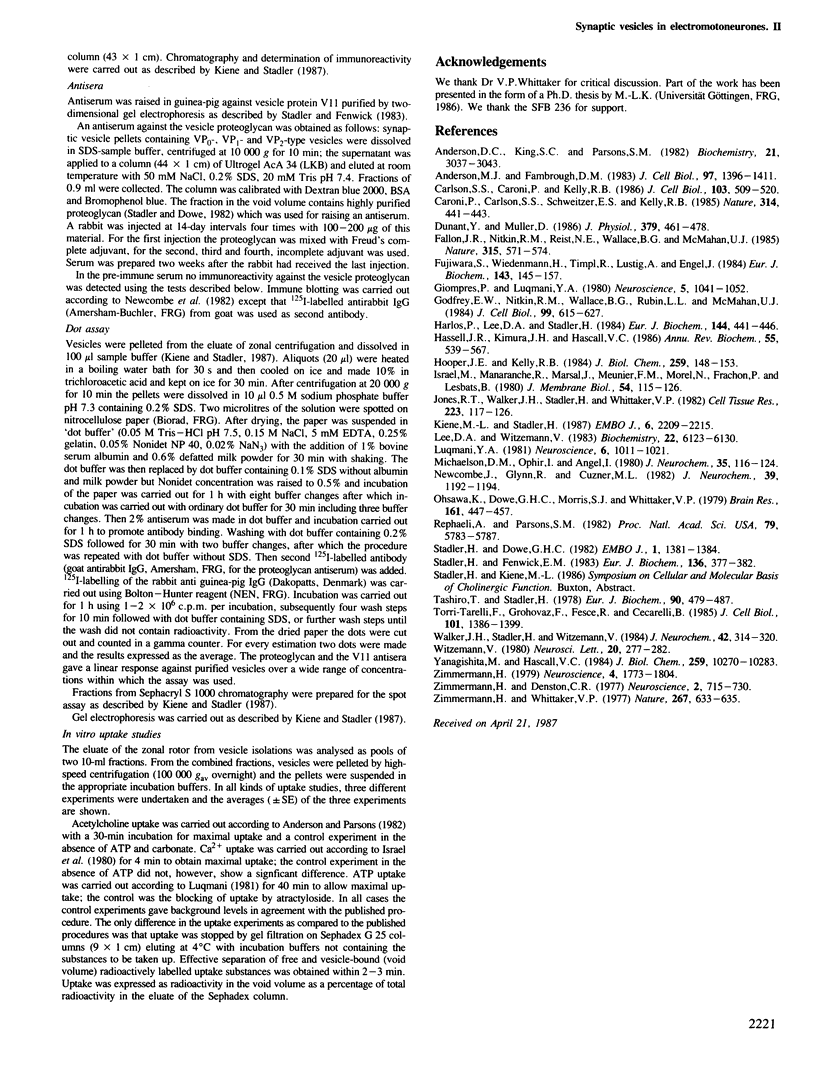
Images in this article
Selected References
These references are in PubMed. This may not be the complete list of references from this article.
- Anderson D. C., King S. C., Parsons S. M. Proton gradient linkage to active uptake of [3H]acetylcholine by Torpedo electric organ synaptic vesicles. Biochemistry. 1982 Jun 22;21(13):3037–3043. doi: 10.1021/bi00256a001. [DOI] [PubMed] [Google Scholar]
- Anderson M. J., Fambrough D. M. Aggregates of acetylcholine receptors are associated with plaques of a basal lamina heparan sulfate proteoglycan on the surface of skeletal muscle fibers. J Cell Biol. 1983 Nov;97(5 Pt 1):1396–1411. doi: 10.1083/jcb.97.5.1396. [DOI] [PMC free article] [PubMed] [Google Scholar]
- Carlson S. S., Caroni P., Kelly R. B. A nerve terminal anchorage protein from electric organ. J Cell Biol. 1986 Aug;103(2):509–520. doi: 10.1083/jcb.103.2.509. [DOI] [PMC free article] [PubMed] [Google Scholar]
- Caroni P., Carlson S. S., Schweitzer E., Kelly R. B. Presynaptic neurones may contribute a unique glycoprotein to the extracellular matrix at the synapse. Nature. 1985 Apr 4;314(6010):441–443. doi: 10.1038/314441a0. [DOI] [PubMed] [Google Scholar]
- Dunant Y., Muller D. Quantal release of acetylcholine evoked by focal depolarization at the Torpedo nerve-electroplaque junction. J Physiol. 1986 Oct;379:461–478. doi: 10.1113/jphysiol.1986.sp016264. [DOI] [PMC free article] [PubMed] [Google Scholar]
- Fallon J. R., Nitkin R. M., Reist N. E., Wallace B. G., McMahan U. J. Acetylcholine receptor-aggregating factor is similar to molecules concentrated at neuromuscular junctions. Nature. 1985 Jun 13;315(6020):571–574. doi: 10.1038/315571a0. [DOI] [PubMed] [Google Scholar]
- Fujiwara S., Wiedemann H., Timpl R., Lustig A., Engel J. Structure and interactions of heparan sulfate proteoglycans from a mouse tumor basement membrane. Eur J Biochem. 1984 Aug 15;143(1):145–157. doi: 10.1111/j.1432-1033.1984.tb08353.x. [DOI] [PubMed] [Google Scholar]
- Giompres P., Luqmani Y. A. Cholinergic synaptic vesicles isolated from Torpedo marmorata: demonstration of acetylcholine and choline uptake in an in vitro system. Neuroscience. 1980;5(6):1041–1052. doi: 10.1016/0306-4522(80)90185-2. [DOI] [PubMed] [Google Scholar]
- Godfrey E. W., Nitkin R. M., Wallace B. G., Rubin L. L., McMahan U. J. Components of Torpedo electric organ and muscle that cause aggregation of acetylcholine receptors on cultured muscle cells. J Cell Biol. 1984 Aug;99(2):615–627. doi: 10.1083/jcb.99.2.615. [DOI] [PMC free article] [PubMed] [Google Scholar]
