Abstract
1. The development of Ca2+ and K+ currents was studied in ascidian muscle cells at twelve embryonic stages from gastrulation to the mature cell, a period of 24 h. A high degree of co-ordination occurs between the development of the inwardly rectifying K+ current (IK(IR)), which sets the resting potential, and Ca2+ and outward K+ currents, which determine action potential waveform. 2. At neurulation IK(IR), which had been present since fertilization, begins to decrease, reaching 12% of its previous density in 6 h. IK(IR) then immediately begins to increase again, reaching its previous density in another 6 h. 3. When IK(IR) begins to decrease, a high-threshold inactivating Ca2+ current and a slowly activating voltage-gated K+ current appear. 4. When IK(IR) returns to its previous density, two new currents appear: a sustained Ca2+ current with the same voltage dependence, but different conotoxin sensitivity than the inactivating Ca2+ current; and a Ca(2+)-dependent K+ current, which activates 8-10 times faster and at potentials 20-30 mV more negative than the voltage-dependent K+ current. 5. The transient downregulation of IK(IR) destabilizes the resting potential and causes spontaneous action potentials to occur. Because IK(IR) is absent when only a slowly activating high-threshold outward K+ current is present, these action potentials are long in duration. 6. The return of IK(IR) and the appearance of the rapidly activating