Abstract
Proplastids and etioplasts are common starting points for monitoring chloroplast development in higher plants. Although proplastids are the primary precursor of chloroplasts, most proplastid to chloroplast systems are cumbersome to study temporally. Conversely, the etioplast to chloroplast transition is initiated by light and is readily examined as a function of time. Etioplasts, however, are found mostly in plants germinated in the dark and are not an obligatory step in chloroplast development. We have chosen to study chloroplast ontogeny in Spirodela oligorrhiza (Kurtz) Hegelm (a C3-monocot) because of its unique ability to grow indefinitely in the dark. Ultrastructural, physiological, and molecular evidence is presented in support of a temporal, light-triggered proplastid to chloroplast transition in Spirodela. The dark-grown plants are devoid of chlorophyll, and upon illumination synchronously green over a 3- to 5-day period. Synthesis of chloroplast proteins involved in photosynthesis is coincident with thylakoid assembly, chlorophyll accumulation, and appearance of CO2 fixation activity. Interestingly, the developmental sequence in Spirodela was slow enough to reveal that biosynthesis of the D1 photosystem II reaction center protein precedes biosynthesis of the major light-harvesting antenna proteins. This, coupled with the high chlorophyll a/b ratio observed early in development, indicated that reaction center assembly occurred prior to accumulation of the light-harvesting complexes. Thus, with Spirodela one can study proplastid to chloroplast conversions temporally in higher plants and follow the process on a time scale that enables a detailed dissection of plastid maturation processes.
Full text
PDF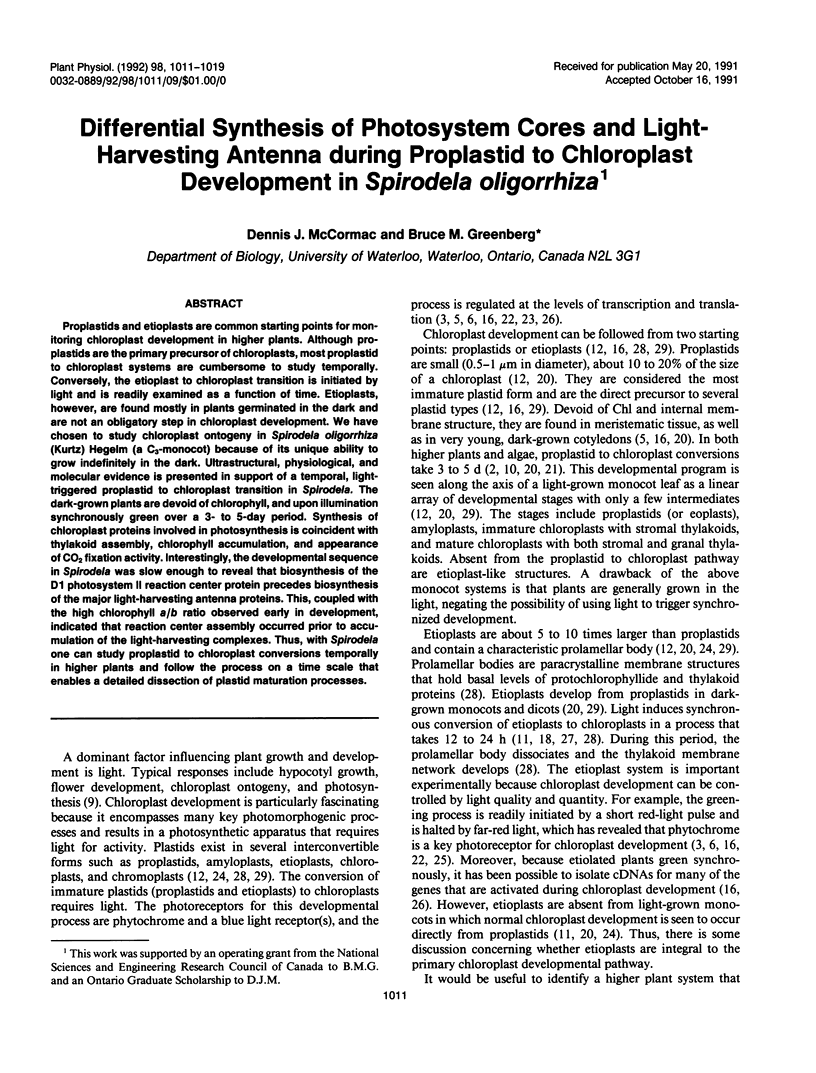
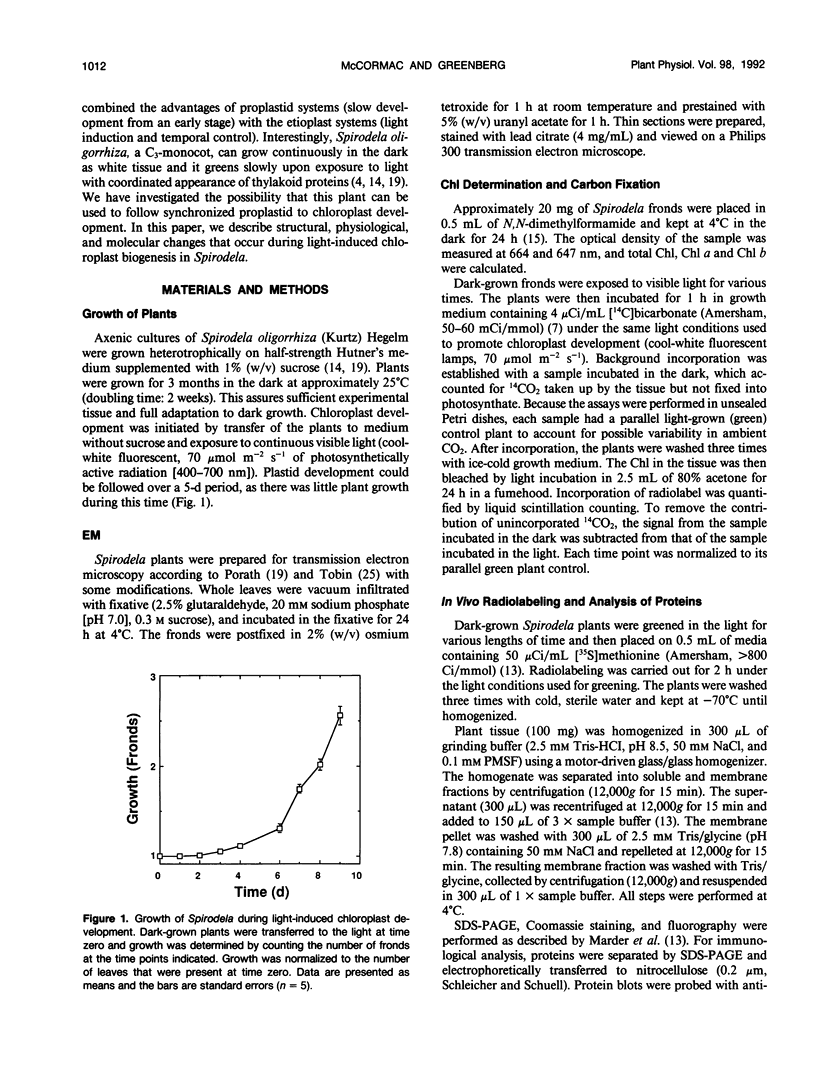
