Abstract
Mass spectrometry has been used to confirm the presence of an active transport system for CO2 in Synechococcus UTEX 625. Cells were incubated at pH 8.0 in 100 micromolar KHCO3 in the absence of Na+ (to prevent HCO3− transport). Upon illumination the cells rapidly removed almost all the free CO2 from the medium. Addition of carbonic anhydrase revealed that the CO2 depletion resulted from a selective uptake of CO2, rather than a total uptake of all inorganic carbon species. CO2 transport stopped rapidly (<3 seconds) when the light was turned off. Iodoacetamide (3.3 millimolar) completely inhibited CO2 fixation but had little effect on CO2 transport. In iodoacetamide poisoned cells, transport of CO2 occurred against a concentration gradient of about 18,000 to 1. Transport of CO2 was completely inhibited by 10 micromolar diethylstilbestrol, a membrane-bound ATPase inhibitor. Studies with DCMU and PSI light indicated that CO2 transport was driven by ATP produced by cyclic or pseudocyclic photophosphorylation. Low concentrations of Na+ (<100 microequivalents per liter), but not of K+, stimulated CO2 transport as much as 2.4-fold. Unlike Na+-dependent HCO3− transport, the transport of CO2 was not inhibited by high concentrations (30 milliequivalents per liter) of Li+. During illumination, the CO2 concentration in the medium remained far below its equilibrium value for periods up to 15 minutes. This could only happen if CO2 transport was continuously occurring at a rapid rate, since the continuing dehydration of HCO3− to CO2 would rapidly raise the CO2 concentration to its equilibrium value if transport ceased. Measurement of the rate of dissolved inorganic carbon accumulation under these conditions indicated that at least part of the continuing CO2 transport was balanced by HCO3− efflux.
Full text
PDF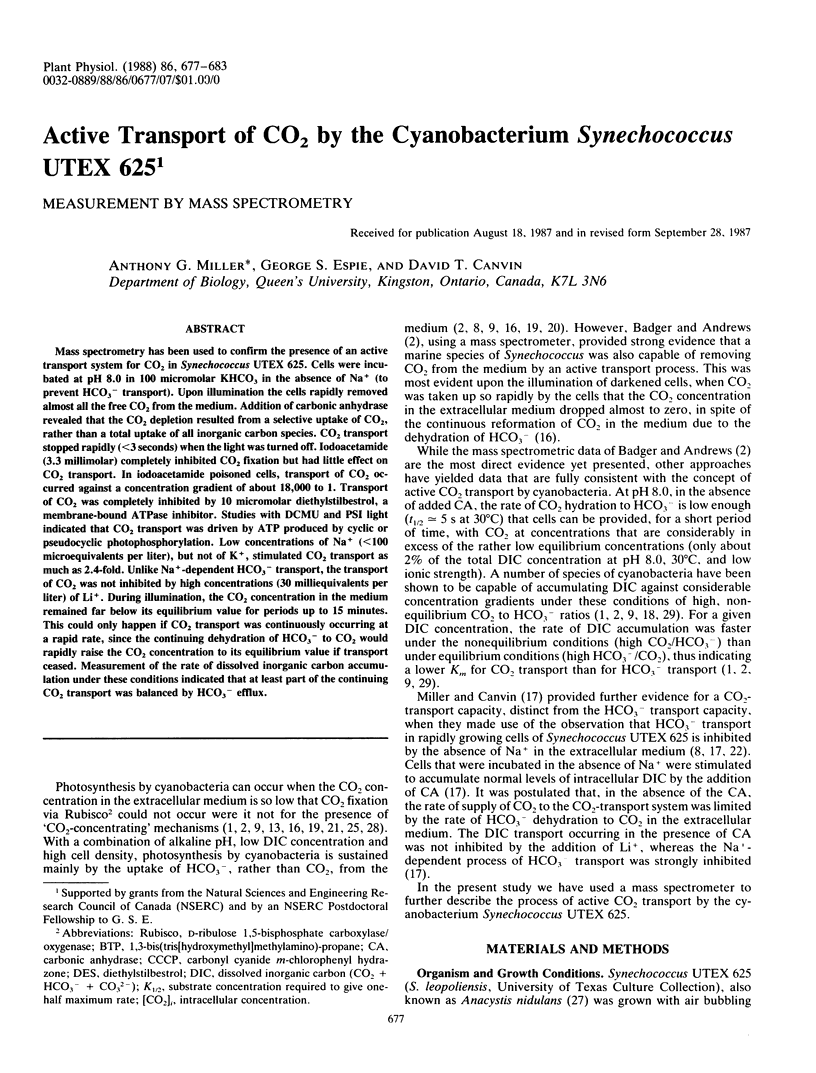
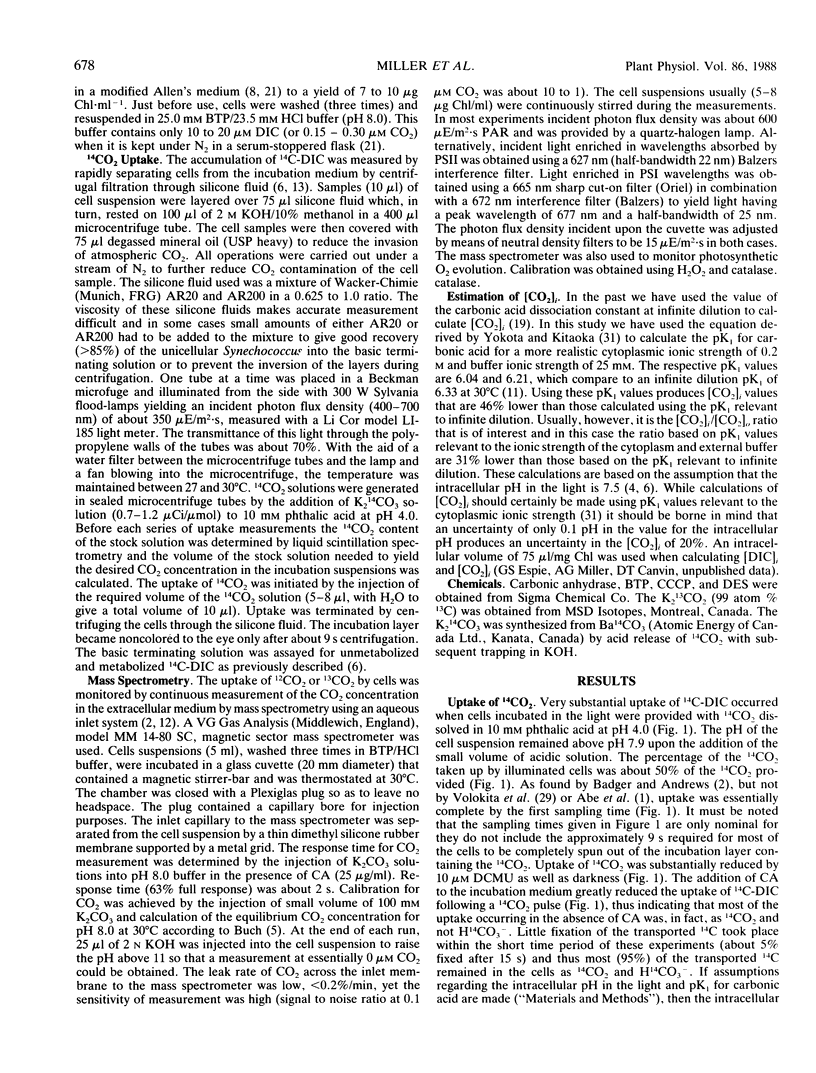
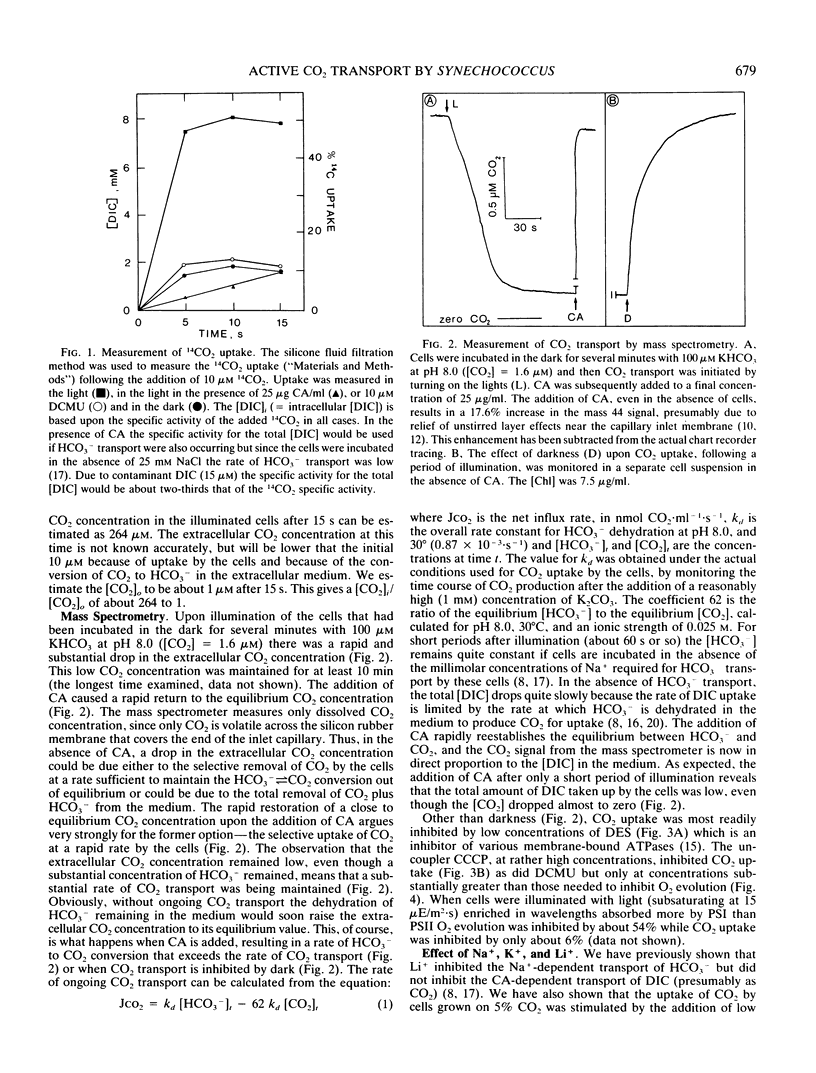
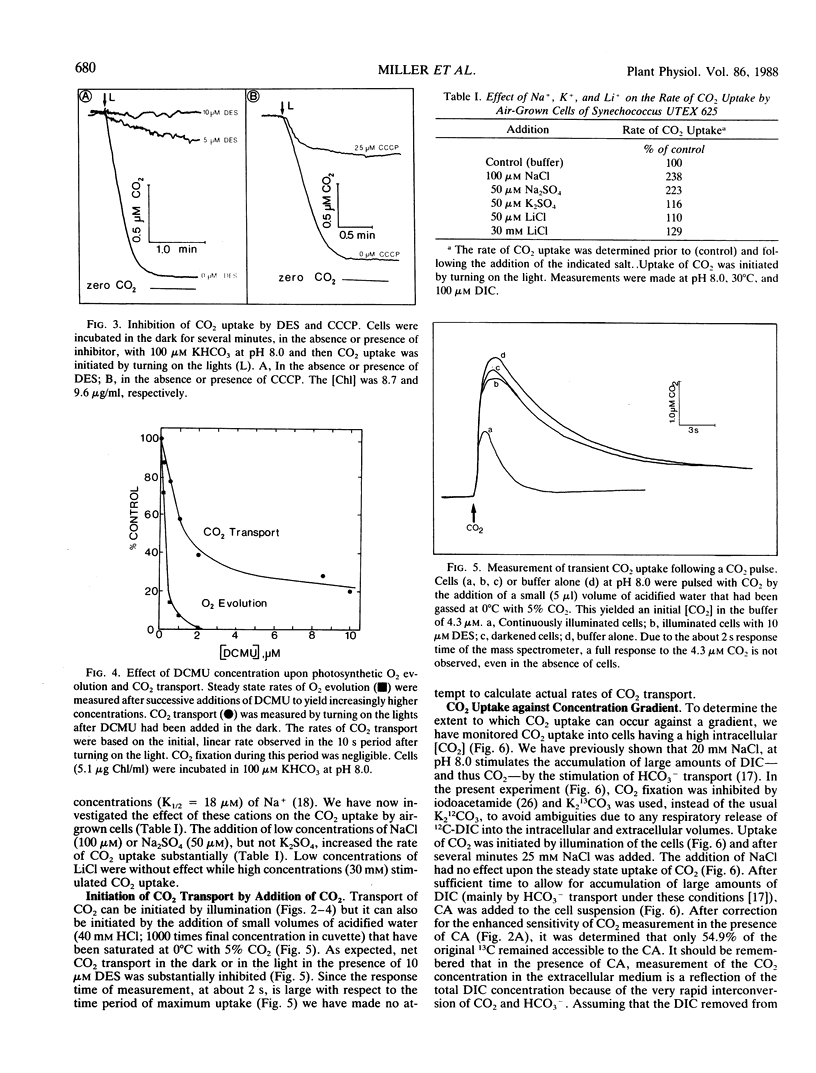
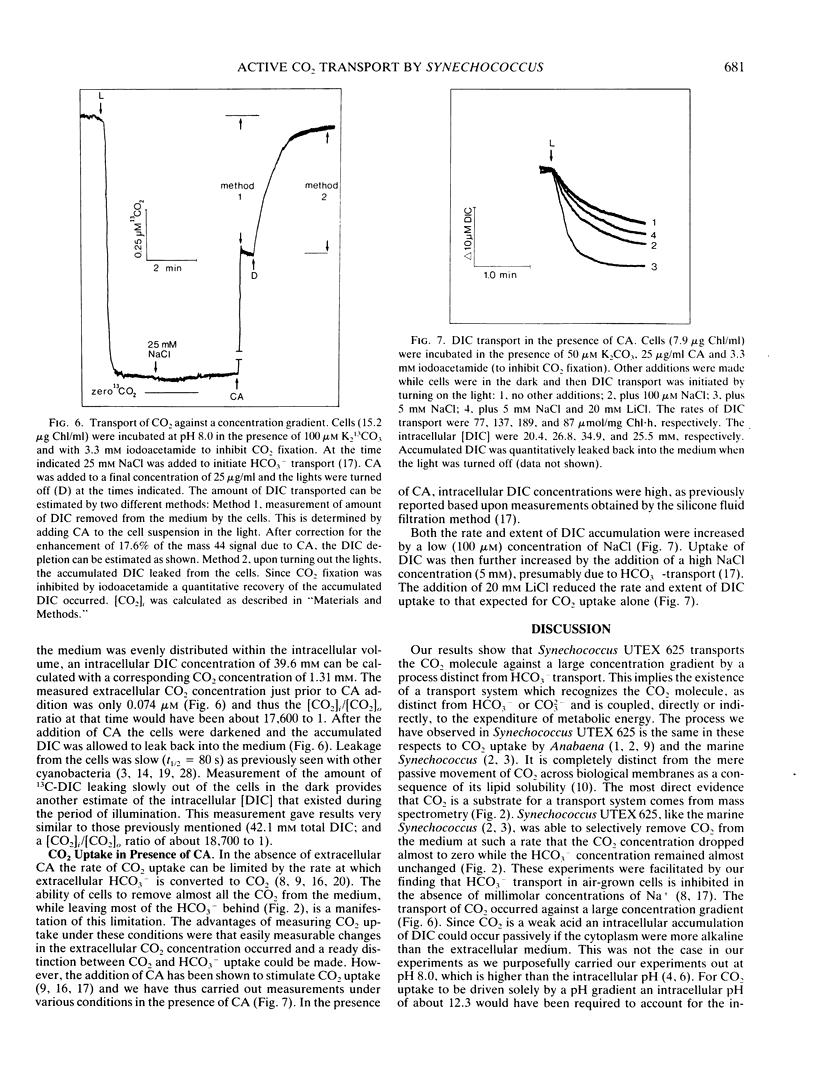
