Abstract
1. The effect of varying the concentration of intracellular magnesium on the Ca(2+)-saturated Ca(2+)-extrusion rate through the Ca2+ pump (phi max) was investigated in human red blood cells with the aid of the divalent cation ionophore A23187. The aim was to characterize the [Mg2+]i dependence of the Ca2+ pump in the intact cell. 2. The initial experimental protocol consisted of applying a high ionophore concentration to obtain rapid sequential Mg2+ and [45Ca]CaCl2 equilibration, prior to measuring phi max at constant internal [MgT]i by either the Co2+ block method or by ionophore removal. With this protocol, competition between Ca2+ and Mg2+ through the ionophore prevented Ca2+ equilibration at high [Mg2+]o. To provide rapid and comparable Ca2+ loads and maintain intracellular ATP within normal levels it was necessary to separate the Mg2+ and the Ca2+ loading-extrusion stages by an intermediate ionophore and external Mg2+ removal step, and to use different metabolic substrates during Mg2+ loading (glucose) and Ca2+ loading-extrusion (inosine) periods. 3. Intracellular Co2+ was found to sustain Ca2+ extrusion by the pump at subphysiological [Mg2+]i. Ionophore removal was therefore used to estimate the [Mg2+]i dependence of the pump at levels below [MgT]i (approximately 2 mmol (340 g Hb)-1), whereas both ionophore removal and Co2+ block were used for higher [MgT]i levels. 4. [Mg2+]i was computed from measured [MgT]i using known cytoplasmic Mg(2+)-buffering data. The phi max of the Ca2+ pump increased hyperbolically with [Mg2+]i. The Michaelis parameter (K 1/2) of activation was 0.12 +/- 0.04 mmol (1 cell water)-1 (mean +/- S.E.M.). Increasing [MgT]i and [Mg2+]i to 9 mmol (340 g Hb)-1 and 2.6 mmol (1 cell water)-1, respectively, failed to cause significant inhibition of the phi max of the Ca2+ pump. 5. The results suggest that within the physiological and pathophysiological range of [Mg2+]i, from 0.3 mmol (1 cell water)-1 in the oxygenated state to 1.2 mmol (1 cell water)-1 in the deoxygenated state, the Ca(2+)-saturated Ca2+ pump remains unaffected by [Mg2+]i at normal ATP levels.