Ca(2+)-dependent K+ current eventually terminate this activity. The action potentials of the mature cell occur only on stimulation, and are 10 times shorter in duration than those in the immature cell.
Full text
PDF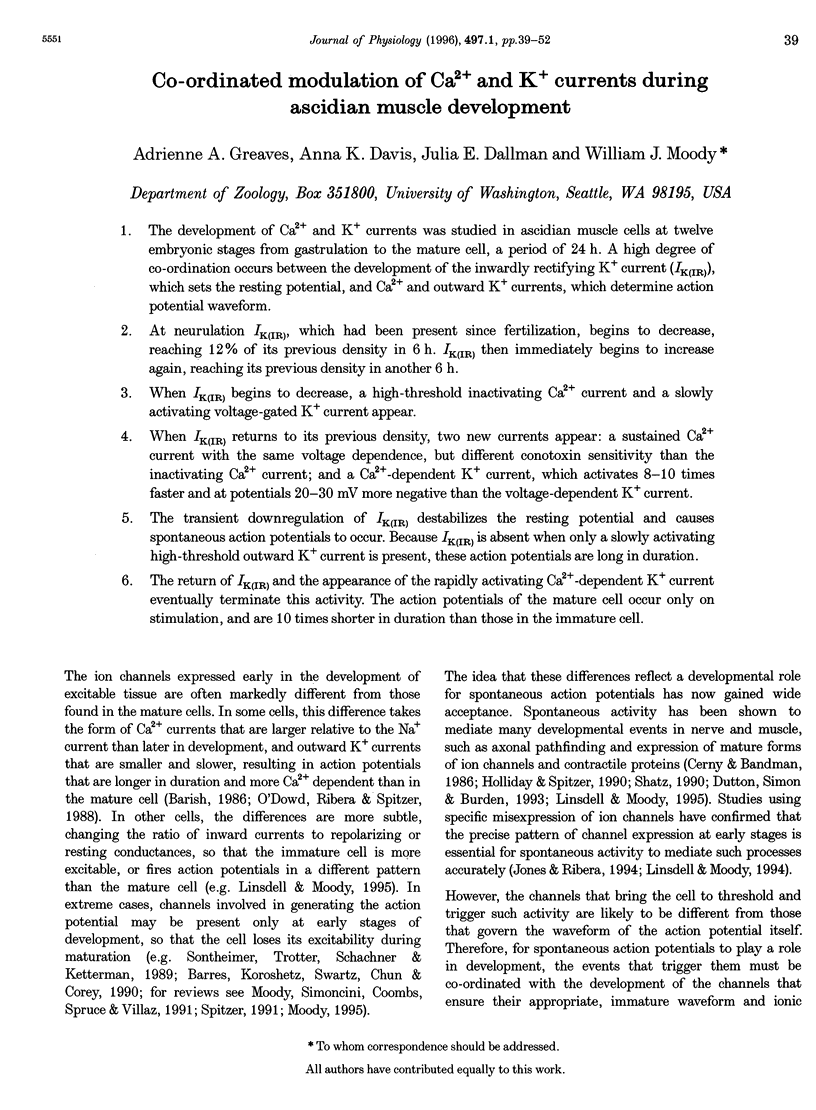
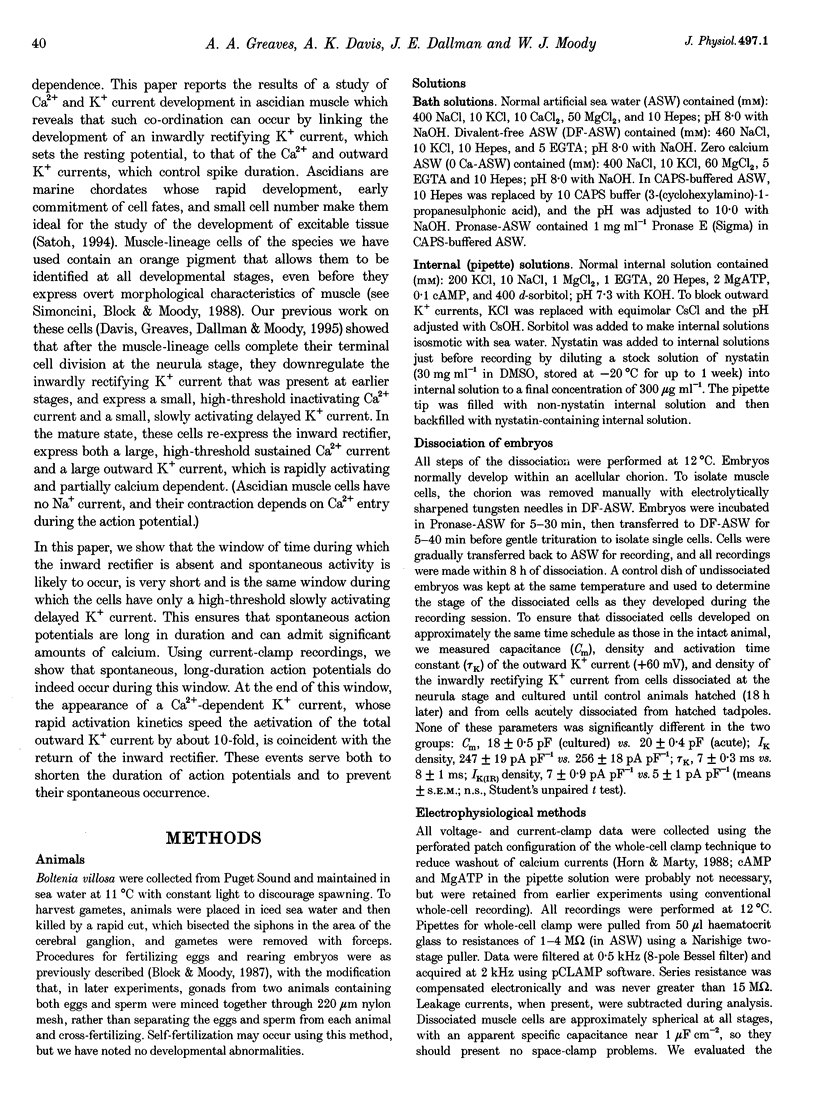
