Abstract
1. Cloned large-conductance Ca(2+)-activated K+ channels from Drosophila (dslo) and human (hslo) were expressed in Xenopus oocytes. The effects of Ca2+ and voltage on these channels were compared by analysing both macroscopic currents and single-channel transitions. 2. The activation kinetics of dslo Ca(2+)-activated K+ channels are strongly influenced by the intracellular Ca2+ concentration, but are only minimally affected by membrane voltage. Current activation kinetics increase more than 60-fold in response to Ca2+ concentration increases in the range 0.56-405 microM, but increase less than 2-fold by voltage changes from -60 to +80 mV. 3. The activation kinetics of hslo channels are similarly influenced by increases in Ca2+ concentration; however, these kinetics are also increased 5- to 10-fold by voltage changes from -60 to +80 mV. 4. The deactivation kinetics of both dslo and hslo channels are also more Ca2+ sensitive than voltage sensitive. Increasing concentrations of Ca2+ slow deactivation kinetics more than 40-fold, while changes in the membrane voltage cause less than 2-fold changes. 5. Ca2+ increases the activation kinetics by altering first latency distributions. Increasing the Ca2+ concentration from 0.56 to 2.4 microM causes a 20-fold decrease in the mean time to first channel opening. 6. Both Ca2+ and voltage have large effects on regulating the steady-state open probability of these ion channels. Plots relating open probability (Po) to membrane voltage show a voltage dependence of 16.5 mV per e-fold change in Po for dslo and 12.3 mV per e-fold change in Po for hslo. At any given voltage the Ca2+ sensitivity of dslo is lower than that for hslo. The Hill coefficient for Ca2+ activation is 1.9 +/- 0.15, indicating that the binding of at least two Ca2+ ions is required to maximally activate both dslo and hslo channels. 7. The gating kinetics of both dslo and hslo channels can be well described by three open and five closed states. Changing the free Ca2+ concentration alters the time constants for the three longest closed states, without affecting any of the open states. Changing the membrane voltage alters the same three closed states, as well as the longest of the three open states. The two shortest occupancy open and closed time constants underlying these states are largely independent of voltage and Ca2+. 8. To account for these data, we propose that Ca2+ binding to the closed channel is the slow rate-limiting step in the activation pathway and, conversely, that Ca2+ unbinding is the slow rate-limiting step in the deactivation pathway. Hence, Ca2+ appears to bind to the closed channel and allows it to undergo a number of slow conformational changes that bring the channel to a state from which it can quickly open upon depolarization. These data imply that while both Ca2+ and voltage can alter the steady-state open probability of these channels, only Ca2+ has large effects on altering non-steady-state parameters and thus is the intracellular signal that predominantly modulates the rate of channel activation and deactivation.