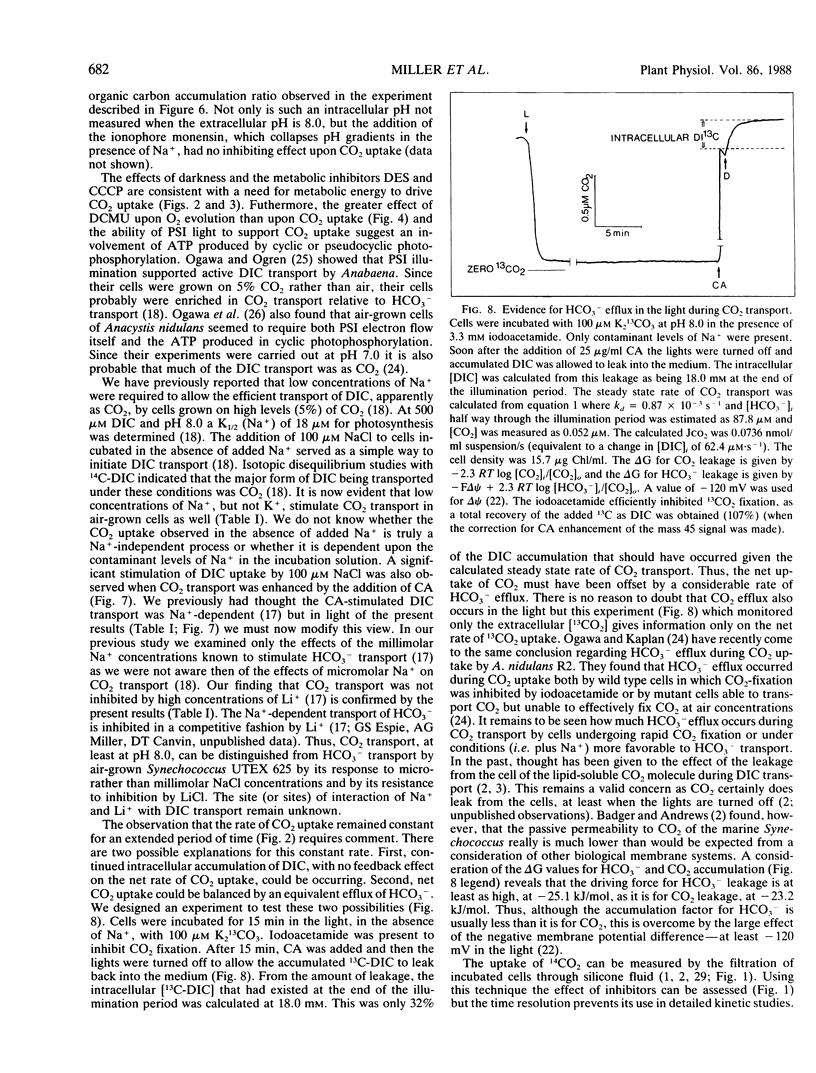
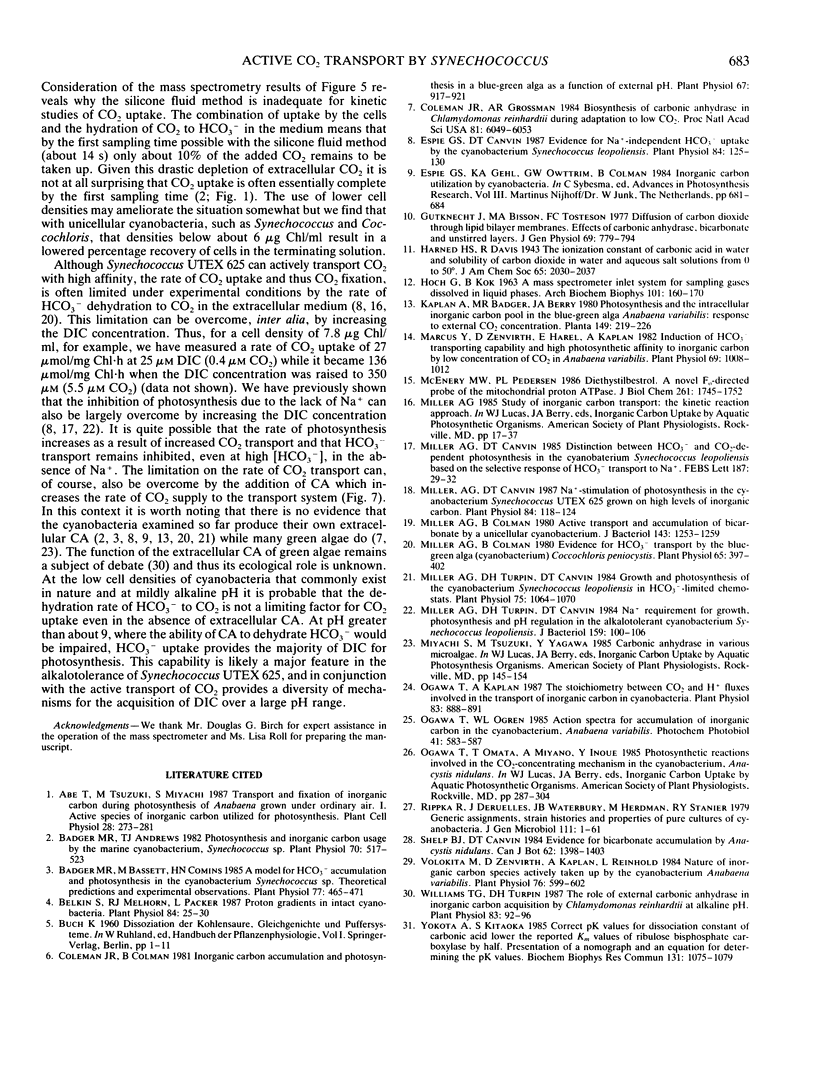
Selected References
These references are in PubMed. This may not be the complete list of references from this article.
- Badger M. R., Andrews T. J. Photosynthesis and Inorganic Carbon Usage by the Marine Cyanobacterium, Synechococcus sp. Plant Physiol. 1982 Aug;70(2):517–523. doi: 10.1104/pp.70.2.517. [DOI] [PMC free article] [PubMed] [Google Scholar]
- Badger M. R., Bassett M., Comins H. N. A Model for HCO(3) Accumulation and Photosynthesis in the Cyanobacterium Synechococcus sp: Theoretical Predictions and Experimental Observations. Plant Physiol. 1985 Feb;77(2):465–471. doi: 10.1104/pp.77.2.465. [DOI] [PMC free article] [PubMed] [Google Scholar]
- Belkin S., Mehlhorn R. J., Packer L. Proton gradients in intact cyanobacteria. Plant Physiol. 1987;84:25–30. doi: 10.1104/pp.84.1.25. [DOI] [PMC free article] [PubMed] [Google Scholar]