- Harlos P., Lee D. A., Stadler H. Characterization of a Mg2+-ATPase and a proton pump in cholinergic synaptic vesicles from the electric organ of Torpedo marmorata. Eur J Biochem. 1984 Nov 2;144(3):441–446. doi: 10.1111/j.1432-1033.1984.tb08485.x. [DOI] [PubMed] [Google Scholar]
- Hassell J. R., Kimura J. H., Hascall V. C. Proteoglycan core protein families. Annu Rev Biochem. 1986;55:539–567. doi: 10.1146/annurev.bi.55.070186.002543. [DOI] [PubMed] [Google Scholar]
- Hooper J. E., Kelly R. B. Calmodulin is tightly associated with synaptic vesicles independent of calcium. J Biol Chem. 1984 Jan 10;259(1):148–153. [PubMed] [Google Scholar]
- Israël M., Manaranche R., Marsal J., Meunier F. M., Morel N., Frachon P., Lesbats B. ATP-dependent calcium uptake by cholinergic synaptic vesicles isolated from Torpedo electric organ. J Membr Biol. 1980 May 23;54(2):115–126. doi: 10.1007/BF01940565. [DOI] [PubMed] [Google Scholar]
- Jones R. T., Walker J. H., Stadler H., Whittaker V. P. Immunohistochemical localization of a synaptic-vesicle antigen in a cholinergic neuron under conditions of stimulation and rest. Cell Tissue Res. 1982;223(1):117–126. doi: 10.1007/BF00221503. [DOI] [PubMed] [Google Scholar]
- Kiene M. L., Stadler H. Synaptic vesicles in electromotoneurones. I. Axonal transport, site of transmitter uptake and processing of a core proteoglycan during maturation. EMBO J. 1987 Aug;6(8):2209–2215. doi: 10.1002/j.1460-2075.1987.tb02492.x. [DOI] [PMC free article] [PubMed] [Google Scholar]
- Lee D. A., Witzemann V. Photoaffinity labeling of a synaptic vesicle specific nucleotide transport system from Torpedo marmorata. Biochemistry. 1983 Dec 20;22(26):6123–6130. doi: 10.1021/bi00295a013. [DOI] [PubMed] [Google Scholar]
- Luqmani Y. A. Nucleotide uptake by isolated cholinergic synaptic vesicles: evidence for a carrier of adenosine 5'-triphosphate. Neuroscience. 1981;6(6):1011–1021. doi: 10.1016/0306-4522(81)90067-1. [DOI] [PubMed] [Google Scholar]
- Michaelson D. M., Ophir I., Angel I. ATP-stimulated Ca2+ transport into cholinergic Torpedo synaptic vesicles. J Neurochem. 1980 Jul;35(1):116–124. doi: 10.1111/j.1471-4159.1980.tb12496.x. [DOI] [PubMed] [Google Scholar]
- Newcombe J., Glynn P., Cuzner M. L. Analysis by transfer electrophoresis of reactivity of IgG with brain proteins in multiple sclerosis. J Neurochem. 1982 Oct;39(4):1192–1194. doi: 10.1111/j.1471-4159.1982.tb11515.x. [DOI] [PubMed] [Google Scholar]
- Ohsawa K., Dowe G. H., Morris S. J., Whittaker V. P. The lipid and protein content of cholinergic synaptic vesicles from the electric organ of Torpedo marmorata purified to constant composition: implications for vesicle structure. Brain Res. 1979 Feb 9;161(3):447–457. doi: 10.1016/0006-8993(79)90674-7. [DOI] [PubMed] [Google Scholar]