Full text
PDF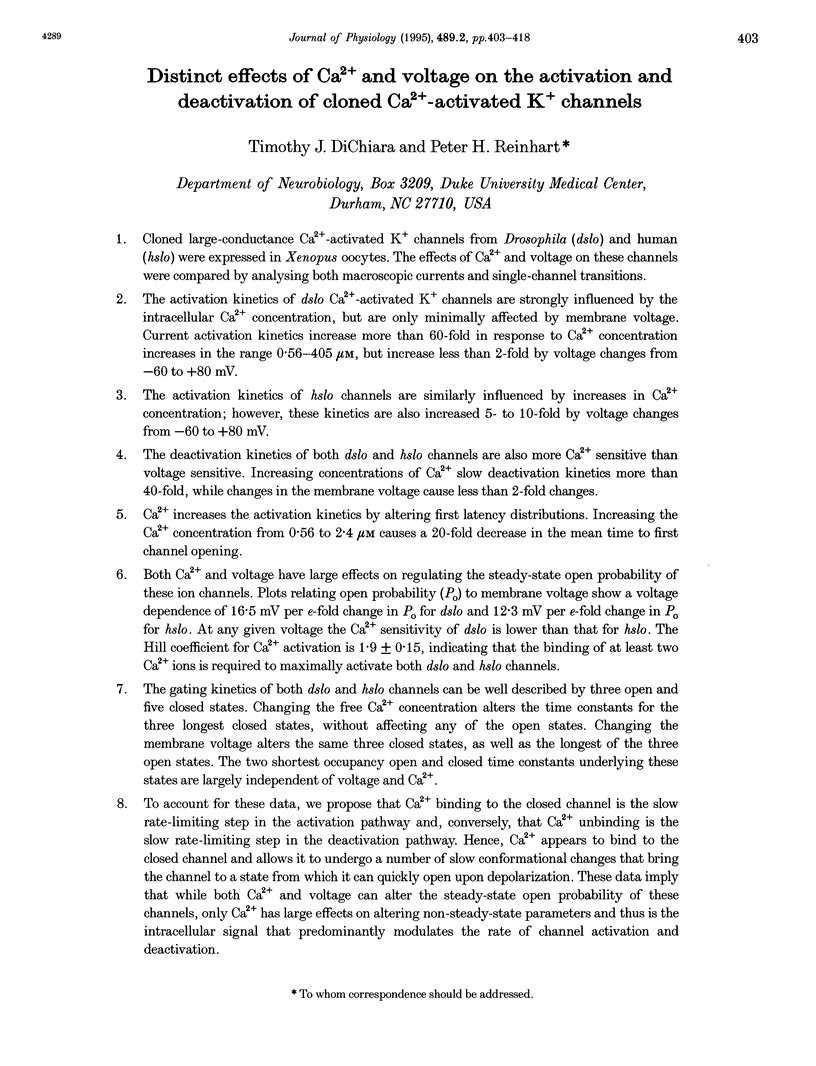
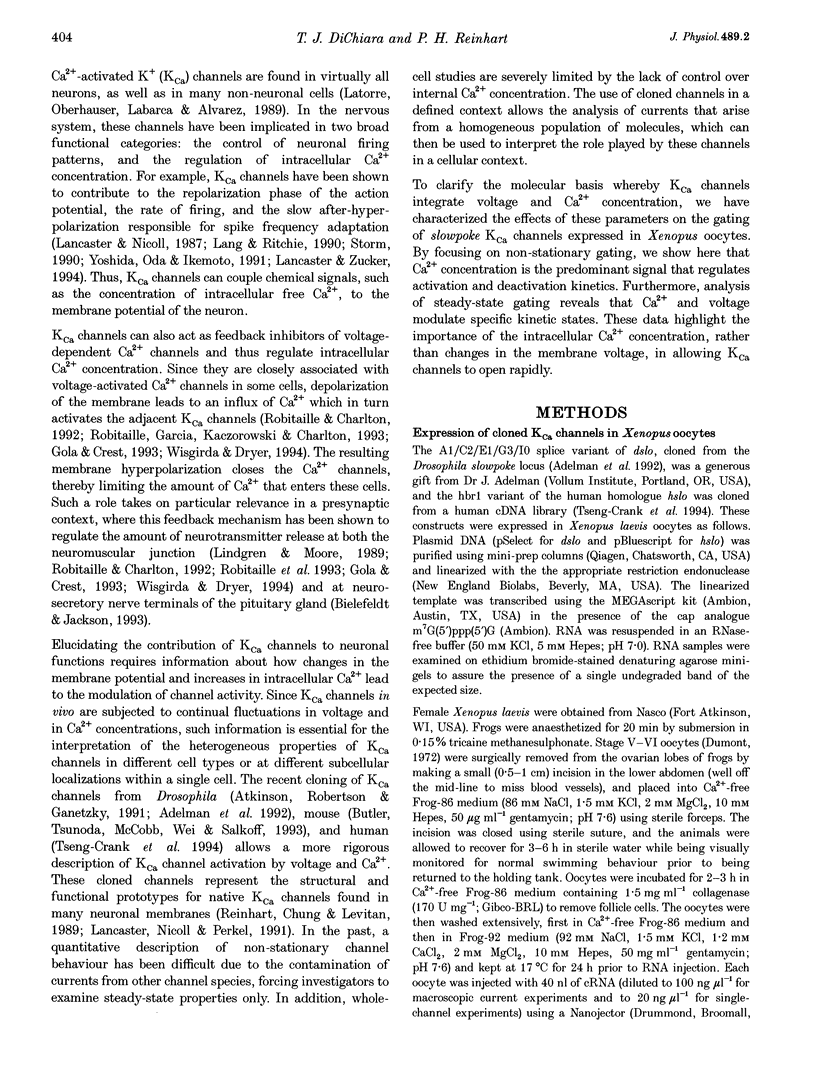
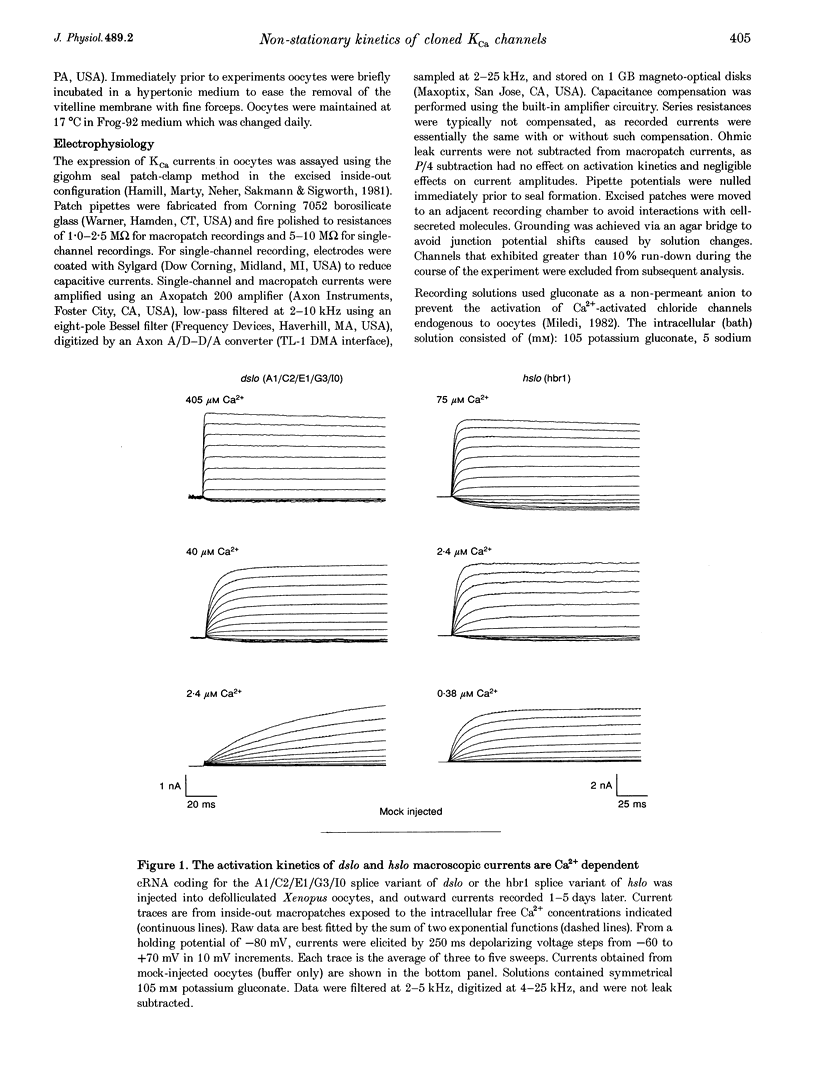
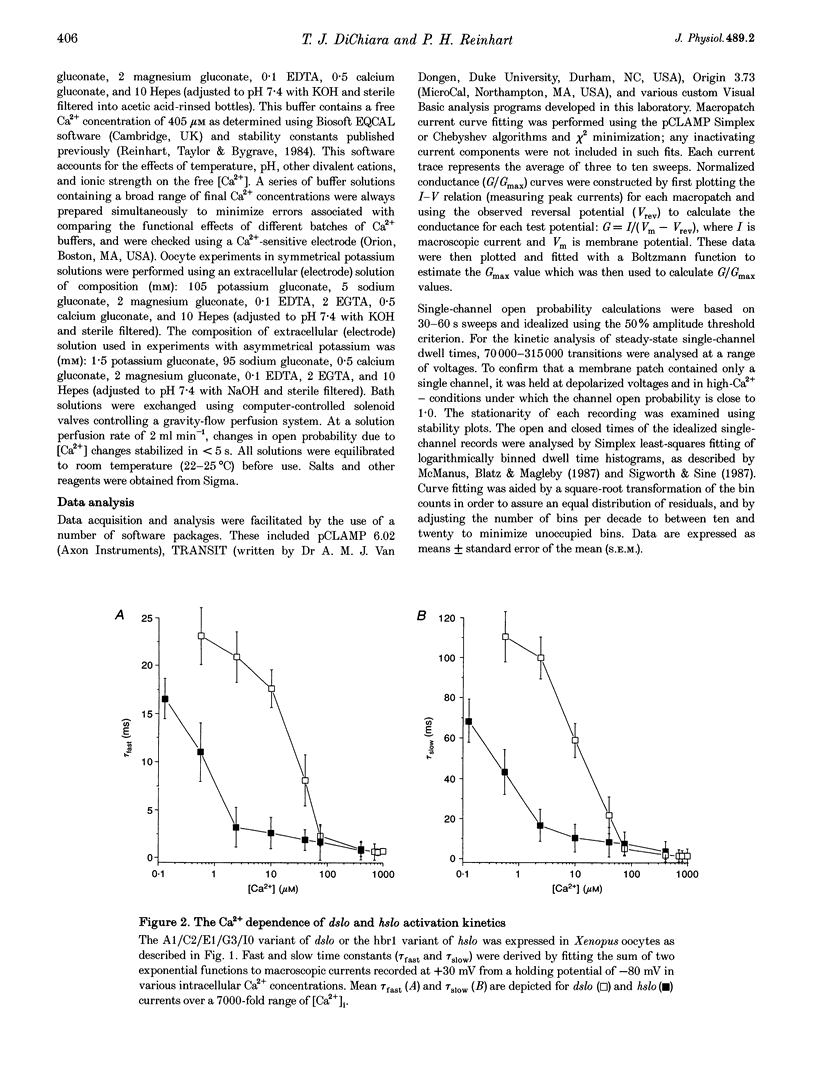
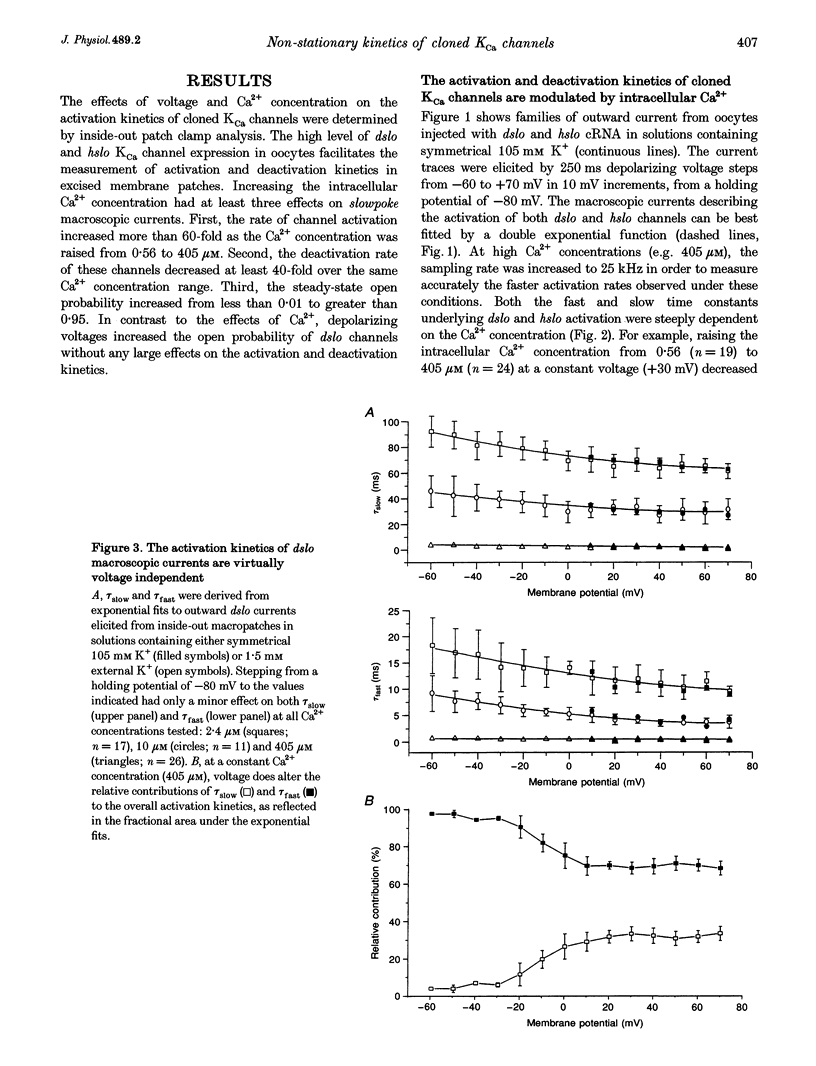
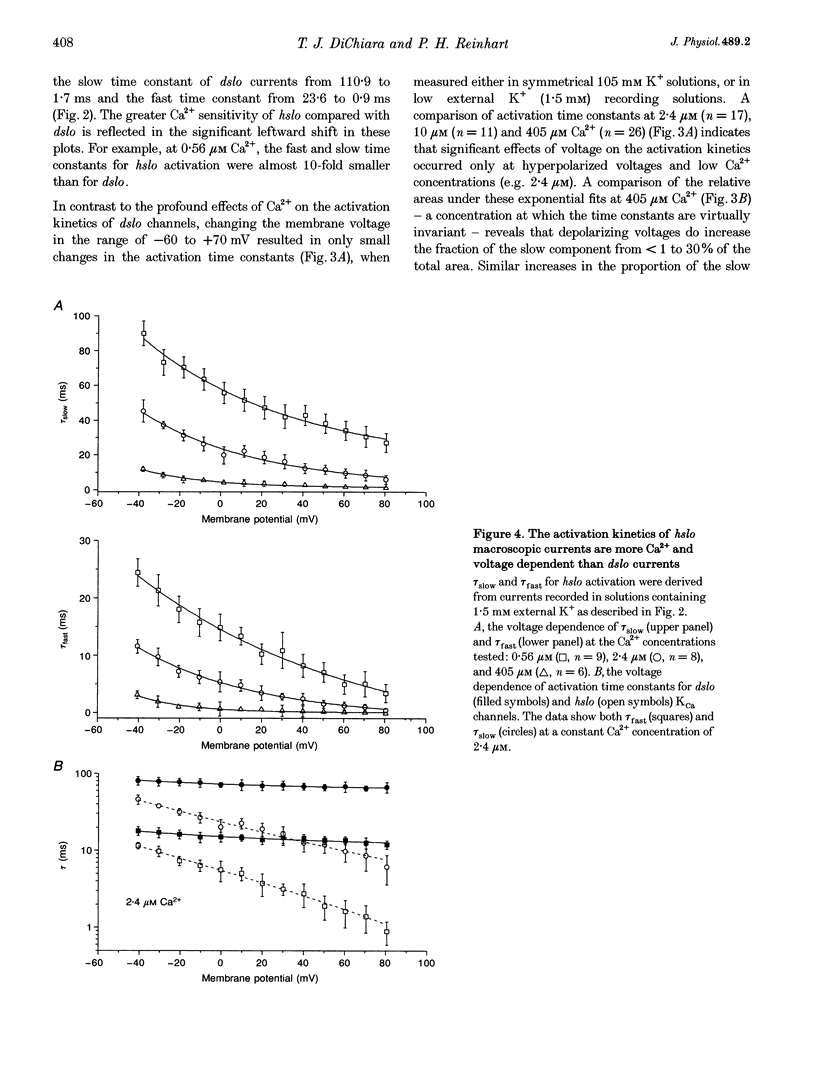
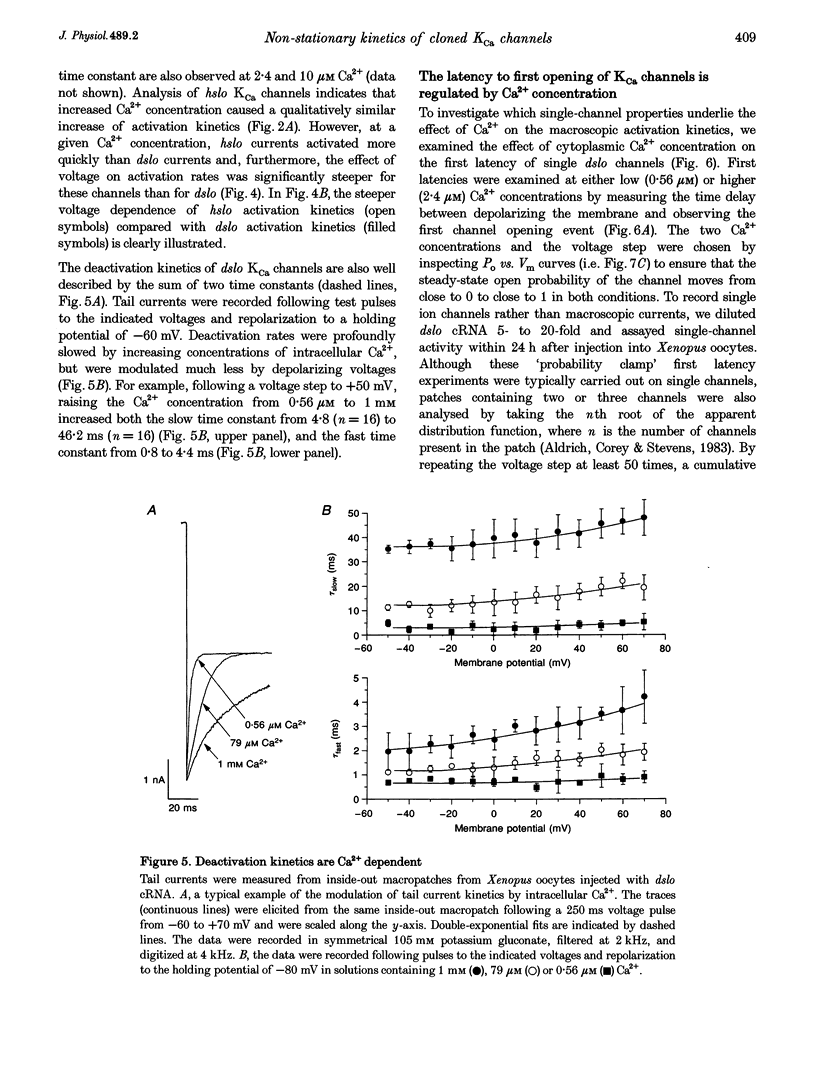
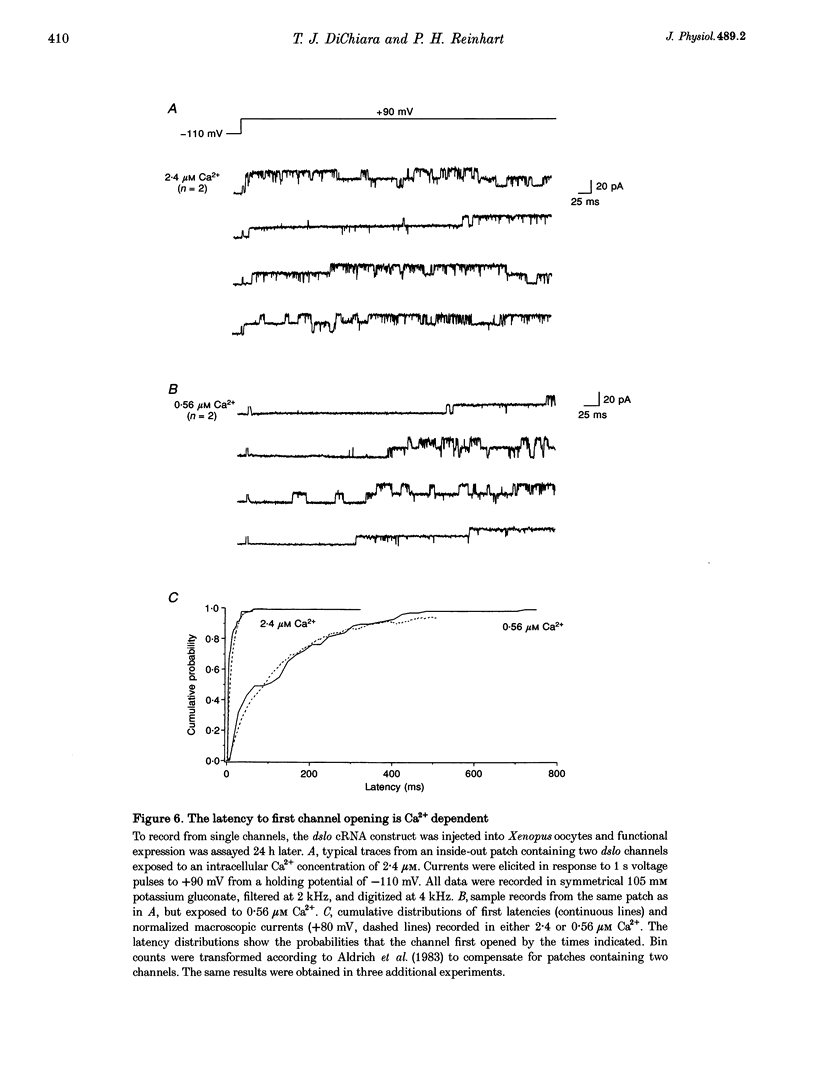
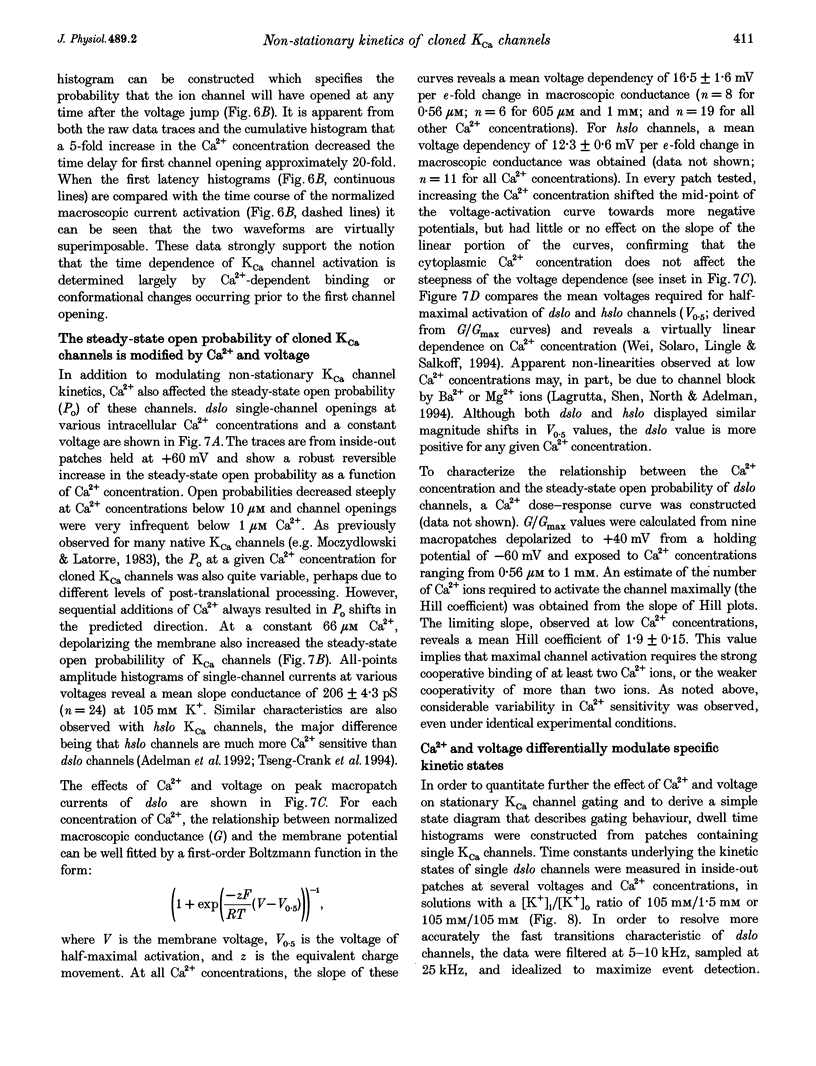
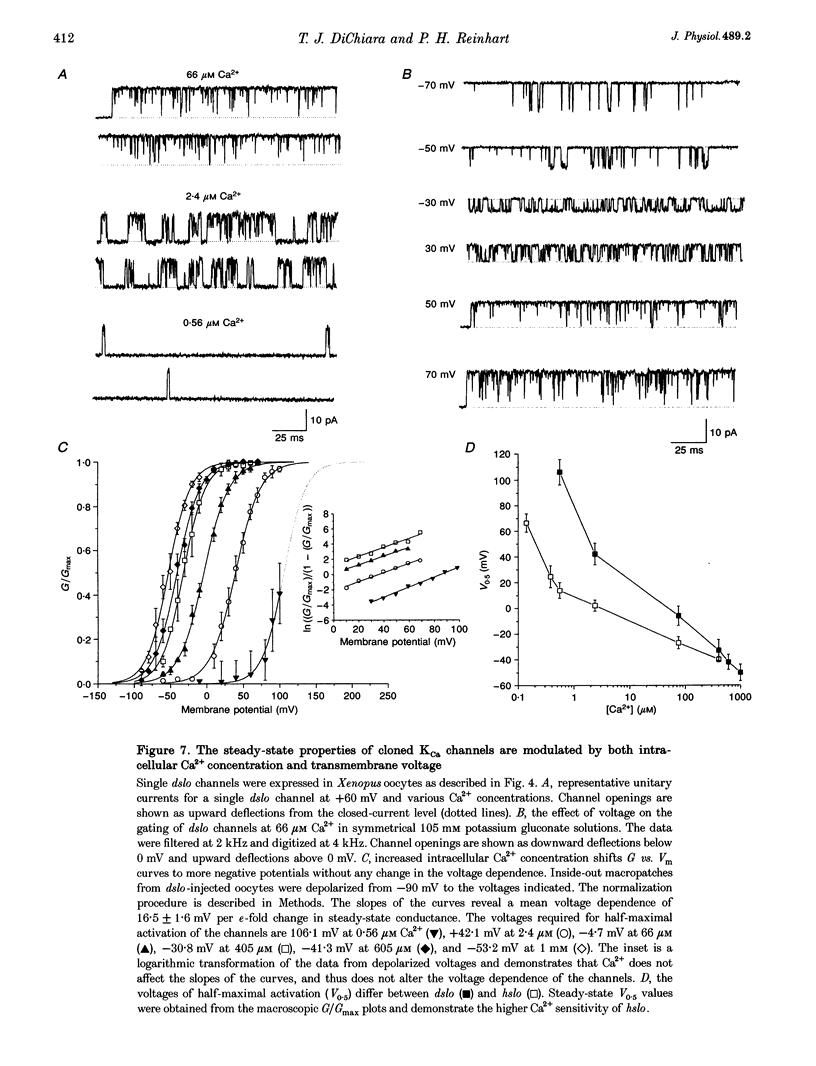
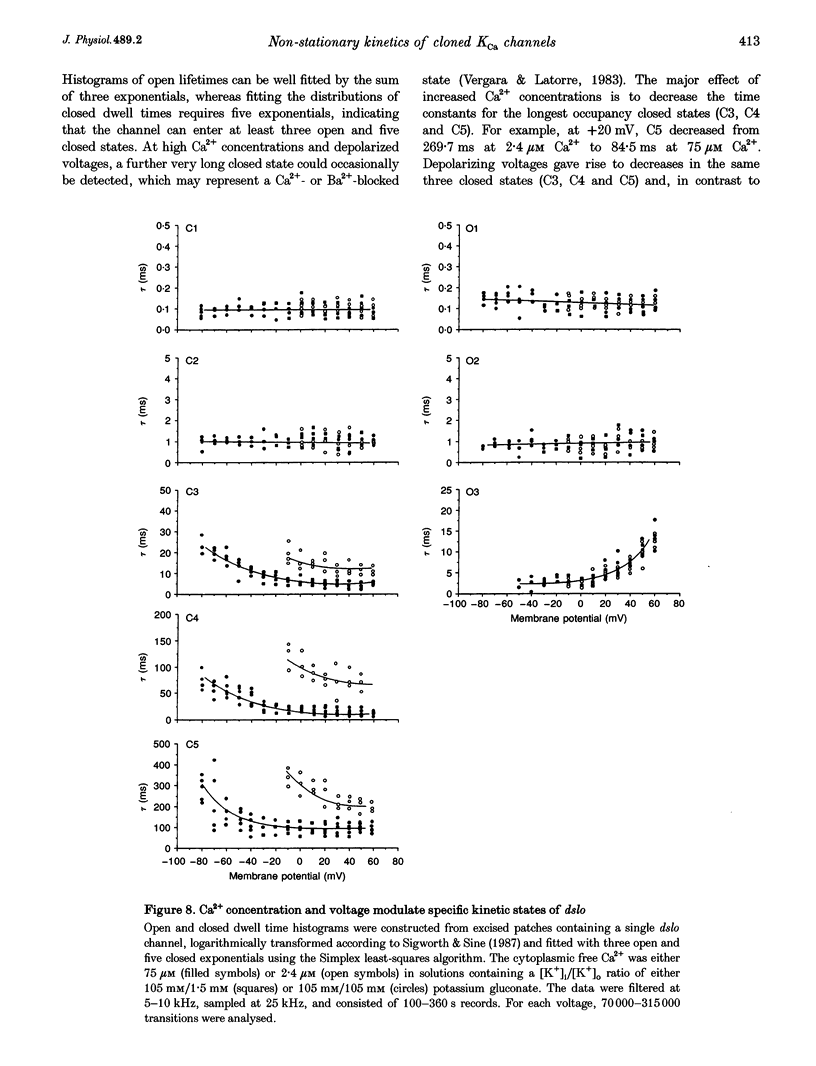
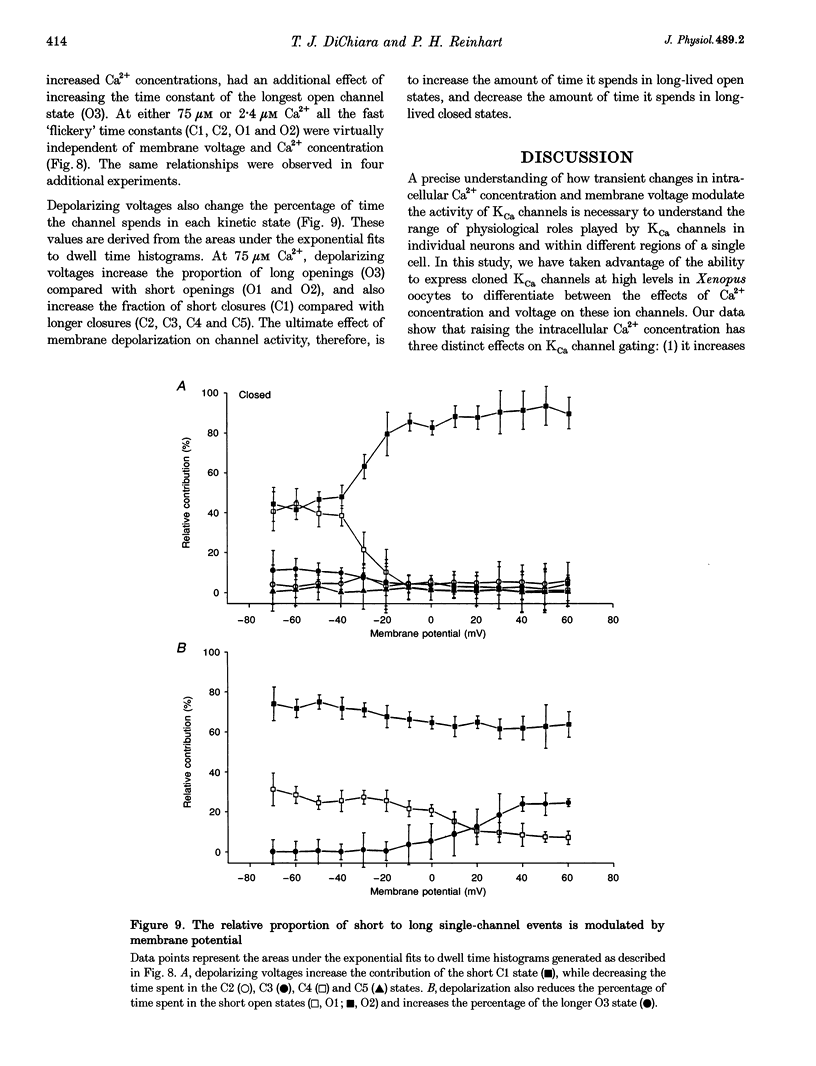
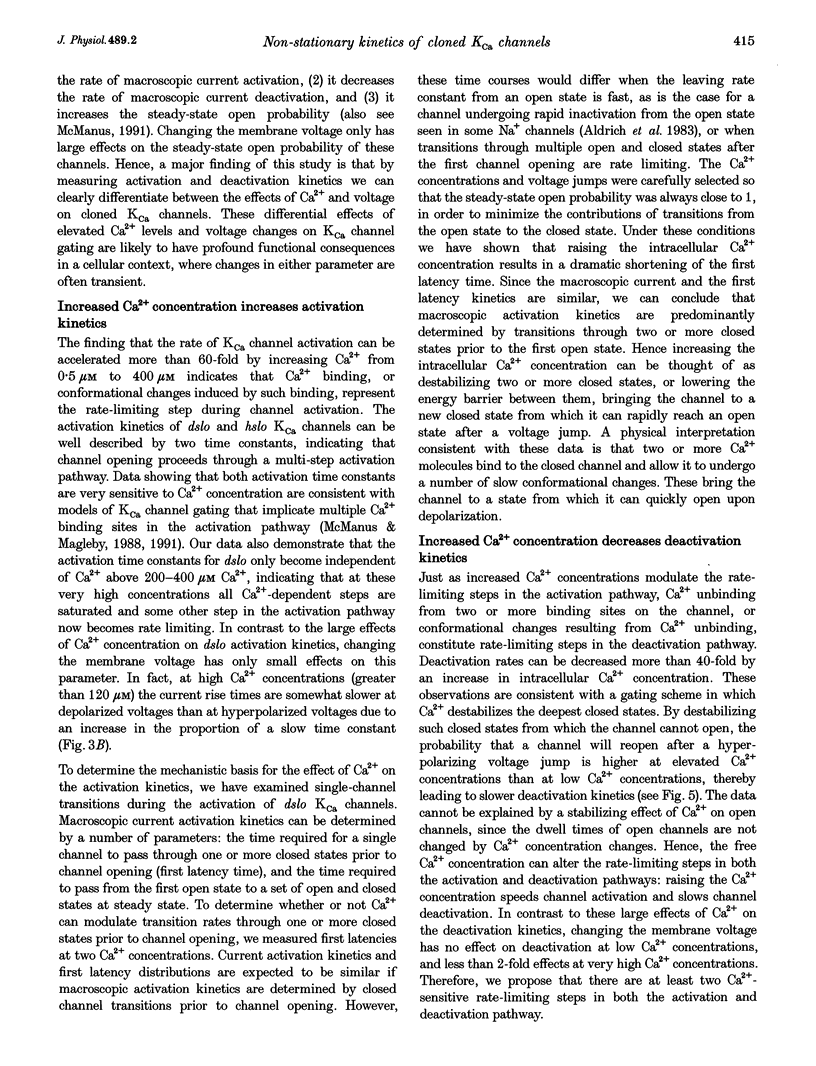
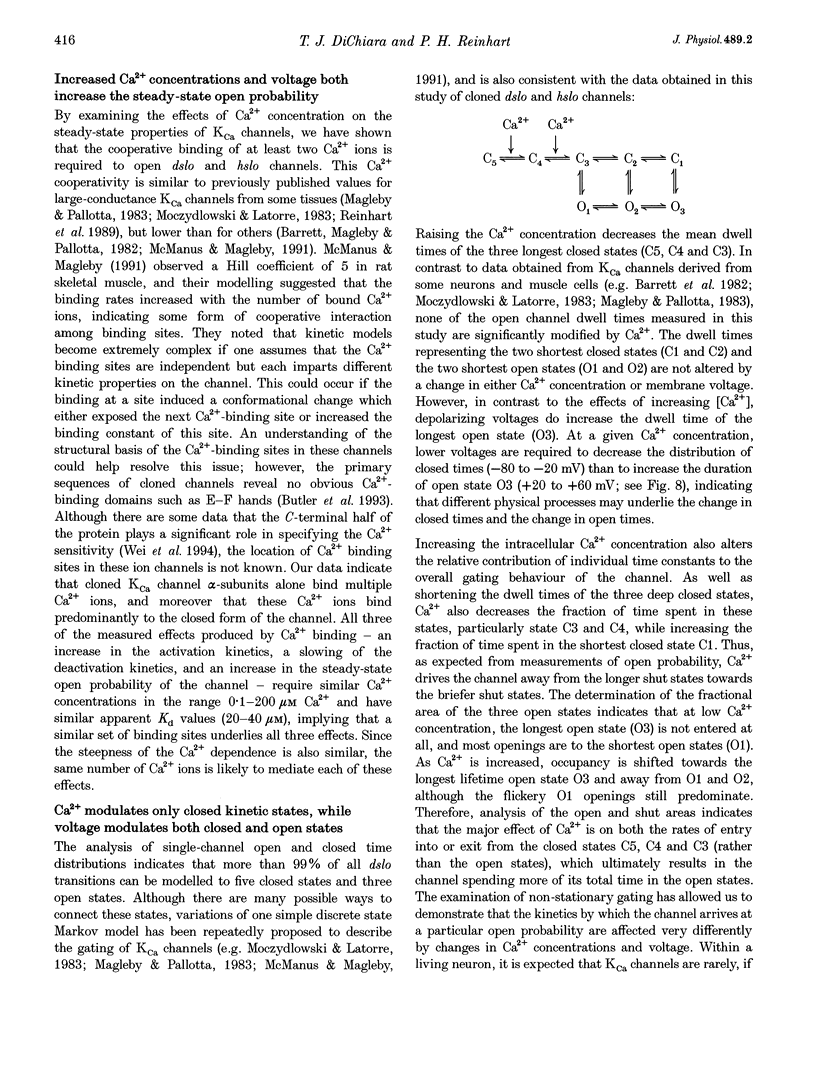
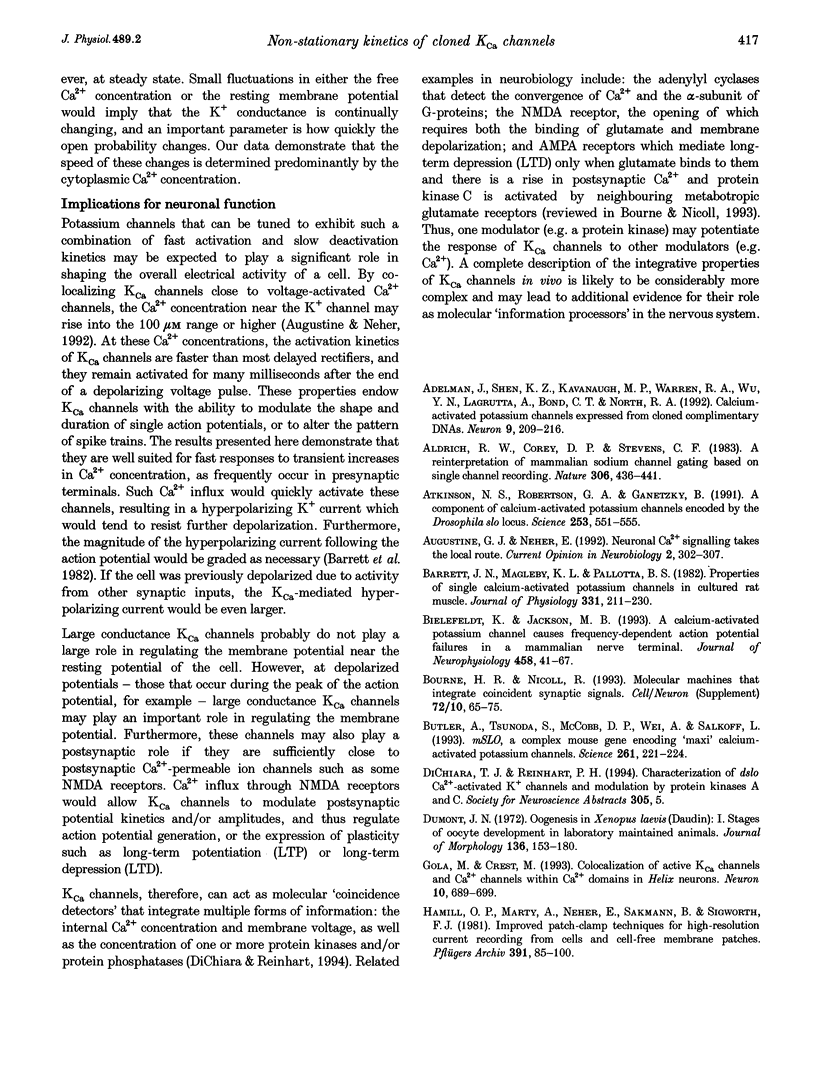
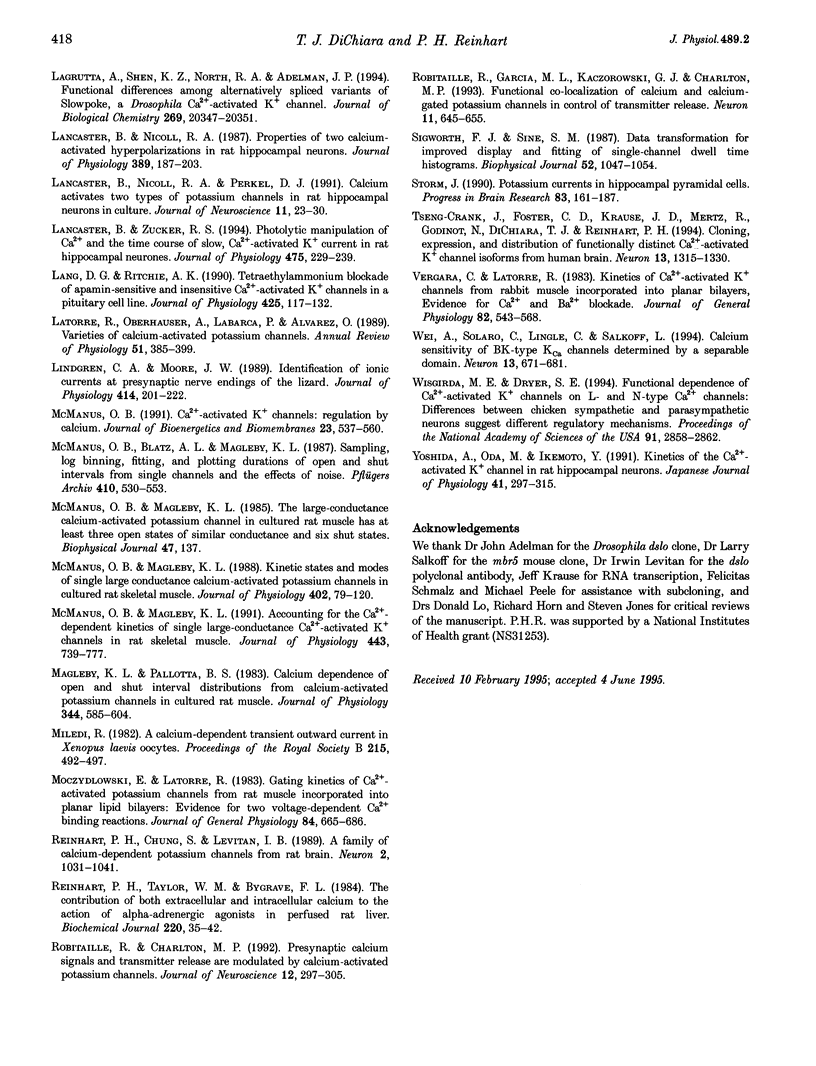
Selected References
These references are in PubMed. This may not be the complete list of references from this article.
- Adelman J. P., Shen K. Z., Kavanaugh M. P., Warren R. A., Wu Y. N., Lagrutta A., Bond C. T., North R. A. Calcium-activated potassium channels expressed from cloned complementary DNAs. Neuron. 1992 Aug;9(2):209–216. doi: 10.1016/0896-6273(92)90160-f. [DOI] [PubMed] [Google Scholar]
- Aldrich R. W., Corey D. P., Stevens C. F. A reinterpretation of mammalian sodium channel gating based on single channel recording. Nature. 1983 Dec 1;306(5942):436–441. doi: 10.1038/306436a0. [DOI] [PubMed] [Google Scholar]