Full text
PDF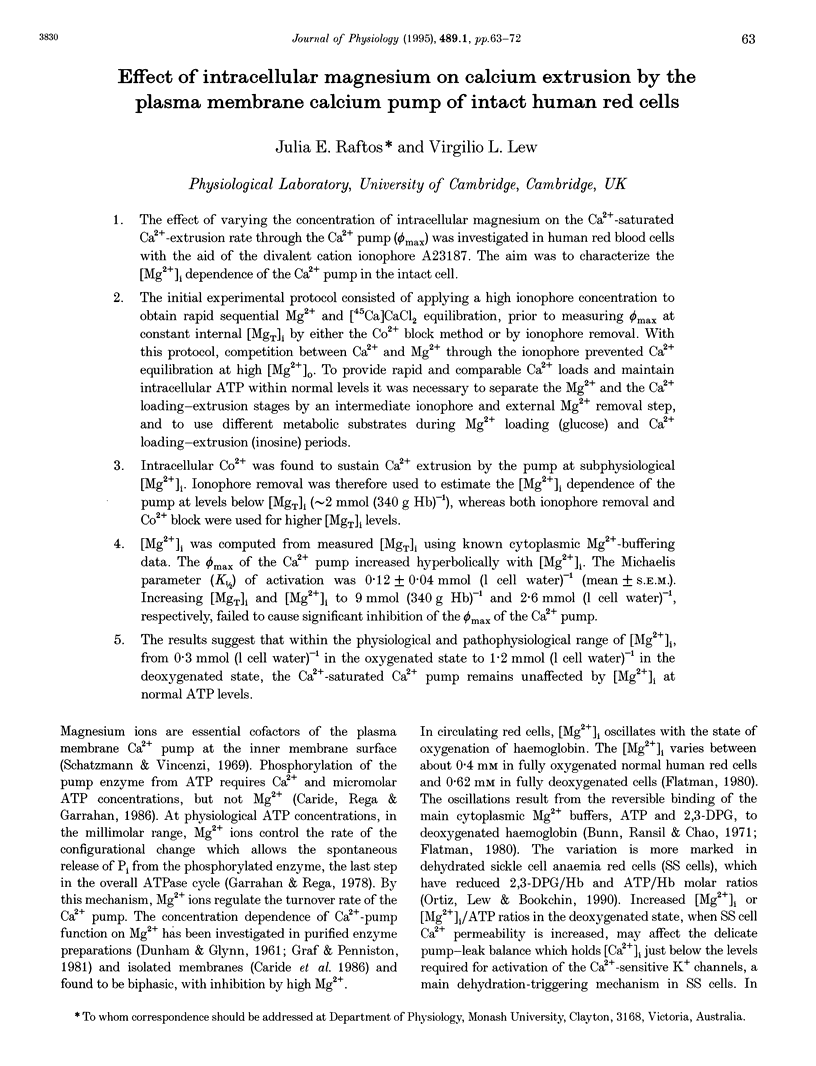
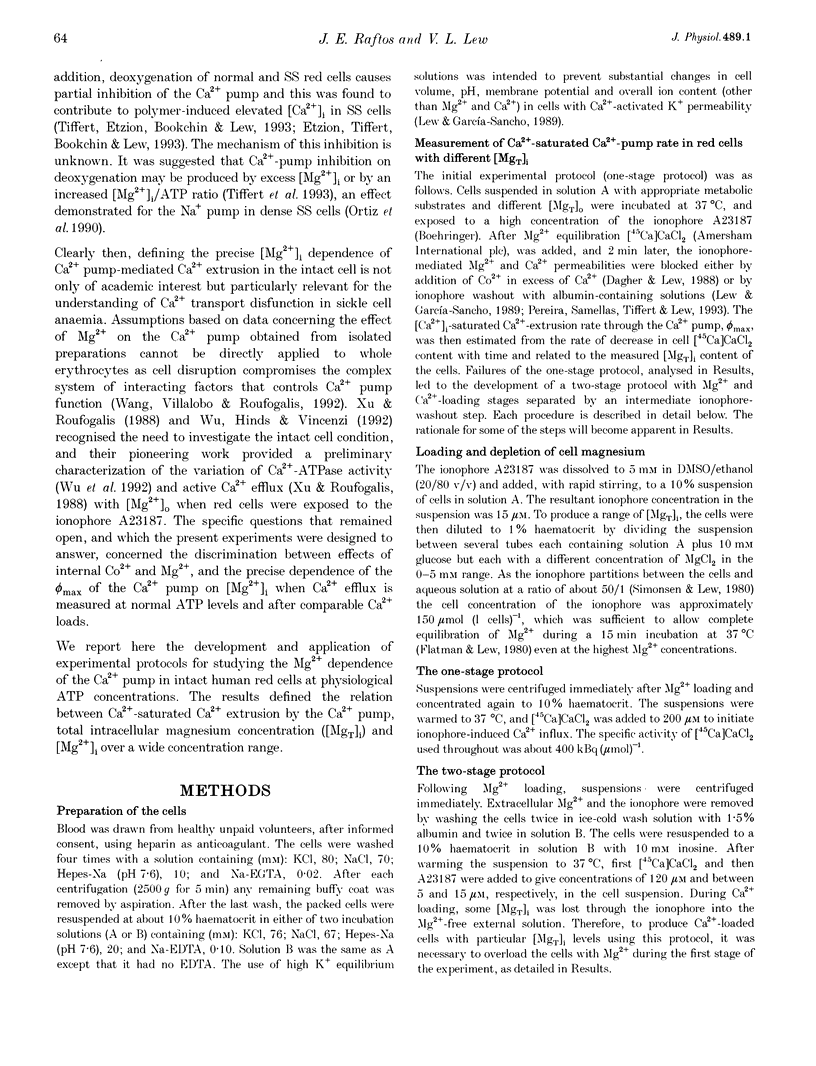
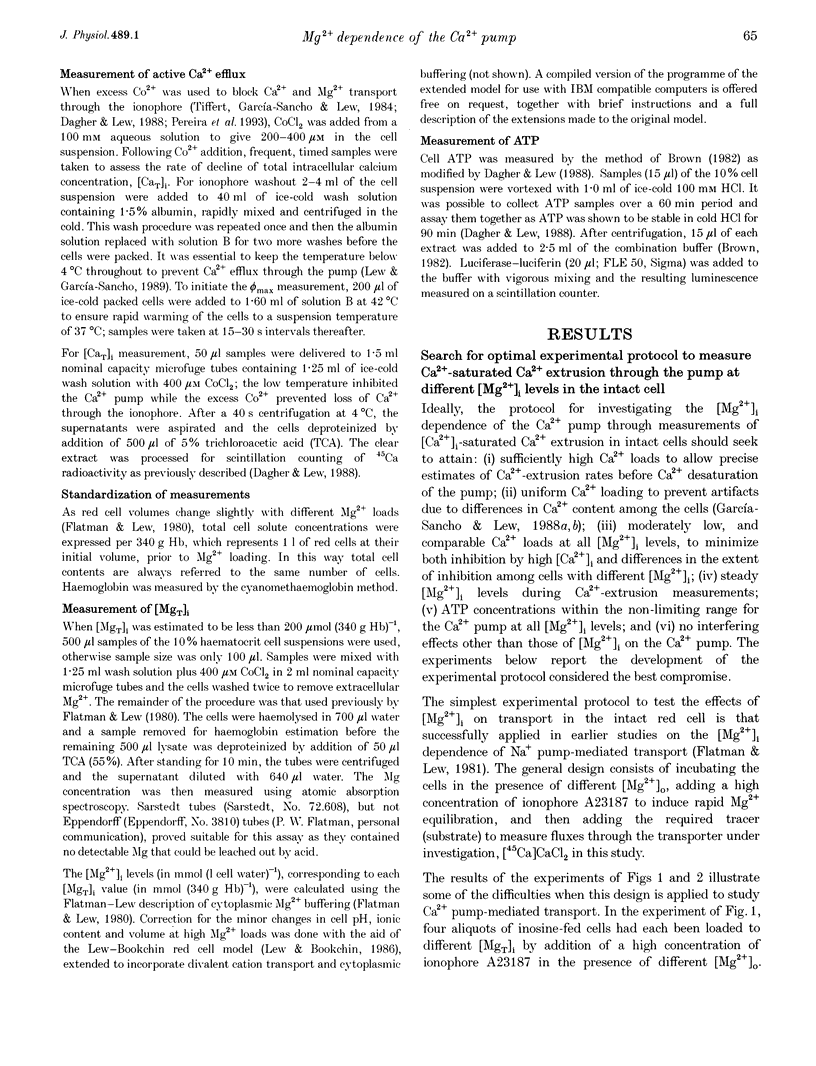
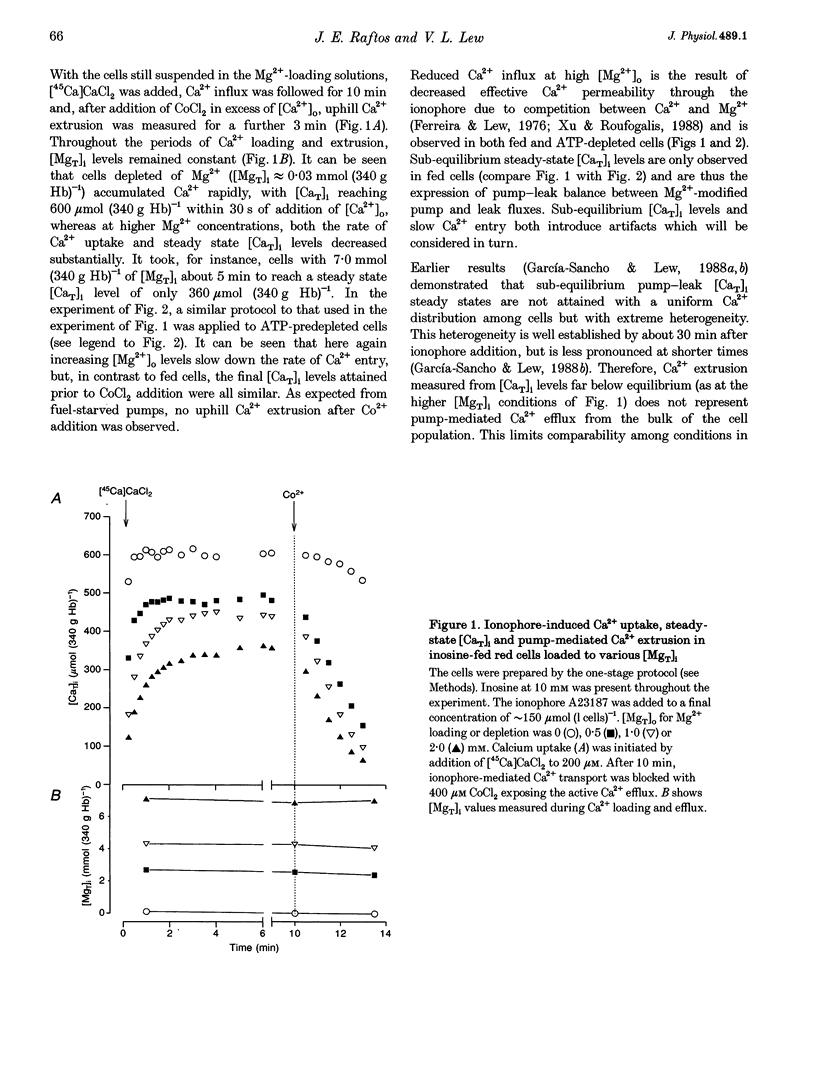
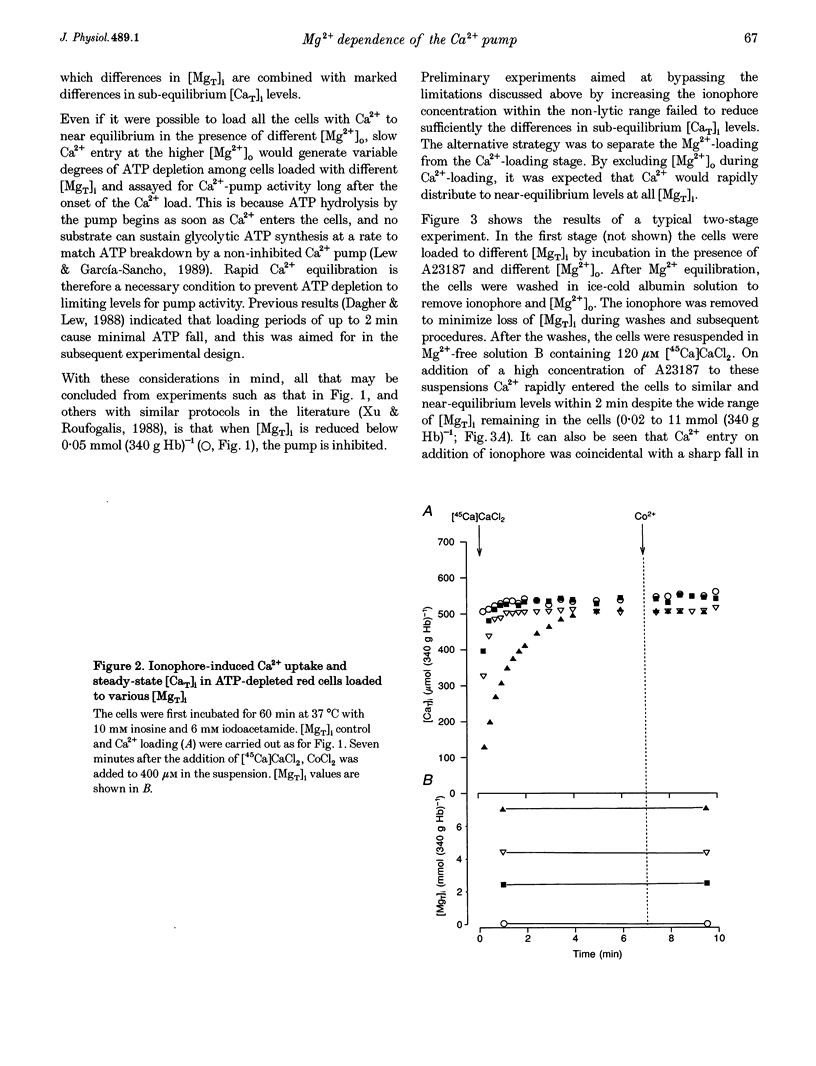
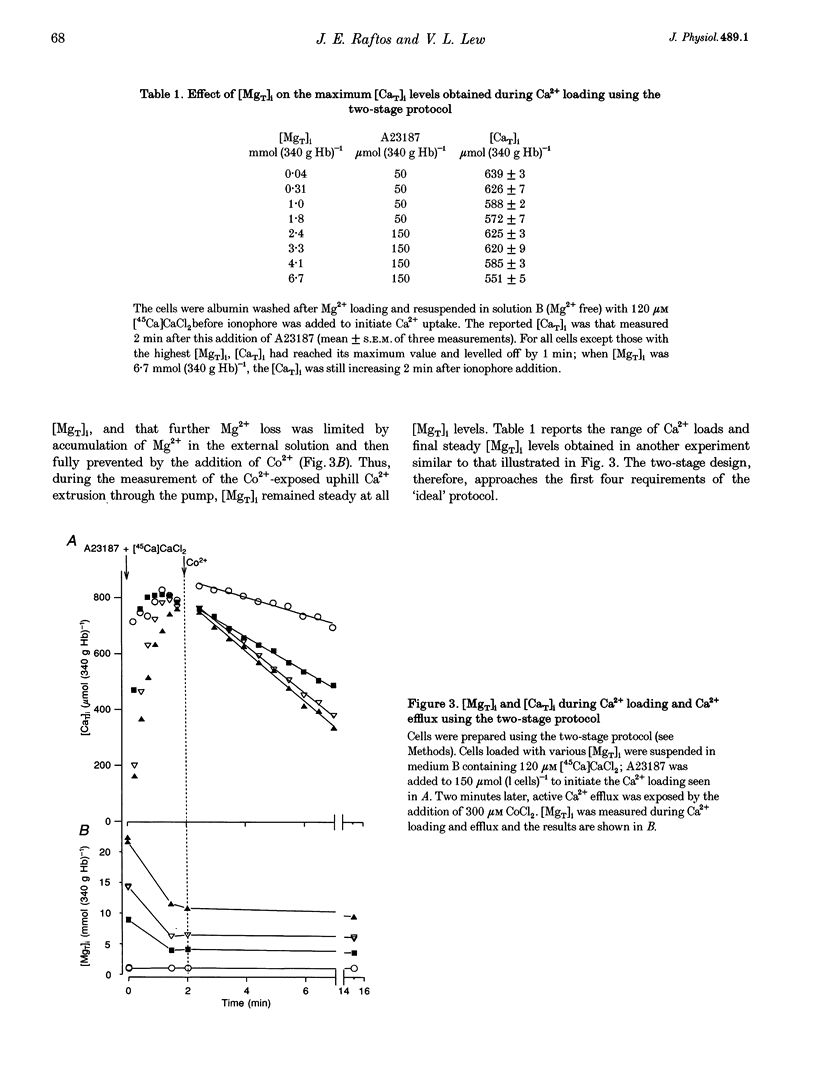
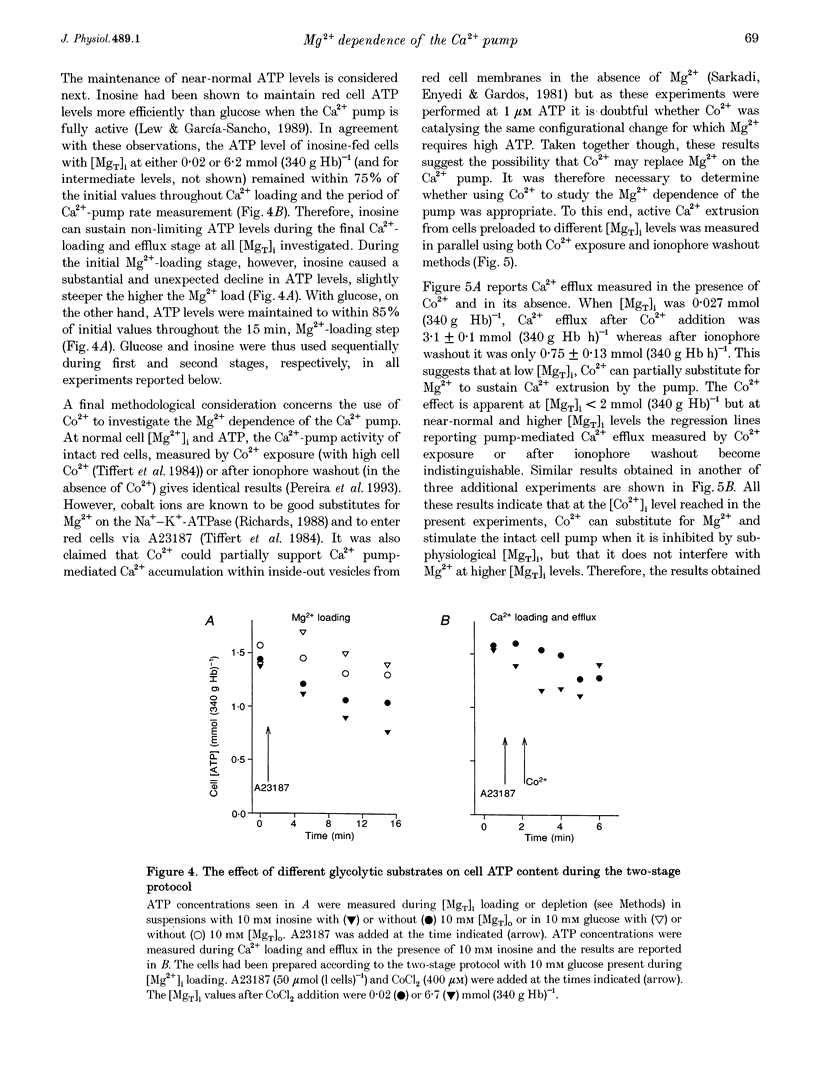
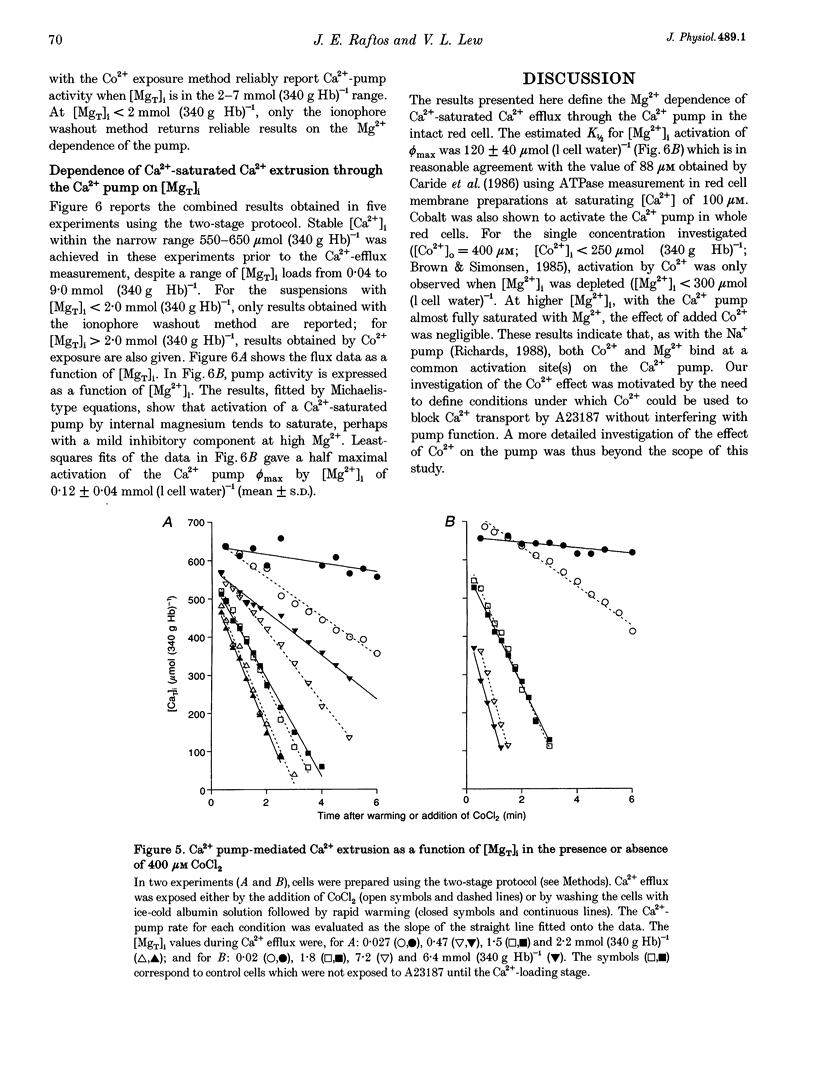
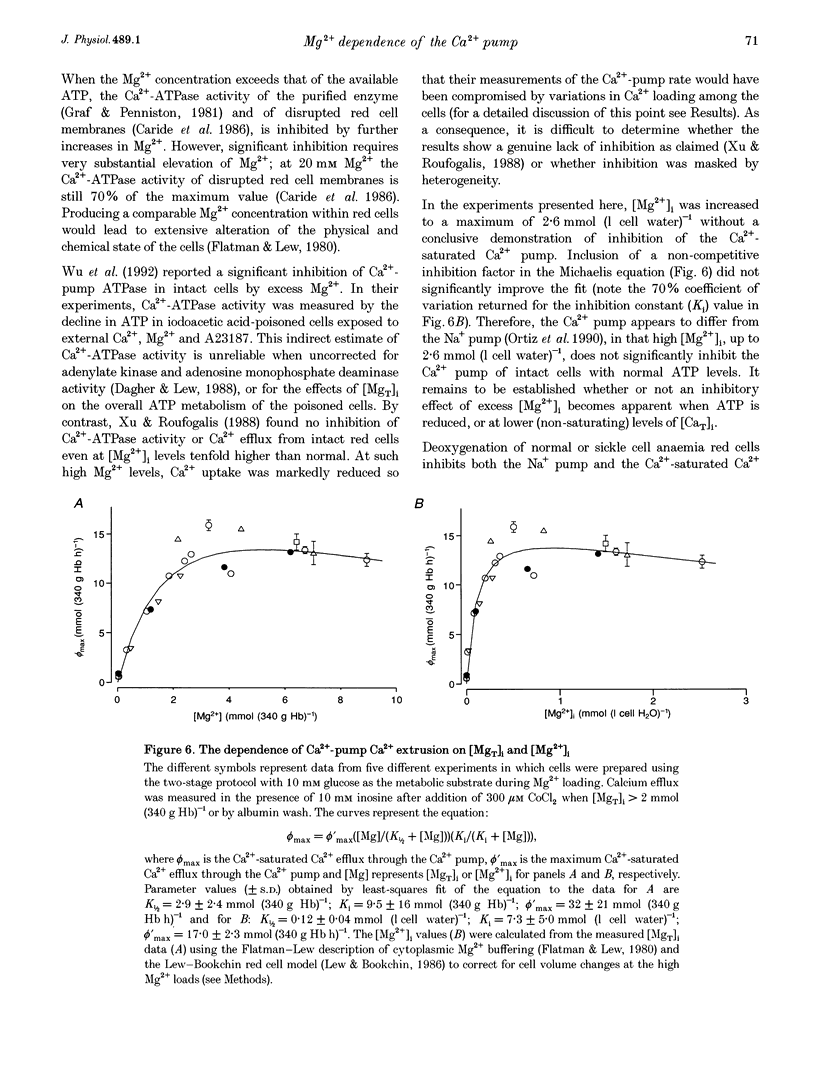
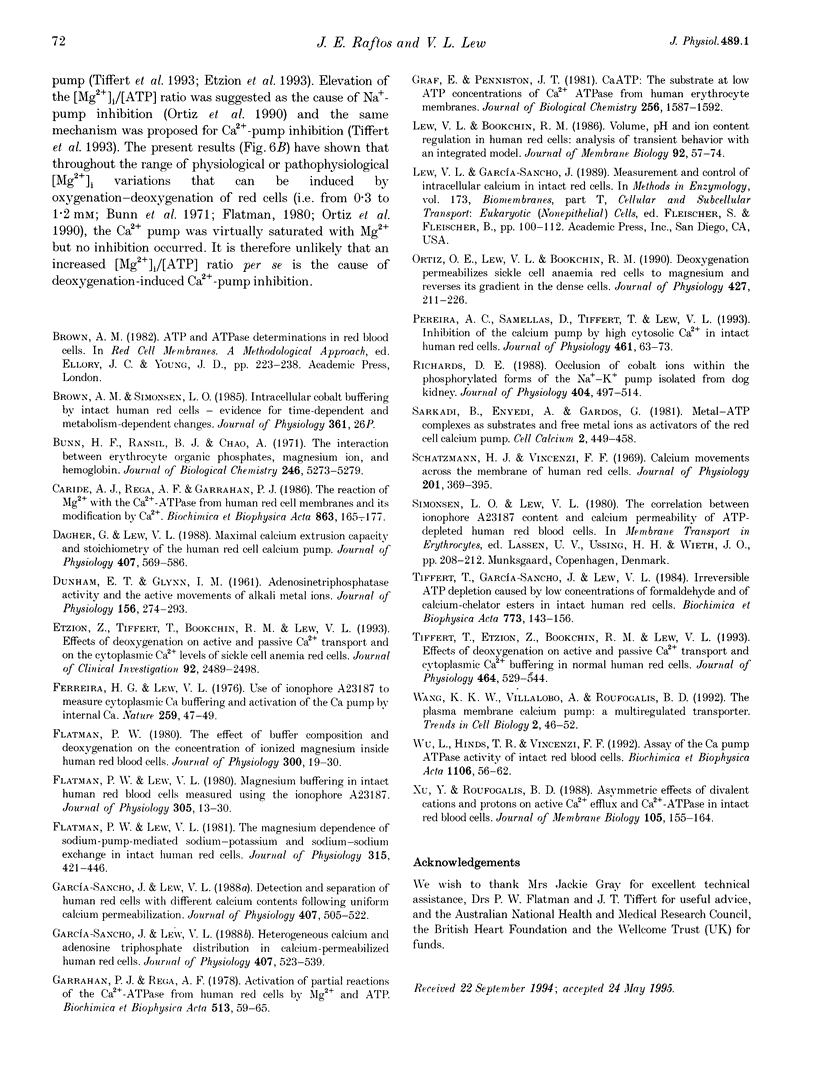
Selected References
These references are in PubMed. This may not be the complete list of references from this article.
- Bunn H. F., Ransil B. J., Chao A. The interaction between erythrocyte organic phosphates, magnesium ion, and hemoglobin. J Biol Chem. 1971 Sep 10;246(17):5273–5279. [PubMed] [Google Scholar]
- Caride A. J., Rega A. F., Garrahan P. J. The reaction of Mg2+ with the Ca2+-ATPase from human red cell membranes and its modification by Ca2+. Biochim Biophys Acta. 1986 Dec 16;863(2):165–177. doi: 10.1016/0005-2736(86)90256-7. [DOI] [PubMed] [Google Scholar]