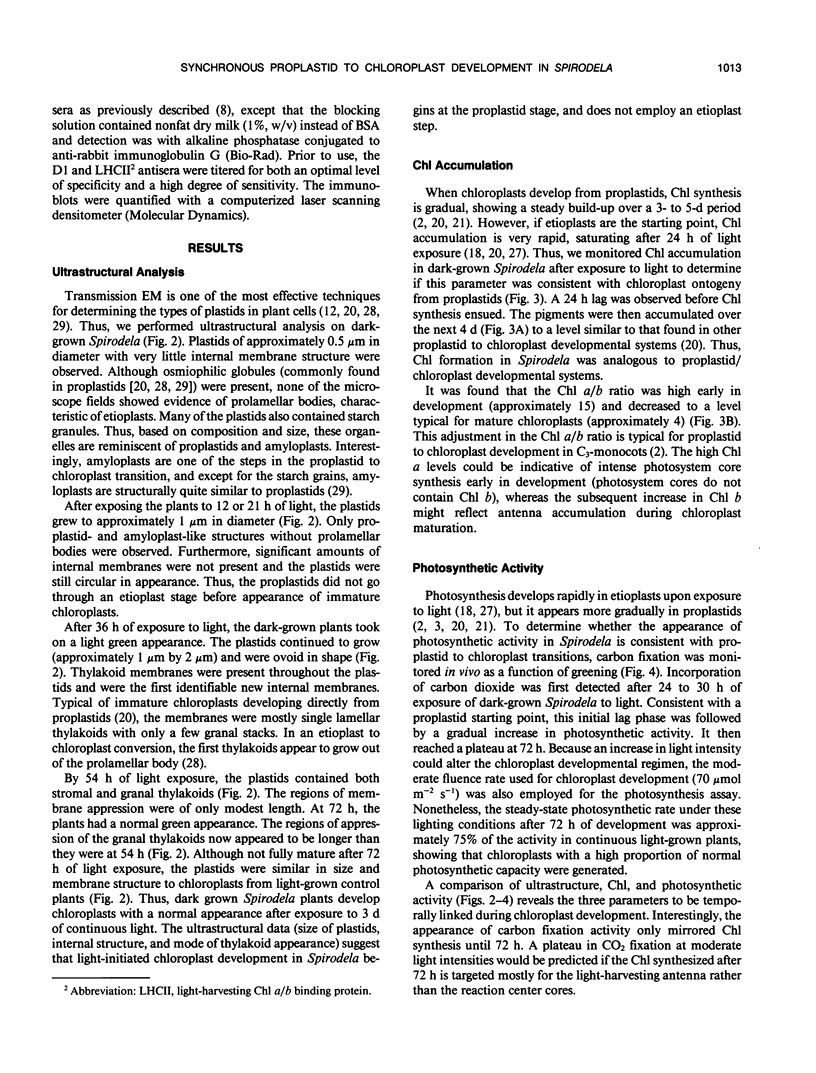
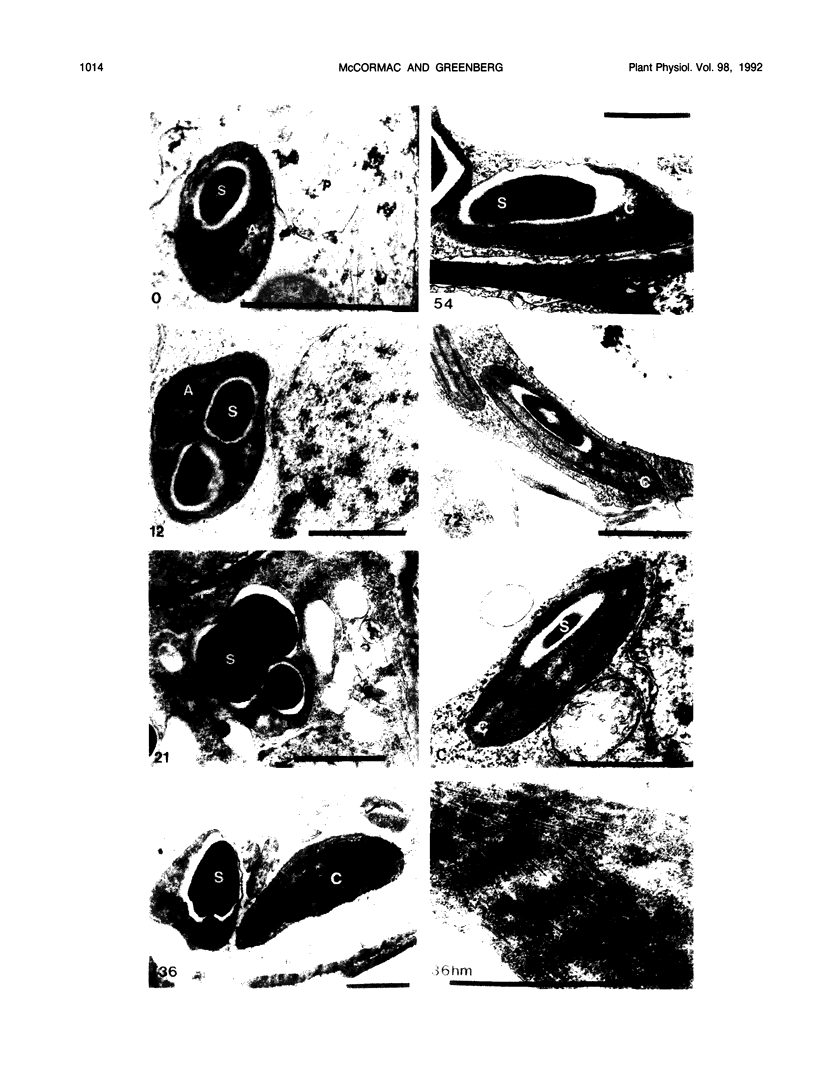
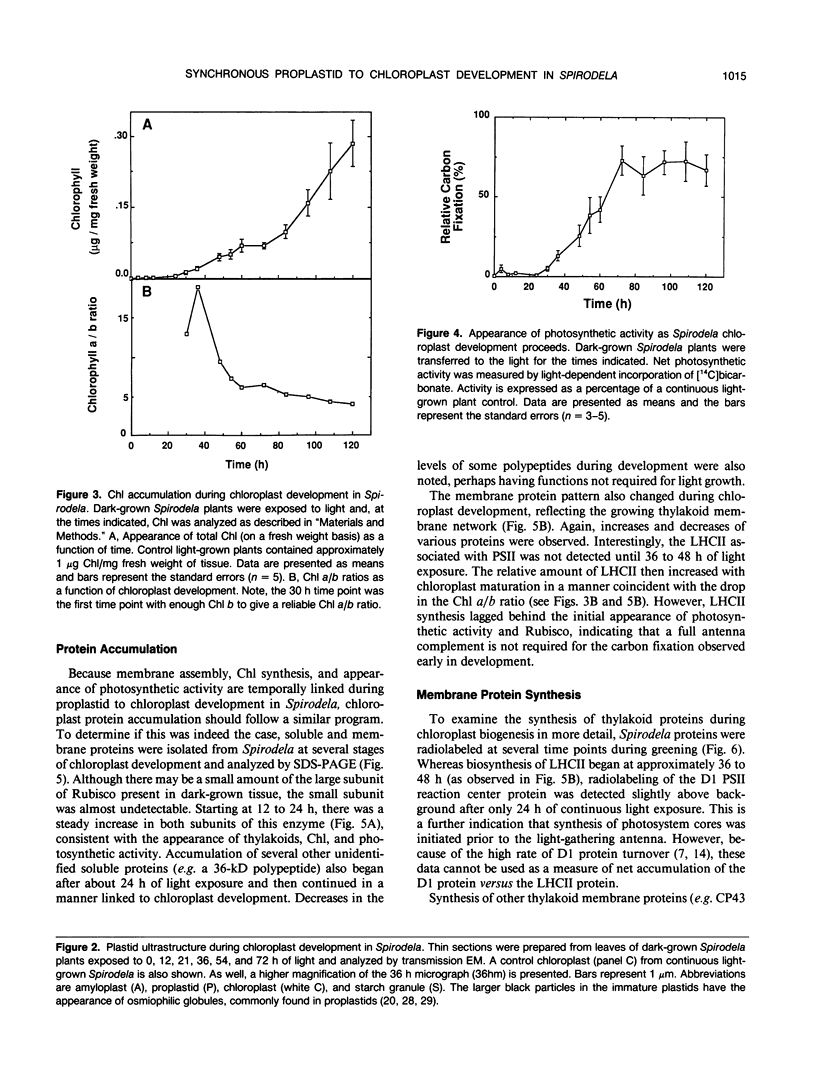
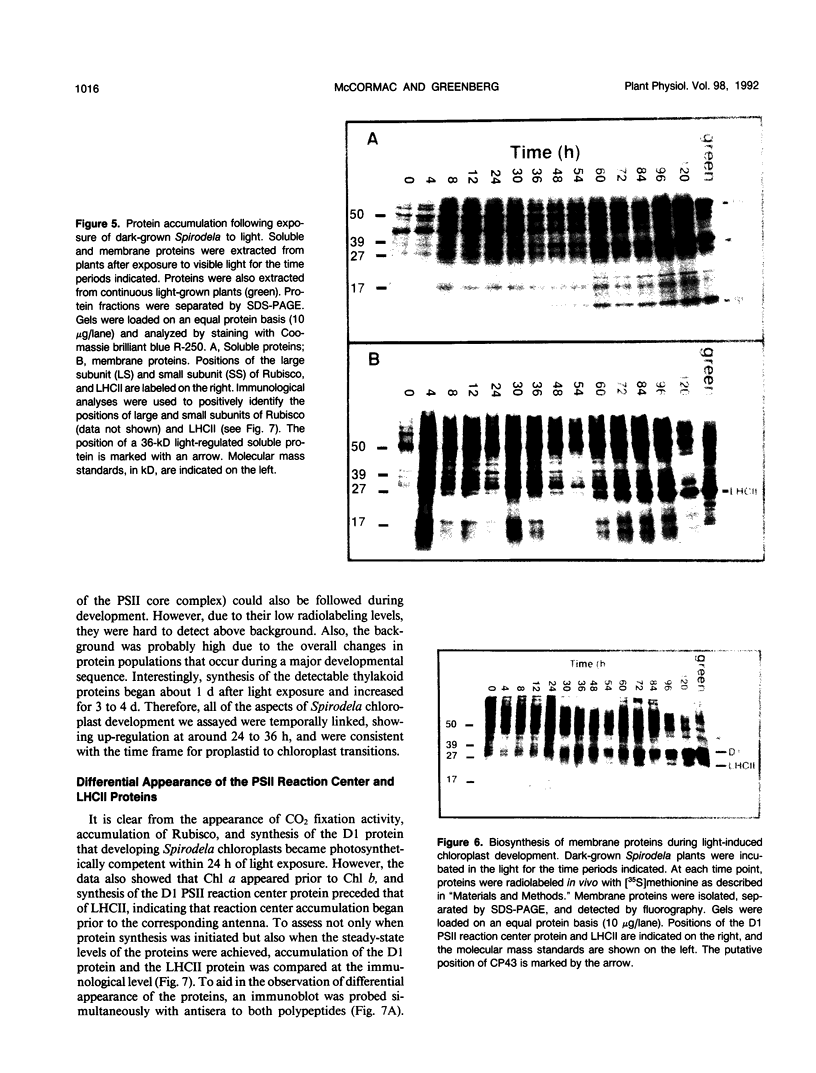
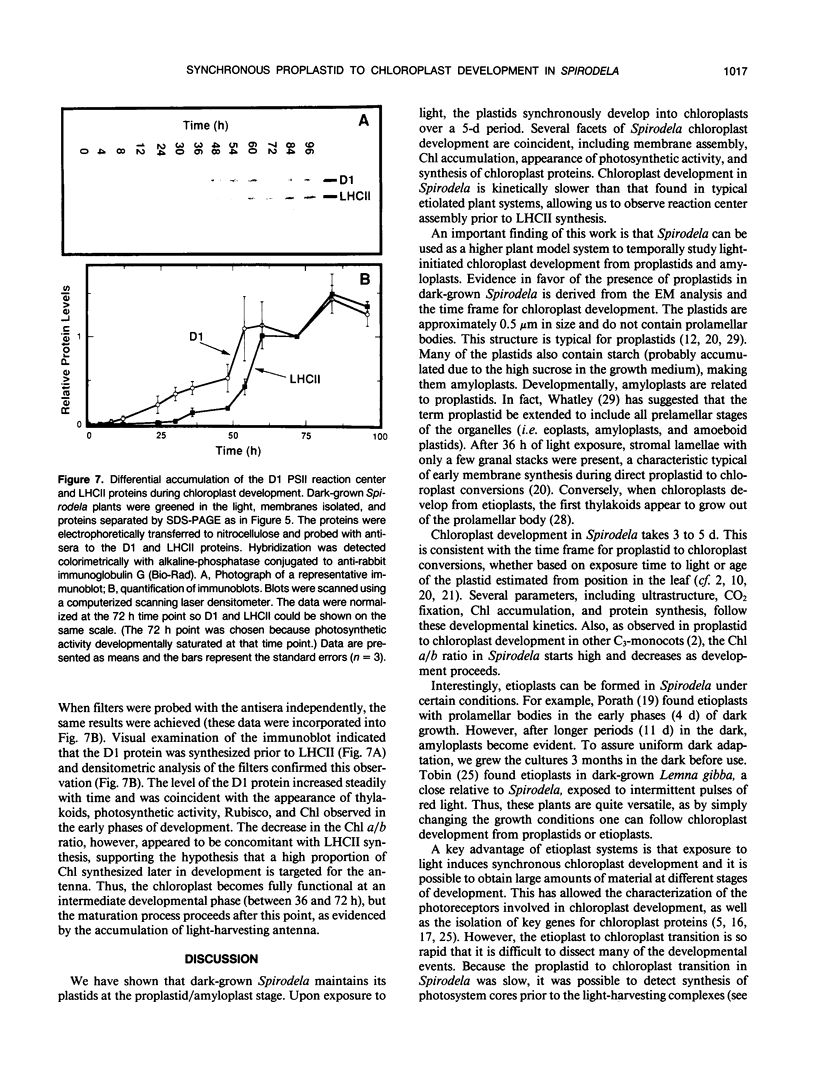
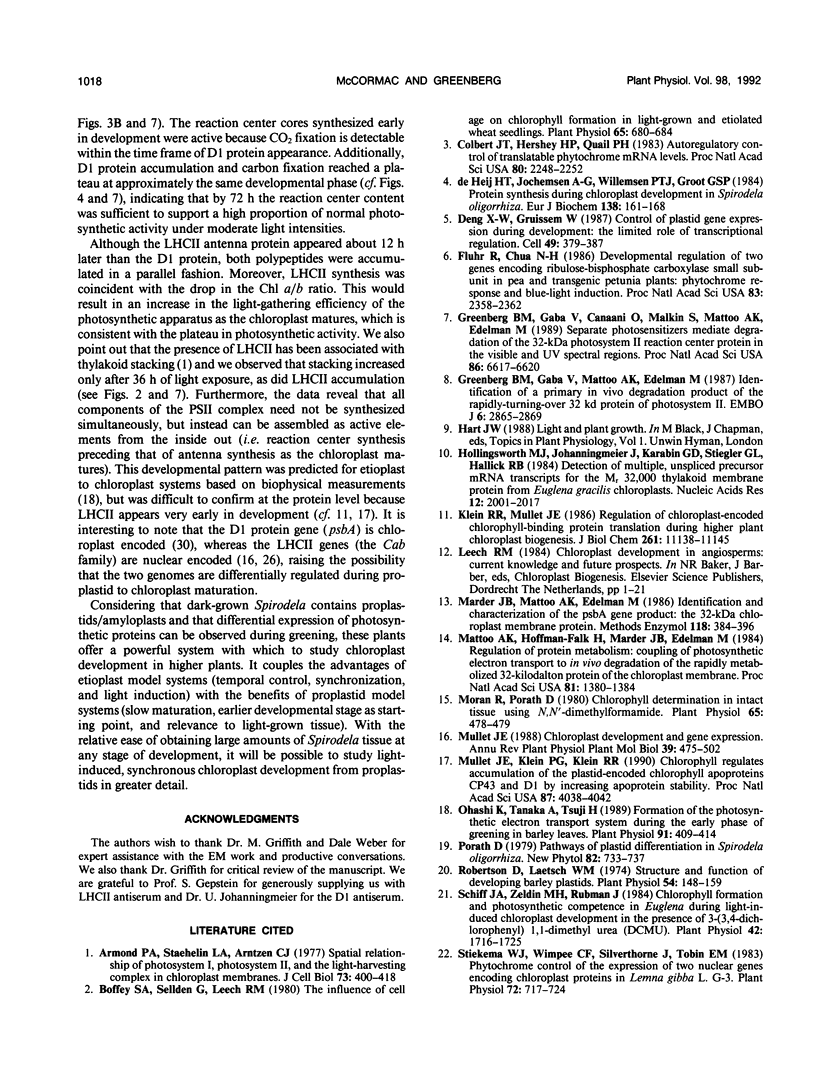
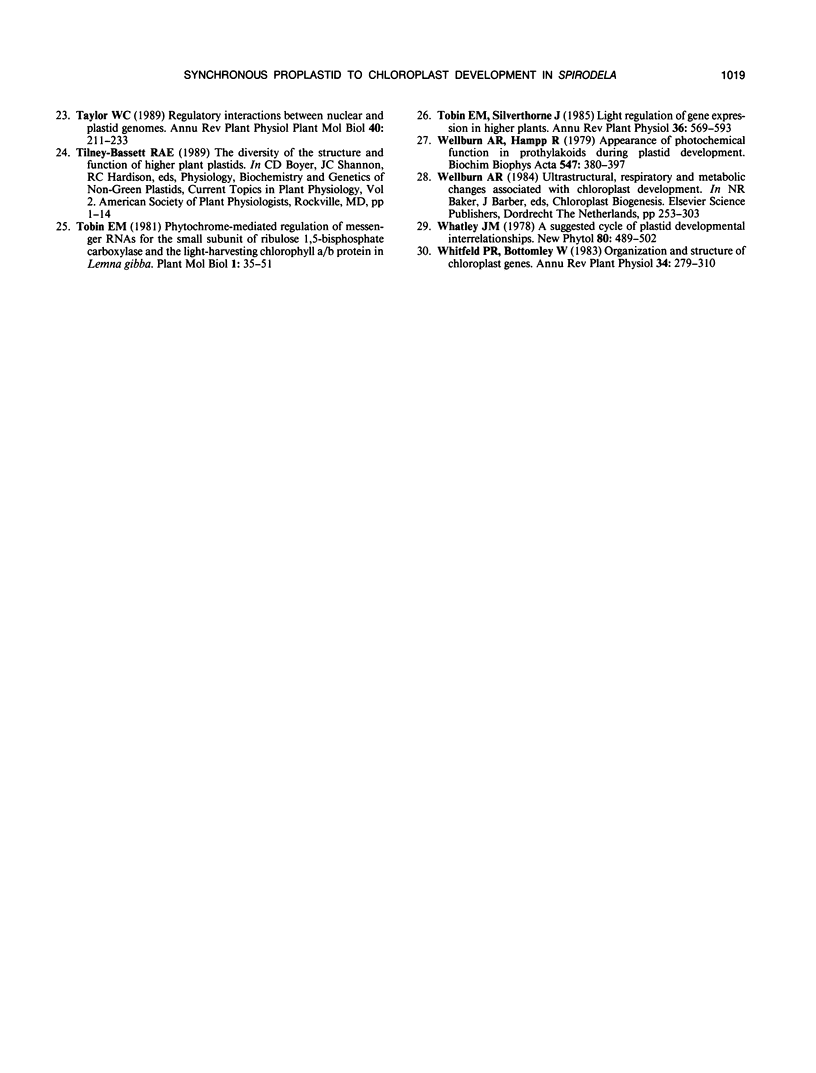
Images in this article
Selected References
These references are in PubMed. This may not be the complete list of references from this article.
- Armond P. A., Staehelin L. A., Arntzen C. J. Spatial relationship of photosystem I, photosystem II, and the light-harvesting complex in chloroplast membranes. J Cell Biol. 1977 May;73(2):400–418. doi: 10.1083/jcb.73.2.400. [DOI] [PMC free article] [PubMed] [Google Scholar]
- Boffey S. A., Selldén G., Leech R. M. Influence of Cell Age on Chlorophyll Formation in Light-grown and Etiolated Wheat Seedlings. Plant Physiol. 1980 Apr;65(4):680–684. doi: 10.1104/pp.65.4.680. [DOI] [PMC free article] [PubMed] [Google Scholar]