- Atkinson N. S., Robertson G. A., Ganetzky B. A component of calcium-activated potassium channels encoded by the Drosophila slo locus. Science. 1991 Aug 2;253(5019):551–555. doi: 10.1126/science.1857984. [DOI] [PubMed] [Google Scholar]
- Augustine G. J., Neher E. Neuronal Ca2+ signalling takes the local route. Curr Opin Neurobiol. 1992 Jun;2(3):302–307. doi: 10.1016/0959-4388(92)90119-6. [DOI] [PubMed] [Google Scholar]
- Barrett J. N., Magleby K. L., Pallotta B. S. Properties of single calcium-activated potassium channels in cultured rat muscle. J Physiol. 1982 Oct;331:211–230. doi: 10.1113/jphysiol.1982.sp014370. [DOI] [PMC free article] [PubMed] [Google Scholar]
- Bourne H. R., Nicoll R. Molecular machines integrate coincident synaptic signals. Cell. 1993 Jan;72 (Suppl):65–75. doi: 10.1016/s0092-8674(05)80029-7. [DOI] [PubMed] [Google Scholar]
- Butler A., Tsunoda S., McCobb D. P., Wei A., Salkoff L. mSlo, a complex mouse gene encoding "maxi" calcium-activated potassium channels. Science. 1993 Jul 9;261(5118):221–224. doi: 10.1126/science.7687074. [DOI] [PubMed] [Google Scholar]
- Dumont J. N. Oogenesis in Xenopus laevis (Daudin). I. Stages of oocyte development in laboratory maintained animals. J Morphol. 1972 Feb;136(2):153–179. doi: 10.1002/jmor.1051360203. [DOI] [PubMed] [Google Scholar]
- Gola M., Crest M. Colocalization of active KCa channels and Ca2+ channels within Ca2+ domains in helix neurons. Neuron. 1993 Apr;10(4):689–699. doi: 10.1016/0896-6273(93)90170-v. [DOI] [PubMed] [Google Scholar]