- Coleman J. R., Colman B. Inorganic Carbon Accumulation and Photosynthesis in a Blue-green Alga as a Function of External pH. Plant Physiol. 1981 May;67(5):917–921. doi: 10.1104/pp.67.5.917. [DOI] [PMC free article] [PubMed] [Google Scholar]
- Coleman J. R., Grossman A. R. Biosynthesis of carbonic anhydrase in Chlamydomonas reinhardtii during adaptation to low CO(2). Proc Natl Acad Sci U S A. 1984 Oct;81(19):6049–6053. doi: 10.1073/pnas.81.19.6049. [DOI] [PMC free article] [PubMed] [Google Scholar]
- Espie G. S., Canvin D. T. Evidence for Na-Independent HCO(3) Uptake by the Cyanobacterium Synechococcus leopoliensis. Plant Physiol. 1987 May;84(1):125–130. doi: 10.1104/pp.84.1.125. [DOI] [PMC free article] [PubMed] [Google Scholar]
- Gutknecht J., Bisson M. A., Tosteson F. C. Diffusion of carbon dioxide through lipid bilayer membranes: effects of carbonic anhydrase, bicarbonate, and unstirred layers. J Gen Physiol. 1977 Jun;69(6):779–794. doi: 10.1085/jgp.69.6.779. [DOI] [PMC free article] [PubMed] [Google Scholar]