- Colbert J. T., Hershey H. P., Quail P. H. Autoregulatory control of translatable phytochrome mRNA levels. Proc Natl Acad Sci U S A. 1983 Apr;80(8):2248–2252. doi: 10.1073/pnas.80.8.2248. [DOI] [PMC free article] [PubMed] [Google Scholar]
- Deng X. W., Gruissem W. Control of plastid gene expression during development: the limited role of transcriptional regulation. Cell. 1987 May 8;49(3):379–387. doi: 10.1016/0092-8674(87)90290-x. [DOI] [PubMed] [Google Scholar]
- Fluhr R., Chua N. H. Developmental regulation of two genes encoding ribulose-bisphosphate carboxylase small subunit in pea and transgenic petunia plants: Phytochrome response and blue-light induction. Proc Natl Acad Sci U S A. 1986 Apr;83(8):2358–2362. doi: 10.1073/pnas.83.8.2358. [DOI] [PMC free article] [PubMed] [Google Scholar]
- Greenberg B. M., Gaba V., Canaani O., Malkin S., Mattoo A. K., Edelman M. Separate photosensitizers mediate degradation of the 32-kDa photosystem II reaction center protein in the visible and UV spectral regions. Proc Natl Acad Sci U S A. 1989 Sep;86(17):6617–6620. doi: 10.1073/pnas.86.17.6617. [DOI] [PMC free article] [PubMed] [Google Scholar]
- Greenberg B. M., Gaba V., Mattoo A. K., Edelman M. Identification of a primary in vivo degradation product of the rapidly-turning-over 32 kd protein of photosystem II. EMBO J. 1987 Oct;6(10):2865–2869. doi: 10.1002/j.1460-2075.1987.tb02588.x. [DOI] [PMC free article] [PubMed] [Google Scholar]
- Hollingsworth M. J., Johanningmeier U., Karabin G. D., Stiegler G. L., Hallick R. B. Detection of multiple, unspliced precursor mRNA transcripts for the Mr 32,000 thylakoid membrane protein from Euglena gracilis chloroplasts. Nucleic Acids Res. 1984 Feb 24;12(4):2001–2017. doi: 10.1093/nar/12.4.2001. [DOI] [PMC free article] [PubMed] [Google Scholar]