- Hamill O. P., Marty A., Neher E., Sakmann B., Sigworth F. J. Improved patch-clamp techniques for high-resolution current recording from cells and cell-free membrane patches. Pflugers Arch. 1981 Aug;391(2):85–100. doi: 10.1007/BF00656997. [DOI] [PubMed] [Google Scholar]
- Lagrutta A., Shen K. Z., North R. A., Adelman J. P. Functional differences among alternatively spliced variants of Slowpoke, a Drosophila calcium-activated potassium channel. J Biol Chem. 1994 Aug 12;269(32):20347–20351. [PubMed] [Google Scholar]
- Lancaster B., Nicoll R. A., Perkel D. J. Calcium activates two types of potassium channels in rat hippocampal neurons in culture. J Neurosci. 1991 Jan;11(1):23–30. doi: 10.1523/JNEUROSCI.11-01-00023.1991. [DOI] [PMC free article] [PubMed] [Google Scholar]
- Lancaster B., Nicoll R. A. Properties of two calcium-activated hyperpolarizations in rat hippocampal neurones. J Physiol. 1987 Aug;389:187–203. doi: 10.1113/jphysiol.1987.sp016653. [DOI] [PMC free article] [PubMed] [Google Scholar]
- Lancaster B., Zucker R. S. Photolytic manipulation of Ca2+ and the time course of slow, Ca(2+)-activated K+ current in rat hippocampal neurones. J Physiol. 1994 Mar 1;475(2):229–239. doi: 10.1113/jphysiol.1994.sp020064. [DOI] [PMC free article] [PubMed] [Google Scholar]
- Lang D. G., Ritchie A. K. Tetraethylammonium blockade of apamin-sensitive and insensitive Ca2(+)-activated K+ channels in a pituitary cell line. J Physiol. 1990 Jun;425:117–132. doi: 10.1113/jphysiol.1990.sp018095. [DOI] [PMC free article] [PubMed] [Google Scholar]