- HOCH G., KOK B. A mass spectrometer inlet system for sampling gases dissolved in liquid phases. Arch Biochem Biophys. 1963 Apr;101:160–170. doi: 10.1016/0003-9861(63)90546-0. [DOI] [PubMed] [Google Scholar]
- Marcus Y., Zenvirth D., Harel E., Kaplan A. Induction of HCO(3) Transporting Capability and High Photosynthetic Affinity to Inorganic Carbon by Low Concentration of CO(2) in Anabaena variabilis. Plant Physiol. 1982 May;69(5):1008–1012. doi: 10.1104/pp.69.5.1008. [DOI] [PMC free article] [PubMed] [Google Scholar]
- McEnery M. W., Pedersen P. L. Diethylstilbestrol. A novel F0-directed probe of the mitochondrial proton ATPase. J Biol Chem. 1986 Feb 5;261(4):1745–1752. [PubMed] [Google Scholar]
- Miller A. G., Canvin D. T. Na-Stimulation of Photosynthesis in the Cyanobacterium Synechococcus UTEX 625 Grown on High Levels of Inorganic Carbon. Plant Physiol. 1987 May;84(1):118–124. doi: 10.1104/pp.84.1.118. [DOI] [PMC free article] [PubMed] [Google Scholar]
- Miller A. G., Colman B. Active transport and accumulation of bicarbonate by a unicellular cyanobacterium. J Bacteriol. 1980 Sep;143(3):1253–1259. doi: 10.1128/jb.143.3.1253-1259.1980. [DOI] [PMC free article] [PubMed] [Google Scholar]