- Klein R. R., Mullet J. E. Regulation of chloroplast-encoded chlorophyll-binding protein translation during higher plant chloroplast biogenesis. J Biol Chem. 1986 Aug 25;261(24):11138–11145. [PubMed] [Google Scholar]
- Mattoo A. K., Hoffman-Falk H., Marder J. B., Edelman M. Regulation of protein metabolism: Coupling of photosynthetic electron transport to in vivo degradation of the rapidly metabolized 32-kilodalton protein of the chloroplast membranes. Proc Natl Acad Sci U S A. 1984 Mar;81(5):1380–1384. doi: 10.1073/pnas.81.5.1380. [DOI] [PMC free article] [PubMed] [Google Scholar]
- Moran R., Porath D. Chlorophyll determination in intact tissues using n,n-dimethylformamide. Plant Physiol. 1980 Mar;65(3):478–479. doi: 10.1104/pp.65.3.478. [DOI] [PMC free article] [PubMed] [Google Scholar]
- Mullet J. E., Klein P. G., Klein R. R. Chlorophyll regulates accumulation of the plastid-encoded chlorophyll apoproteins CP43 and D1 by increasing apoprotein stability. Proc Natl Acad Sci U S A. 1990 Jun;87(11):4038–4042. doi: 10.1073/pnas.87.11.4038. [DOI] [PMC free article] [PubMed] [Google Scholar]
- Ohashi K., Tanaka A., Tsuji H. Formation of the Photosynthetic Electron Transport System during the Early Phase of Greening in Barley Leaves. Plant Physiol. 1989 Sep;91(1):409–414. doi: 10.1104/pp.91.1.409. [DOI] [PMC free article] [PubMed] [Google Scholar]
- Robertson D., Laetsch W. M. Structure and function of developing barley plastids. Plant Physiol. 1974 Aug;54(2):148–159. doi: 10.1104/pp.54.2.148. [DOI] [PMC free article] [PubMed] [Google Scholar]
- Schiff J. A., Zeldin M. H., Rubman J. Chlorophyll Formation and Photosynthetic Competence in Euglena During Light-Induced Chloroplast Development in the Presence of 3, (3,4-dichlorophenyl) 1,1-Dimethyl Urea (DCMU). Plant Physiol. 1967 Dec;42(12):1716–1725. doi: 10.1104/pp.42.12.1716. [DOI] [PMC free article] [PubMed] [Google Scholar]
- Stiekema W. J., Wimpee C. F., Silverthorne J., Tobin E. M. Phytochrome Control of the Expression of Two Nuclear Genes Encoding Chloroplast Proteins in Lemna gibba L. G-3. Plant Physiol. 1983 Jul;72(3):717–724. doi: 10.1104/pp.72.3.717. [DOI] [PMC free article] [PubMed] [Google Scholar]
- Wellburn A. R., Hampp R. Appearance of photochemical function in prothylakoids during plastid development. Biochim Biophys Acta. 1979 Aug 14;547(2):380–397. doi: 10.1016/0005-2728(79)90019-7. [DOI] [PubMed] [Google Scholar]
- de Heij H. T., Jochemsen A. G., Willemsen P. T., Groot G. S. Protein synthesis during chloroplast development in Spirodela oligorhiza. Coordinated synthesis of chloroplast-encoded and nuclear-encoded subunits of ATPase and ribulose-1,5-bisphosphate carboxylase. Eur J Biochem. 1984 Jan 2;138(1):161–168. doi: 10.1111/j.1432-1033.1984.tb07895.x. [DOI] [PubMed] [Google Scholar]