- Latorre R., Oberhauser A., Labarca P., Alvarez O. Varieties of calcium-activated potassium channels. Annu Rev Physiol. 1989;51:385–399. doi: 10.1146/annurev.ph.51.030189.002125. [DOI] [PubMed] [Google Scholar]
- Lindgren C. A., Moore J. W. Identification of ionic currents at presynaptic nerve endings of the lizard. J Physiol. 1989 Jul;414:201–222. doi: 10.1113/jphysiol.1989.sp017684. [DOI] [PMC free article] [PubMed] [Google Scholar]
- Magleby K. L., Pallotta B. S. Calcium dependence of open and shut interval distributions from calcium-activated potassium channels in cultured rat muscle. J Physiol. 1983 Nov;344:585–604. doi: 10.1113/jphysiol.1983.sp014957. [DOI] [PMC free article] [PubMed] [Google Scholar]
- McManus O. B., Blatz A. L., Magleby K. L. Sampling, log binning, fitting, and plotting durations of open and shut intervals from single channels and the effects of noise. Pflugers Arch. 1987 Nov;410(4-5):530–553. doi: 10.1007/BF00586537. [DOI] [PubMed] [Google Scholar]
- McManus O. B. Calcium-activated potassium channels: regulation by calcium. J Bioenerg Biomembr. 1991 Aug;23(4):537–560. doi: 10.1007/BF00785810. [DOI] [PubMed] [Google Scholar]
- McManus O. B., Magleby K. L. Accounting for the Ca(2+)-dependent kinetics of single large-conductance Ca(2+)-activated K+ channels in rat skeletal muscle. J Physiol. 1991 Nov;443:739–777. doi: 10.1113/jphysiol.1991.sp018861. [DOI] [PMC free article] [PubMed] [Google Scholar]