- Miller A. G., Colman B. Evidence for HCO(3) Transport by the Blue-Green Alga (Cyanobacterium) Coccochloris peniocystis. Plant Physiol. 1980 Feb;65(2):397–402. doi: 10.1104/pp.65.2.397. [DOI] [PMC free article] [PubMed] [Google Scholar]
- Miller A. G., Turpin D. H., Canvin D. T. Growth and Photosynthesis of the Cyanobacterium Synechococcus leopoliensis in HCO(3)-Limited Chemostats. Plant Physiol. 1984 Aug;75(4):1064–1070. doi: 10.1104/pp.75.4.1064. [DOI] [PMC free article] [PubMed] [Google Scholar]
- Miller A. G., Turpin D. H., Canvin D. T. Na+ requirement for growth, photosynthesis, and pH regulation in the alkalotolerant cyanobacterium Synechococcus leopoliensis. J Bacteriol. 1984 Jul;159(1):100–106. doi: 10.1128/jb.159.1.100-106.1984. [DOI] [PMC free article] [PubMed] [Google Scholar]
- Ogawa T., Kaplan A. The Stoichiometry between CO(2) and H Fluxes Involved in the Transport of Inorganic Carbon in Cyanobacteria. Plant Physiol. 1987 Apr;83(4):888–891. doi: 10.1104/pp.83.4.888. [DOI] [PMC free article] [PubMed] [Google Scholar]
- Volokita M., Zenvirth D., Kaplan A., Reinhold L. Nature of the Inorganic Carbon Species Actively Taken Up by the Cyanobacterium Anabaena variabilis. Plant Physiol. 1984 Nov;76(3):599–602. doi: 10.1104/pp.76.3.599. [DOI] [PMC free article] [PubMed] [Google Scholar]
- Williams T. G., Turpin D. H. The Role of External Carbonic Anhydrase in Inorganic Carbon Acquisition by Chlamydomonas reinhardii at Alkaline pH. Plant Physiol. 1987 Jan;83(1):92–96. doi: 10.1104/pp.83.1.92. [DOI] [PMC free article] [PubMed] [Google Scholar]
- Yokota A., Kitaoka S. Correct pK values for dissociation constant of carbonic acid lower the reported Km values of ribulose bisphosphate carboxylase to half. Presentation of a nomograph and an equation for determining the pK values. Biochem Biophys Res Commun. 1985 Sep 30;131(3):1075–1079. doi: 10.1016/0006-291x(85)90200-1. [DOI] [PubMed] [Google Scholar]