- DUNHAM E. T., GLYNN I. M. Adenosinetriphosphatase activity and the active movements of alkali metal ions. J Physiol. 1961 Apr;156:274–293. doi: 10.1113/jphysiol.1961.sp006675. [DOI] [PMC free article] [PubMed] [Google Scholar]
- Dagher G., Lew V. L. Maximal calcium extrusion capacity and stoichiometry of the human red cell calcium pump. J Physiol. 1988 Dec;407:569–586. doi: 10.1113/jphysiol.1988.sp017432. [DOI] [PMC free article] [PubMed] [Google Scholar]
- Etzion Z., Tiffert T., Bookchin R. M., Lew V. L. Effects of deoxygenation on active and passive Ca2+ transport and on the cytoplasmic Ca2+ levels of sickle cell anemia red cells. J Clin Invest. 1993 Nov;92(5):2489–2498. doi: 10.1172/JCI116857. [DOI] [PMC free article] [PubMed] [Google Scholar]
- Ferreira H. G., Lew V. L. Use of ionophore A23187 to measure cytoplasmic Ca buffering and activation of the Ca pump by internal Ca. Nature. 1976 Jan 1;259(5538):47–49. doi: 10.1038/259047a0. [DOI] [PubMed] [Google Scholar]
- Flatman P. W., Lew V. L. Magnesium buffering in intact human red blood cells measured using the ionophore A23187. J Physiol. 1980 Aug;305:13–30. doi: 10.1113/jphysiol.1980.sp013346. [DOI] [PMC free article] [PubMed] [Google Scholar]