- Rephaeli A., Parsons S. M. Calmodulin stimulation of 45Ca2+ transport and protein phosphorylation in cholinergic synaptic vesicles. Proc Natl Acad Sci U S A. 1982 Oct;79(19):5783–5787. doi: 10.1073/pnas.79.19.5783. [DOI] [PMC free article] [PubMed] [Google Scholar]
- Stadler H., Dowe G. H. Identification of a heparan sulphate-containing proteoglycan as a specific core component of cholinergic synaptic vesicles from Torpedo marmorata. EMBO J. 1982;1(11):1381–1384. doi: 10.1002/j.1460-2075.1982.tb01326.x. [DOI] [PMC free article] [PubMed] [Google Scholar]
- Stadler H., Fenwick E. M. Cholinergic synaptic vesicles from Torpedo marmorata contain an atractyloside-binding protein related to the mitochondrial ADP/ATP carrier. Eur J Biochem. 1983 Nov 2;136(2):377–382. doi: 10.1111/j.1432-1033.1983.tb07752.x. [DOI] [PubMed] [Google Scholar]
- Tashiro T., Stadler H. Chemical composition of cholinergic synaptic vesicles from Torpedo marmorata based on improved purification. Eur J Biochem. 1978 Oct 16;90(3):479–487. doi: 10.1111/j.1432-1033.1978.tb12627.x. [DOI] [PubMed] [Google Scholar]
- Torri-Tarelli F., Grohovaz F., Fesce R., Ceccarelli B. Temporal coincidence between synaptic vesicle fusion and quantal secretion of acetylcholine. J Cell Biol. 1985 Oct;101(4):1386–1399. doi: 10.1083/jcb.101.4.1386. [DOI] [PMC free article] [PubMed] [Google Scholar]
- Walker J. H., Stadler H., Witzemann V. Calmodulin binding proteins of the cholinergic electromotor synapse: synaptosomes, synaptic vesicles, receptor-enriched membranes, and cytoskeleton. J Neurochem. 1984 Feb;42(2):314–320. doi: 10.1111/j.1471-4159.1984.tb02680.x. [DOI] [PubMed] [Google Scholar]
- Witzemann V. Characterization of multiple forms of acetylcholinesterase in electric organ of Torpedo marmorata. Neurosci Lett. 1980 Dec;20(3):277–282. doi: 10.1016/0304-3940(80)90160-3. [DOI] [PubMed] [Google Scholar]
- Yanagishita M., Hascall V. C. Metabolism of proteoglycans in rat ovarian granulosa cell culture. Multiple intracellular degradative pathways and the effect of chloroquine. J Biol Chem. 1984 Aug 25;259(16):10270–10283. [PubMed] [Google Scholar]
- Zimmermann H., Denston C. R. Separation of synaptic vesicles of different functional states from the cholinergic synapses of the Torpedo electric organ. Neuroscience. 1977;2(5):715–730. doi: 10.1016/0306-4522(77)90025-2. [DOI] [PubMed] [Google Scholar]
- Zimmermann H. Vesicle recycling and transmitter release. Neuroscience. 1979;4(12):1773–1804. doi: 10.1016/0306-4522(79)90058-7. [DOI] [PubMed] [Google Scholar]
- Zimmermann H., Whittaker V. P. Morphological and biochemical heterogeneity of cholinergic synaptic vesicles. Nature. 1977 Jun 16;267(5612):633–635. doi: 10.1038/267633a0. [DOI] [PubMed] [Google Scholar]