- Moczydlowski E., Garber S. S., Miller C. Batrachotoxin-activated Na+ channels in planar lipid bilayers. Competition of tetrodotoxin block by Na+. J Gen Physiol. 1984 Nov;84(5):665–686. doi: 10.1085/jgp.84.5.665. [DOI] [PMC free article] [PubMed] [Google Scholar]
- Reinhart P. H., Chung S., Levitan I. B. A family of calcium-dependent potassium channels from rat brain. Neuron. 1989 Jan;2(1):1031–1041. doi: 10.1016/0896-6273(89)90227-4. [DOI] [PubMed] [Google Scholar]
- Reinhart P. H., Taylor W. M., Bygrave F. L. The contribution of both extracellular and intracellular calcium to the action of alpha-adrenergic agonists in perfused rat liver. Biochem J. 1984 May 15;220(1):35–42. doi: 10.1042/bj2200035. [DOI] [PMC free article] [PubMed] [Google Scholar]
- Robitaille R., Charlton M. P. Presynaptic calcium signals and transmitter release are modulated by calcium-activated potassium channels. J Neurosci. 1992 Jan;12(1):297–305. doi: 10.1523/JNEUROSCI.12-01-00297.1992. [DOI] [PMC free article] [PubMed] [Google Scholar]
- Robitaille R., Garcia M. L., Kaczorowski G. J., Charlton M. P. Functional colocalization of calcium and calcium-gated potassium channels in control of transmitter release. Neuron. 1993 Oct;11(4):645–655. doi: 10.1016/0896-6273(93)90076-4. [DOI] [PubMed] [Google Scholar]
- Sigworth F. J., Sine S. M. Data transformations for improved display and fitting of single-channel dwell time histograms. Biophys J. 1987 Dec;52(6):1047–1054. doi: 10.1016/S0006-3495(87)83298-8. [DOI] [PMC free article] [PubMed] [Google Scholar]