- Flatman P. W., Lew V. L. The magnesium dependence of sodium-pump-mediated sodium-potassium and sodium-sodium exchange in intact human red cells. J Physiol. 1981 Jun;315:421–446. doi: 10.1113/jphysiol.1981.sp013756. [DOI] [PMC free article] [PubMed] [Google Scholar]
- Flatman P. W. The effect of buffer composition and deoxygenation on the concentration of ionized magnesium inside human red blood cells. J Physiol. 1980 Mar;300:19–30. doi: 10.1113/jphysiol.1980.sp013148. [DOI] [PMC free article] [PubMed] [Google Scholar]
- García-Sancho J., Lew V. L. Detection and separation of human red cells with different calcium contents following uniform calcium permeabilization. J Physiol. 1988 Dec;407:505–522. doi: 10.1113/jphysiol.1988.sp017428. [DOI] [PMC free article] [PubMed] [Google Scholar]
- García-Sancho J., Lew V. L. Heterogeneous calcium and adenosine triphosphate distribution in calcium-permeabilized human red cells. J Physiol. 1988 Dec;407:523–539. doi: 10.1113/jphysiol.1988.sp017429. [DOI] [PMC free article] [PubMed] [Google Scholar]
- Garrahan P. J., Rega A. F. Activation of partial reactions of the Ca2+-ATPase from human red cells by Mg2+ and ATP. Biochim Biophys Acta. 1978 Oct 19;513(1):59–65. doi: 10.1016/0005-2736(78)90111-6. [DOI] [PubMed] [Google Scholar]
- Graf E., Penniston J. T. CaATP: the substrate, at low ATP concentrations, of Ca2+ ATPase from human erythrocyte membranes. J Biol Chem. 1981 Feb 25;256(4):1587–1592. [PubMed] [Google Scholar]
- Lew V. L., Bookchin R. M. Volume, pH, and ion-content regulation in human red cells: analysis of transient behavior with an integrated model. J Membr Biol. 1986;92(1):57–74. doi: 10.1007/BF01869016. [DOI] [PubMed] [Google Scholar]
- Lew V. L., García-Sancho J. Measurement and control of intracellular calcium in intact red cells. Methods Enzymol. 1989;173:100–112. doi: 10.1016/s0076-6879(89)73008-1. [DOI] [PubMed] [Google Scholar]
- Ortiz O. E., Lew V. L., Bookchin R. M. Deoxygenation permeabilizes sickle cell anaemia red cells to magnesium and reverses its gradient in the dense cells. J Physiol. 1990 Aug;427:211–226. doi: 10.1113/jphysiol.1990.sp018168. [DOI] [PMC free article] [PubMed] [Google Scholar]
- Pereira A. C., Samellas D., Tiffert T., Lew V. L. Inhibition of the calcium pump by high cytosolic Ca2+ in intact human red blood cells. J Physiol. 1993 Feb;461:63–73. doi: 10.1113/jphysiol.1993.sp019501. [DOI] [PMC free article] [PubMed] [Google Scholar]
- Richards D. E. Occlusion of cobalt ions within the phosphorylated forms of the Na+-K+ pump isolated from dog kidney. J Physiol. 1988 Oct;404:497–514. doi: 10.1113/jphysiol.1988.sp017302. [DOI] [PMC free article] [PubMed] [Google Scholar]
- Schatzmann H. J., Vincenzi F. F. Calcium movements across the membrane of human red cells. J Physiol. 1969 Apr;201(2):369–395. doi: 10.1113/jphysiol.1969.sp008761. [DOI] [PMC free article] [PubMed] [Google Scholar]
- Tiffert T., Etzion Z., Bookchin R. M., Lew V. L. Effects of deoxygenation on active and passive Ca2+ transport and cytoplasmic Ca2+ buffering in normal human red cells. J Physiol. 1993 May;464:529–544. doi: 10.1113/jphysiol.1993.sp019649. [DOI] [PMC free article] [PubMed] [Google Scholar]
- Tiffert T., Garcia-Sancho J., Lew V. L. Irreversible ATP depletion caused by low concentrations of formaldehyde and of calcium-chelator esters in intact human red cells. Biochim Biophys Acta. 1984 Jun 13;773(1):143–156. doi: 10.1016/0005-2736(84)90559-5. [DOI] [PubMed] [Google Scholar]
- Wang K. K., Villalobo A., Roufogalis B. D. The plasma membrane calcium pump: a multiregulated transporter. Trends Cell Biol. 1992 Feb;2(2):46–52. doi: 10.1016/0962-8924(92)90162-g. [DOI] [PubMed] [Google Scholar]
- Wu L., Hinds T. R., Vincenzi F. F. Assay of the Ca pump ATPase activity of intact red blood cells. Biochim Biophys Acta. 1992 Apr 29;1106(1):56–62. doi: 10.1016/0005-2736(92)90221-7. [DOI] [PubMed] [Google Scholar]
- Xu Y. H., Roufogalis B. D. Asymmetric effects of divalent cations and protons on active Ca2+ efflux and Ca2+-ATPase in intact red blood cells. J Membr Biol. 1988 Oct;105(2):155–164. doi: 10.1007/BF02009168. [DOI] [PubMed] [Google Scholar]