- Storm J. F. Potassium currents in hippocampal pyramidal cells. Prog Brain Res. 1990;83:161–187. doi: 10.1016/s0079-6123(08)61248-0. [DOI] [PubMed] [Google Scholar]
- Tseng-Crank J., Foster C. D., Krause J. D., Mertz R., Godinot N., DiChiara T. J., Reinhart P. H. Cloning, expression, and distribution of functionally distinct Ca(2+)-activated K+ channel isoforms from human brain. Neuron. 1994 Dec;13(6):1315–1330. doi: 10.1016/0896-6273(94)90418-9. [DOI] [PubMed] [Google Scholar]
- Vergara C., Latorre R. Kinetics of Ca2+-activated K+ channels from rabbit muscle incorporated into planar bilayers. Evidence for a Ca2+ and Ba2+ blockade. J Gen Physiol. 1983 Oct;82(4):543–568. doi: 10.1085/jgp.82.4.543. [DOI] [PMC free article] [PubMed] [Google Scholar]
- Wei A., Solaro C., Lingle C., Salkoff L. Calcium sensitivity of BK-type KCa channels determined by a separable domain. Neuron. 1994 Sep;13(3):671–681. doi: 10.1016/0896-6273(94)90034-5. [DOI] [PubMed] [Google Scholar]
- Wisgirda M. E., Dryer S. E. Functional dependence of Ca(2+)-activated K+ current on L- and N-type Ca2+ channels: differences between chicken sympathetic and parasympathetic neurons suggest different regulatory mechanisms. Proc Natl Acad Sci U S A. 1994 Mar 29;91(7):2858–2862. doi: 10.1073/pnas.91.7.2858. [DOI] [PMC free article] [PubMed] [Google Scholar]
- Yoshida A., Oda M., Ikemoto Y. Kinetics of the Ca(2+)-activated K+ channel in rat hippocampal neurons. Jpn J Physiol. 1991;41(2):297–315. doi: 10.2170/jjphysiol.41.297. [DOI] [PubMed] [Google Scholar]