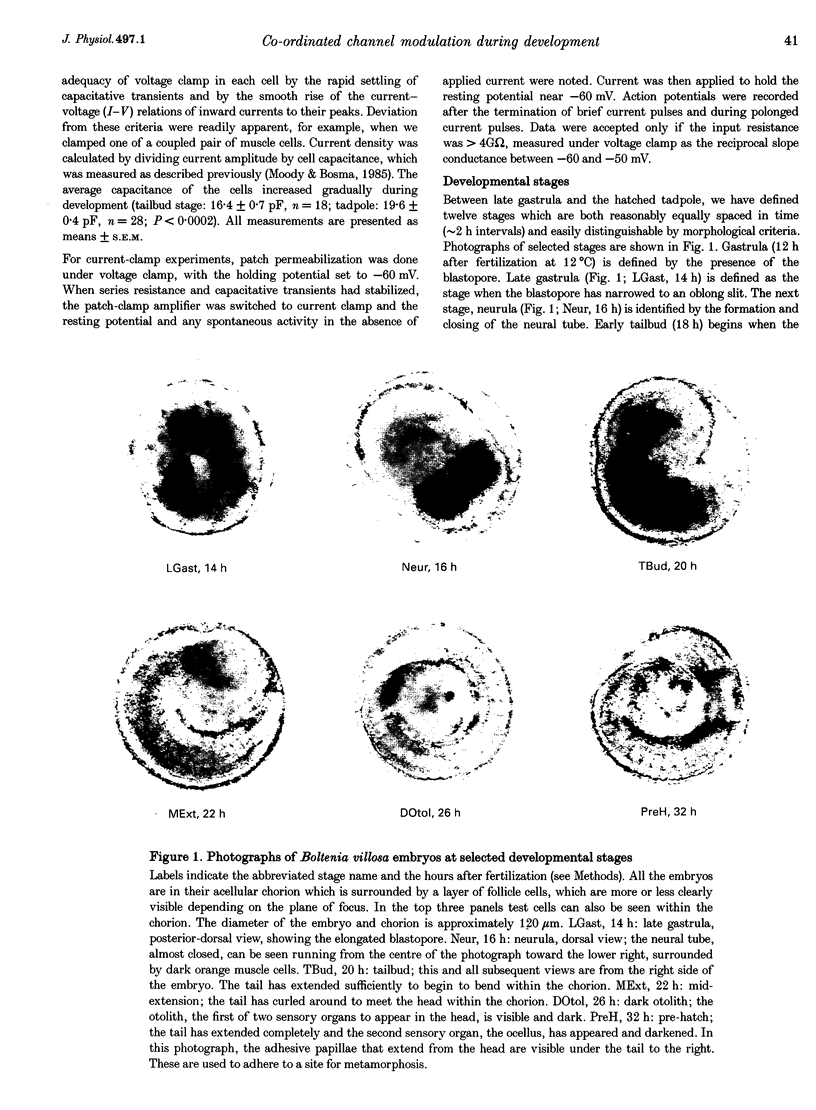
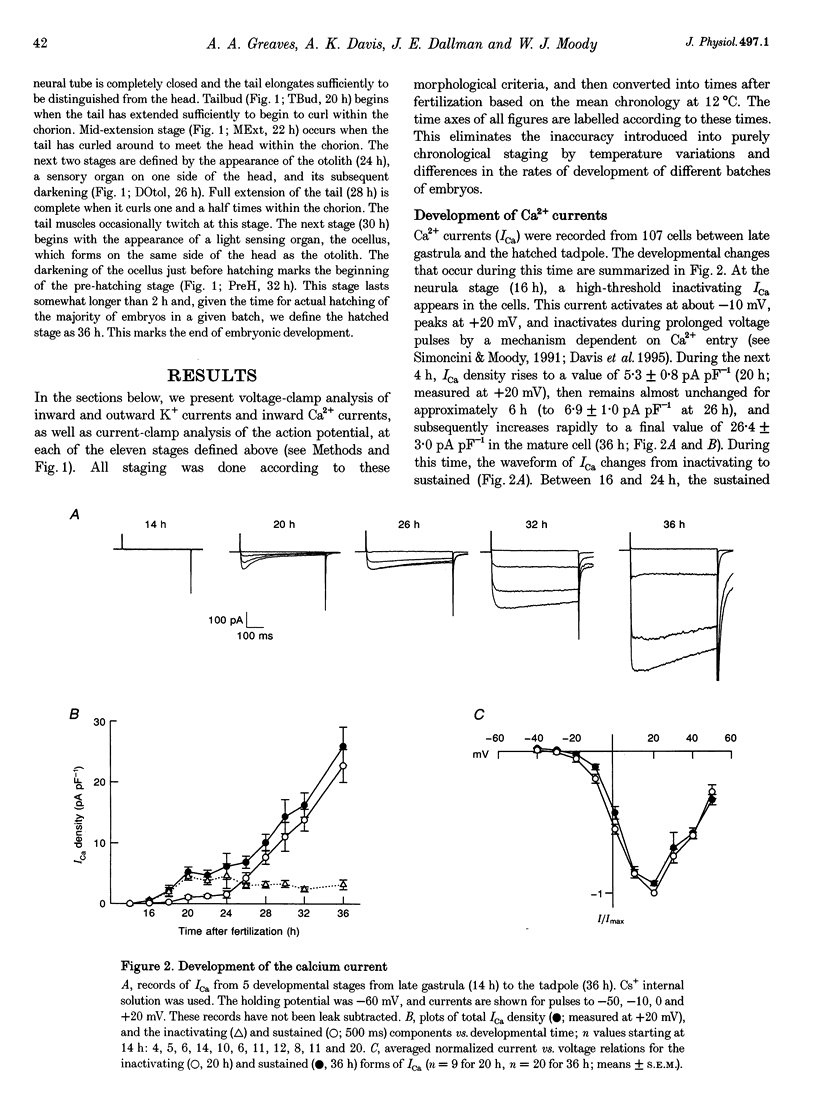
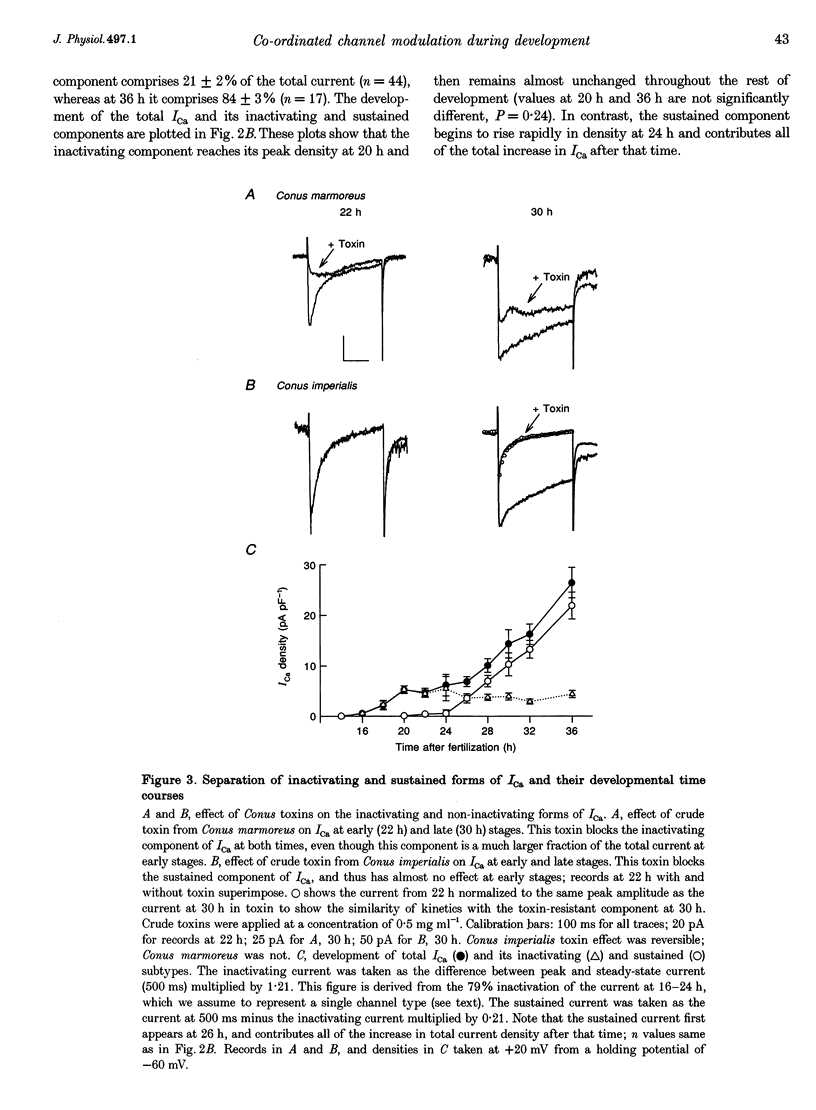
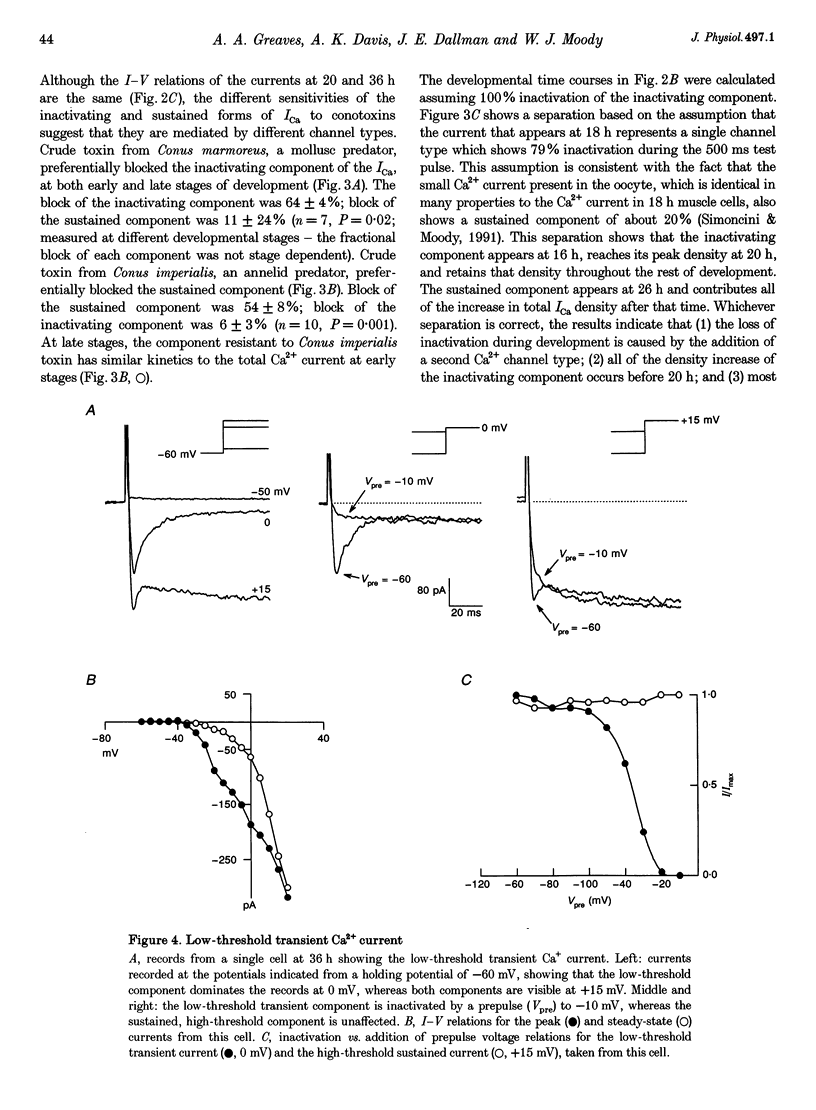
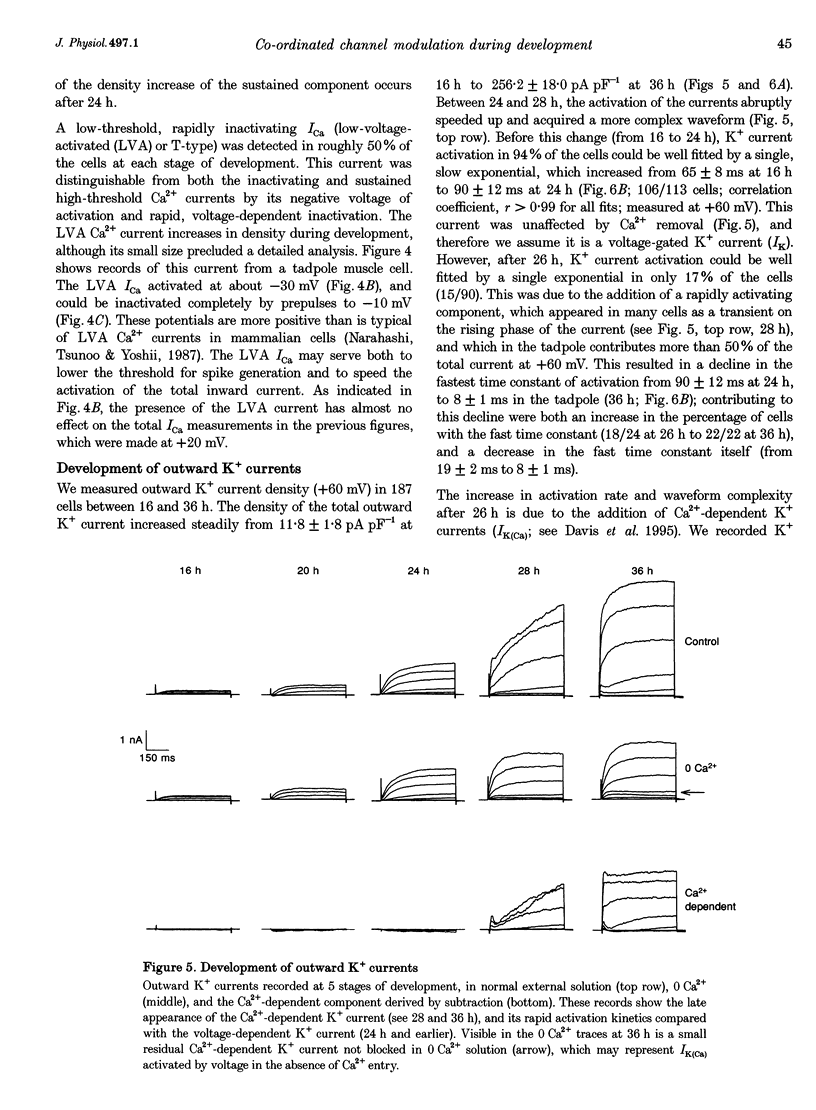
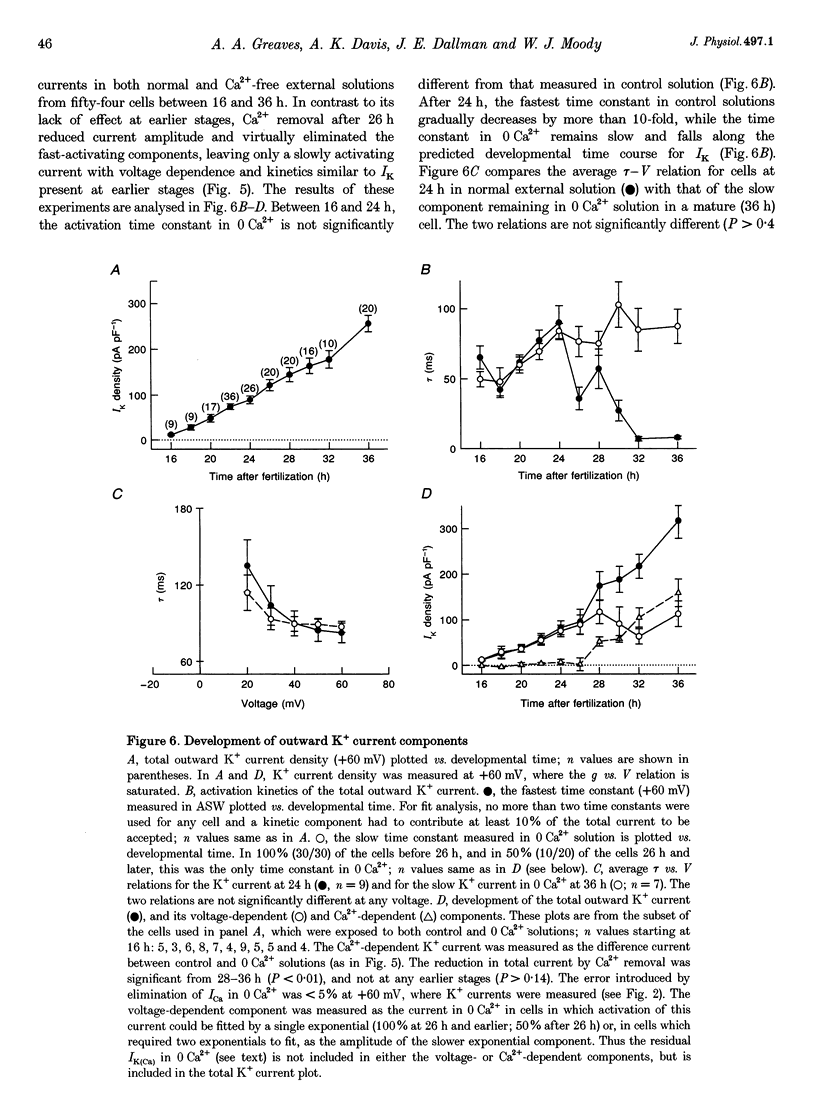
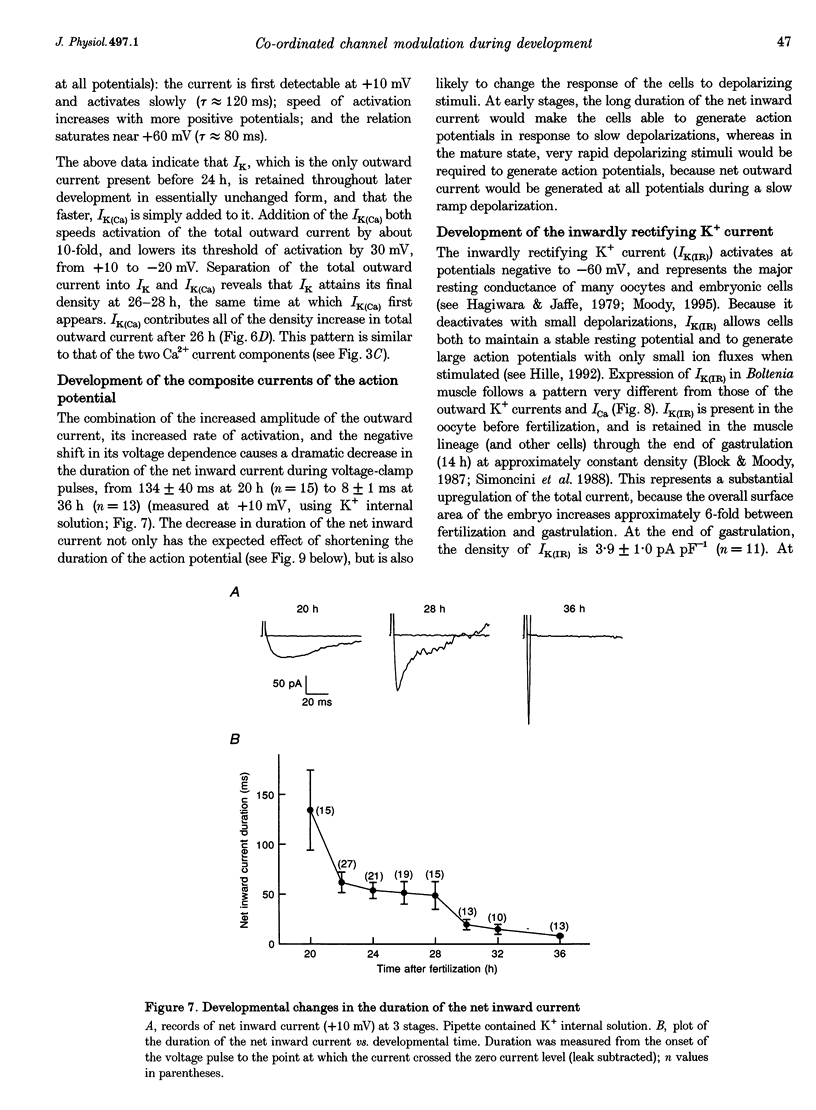
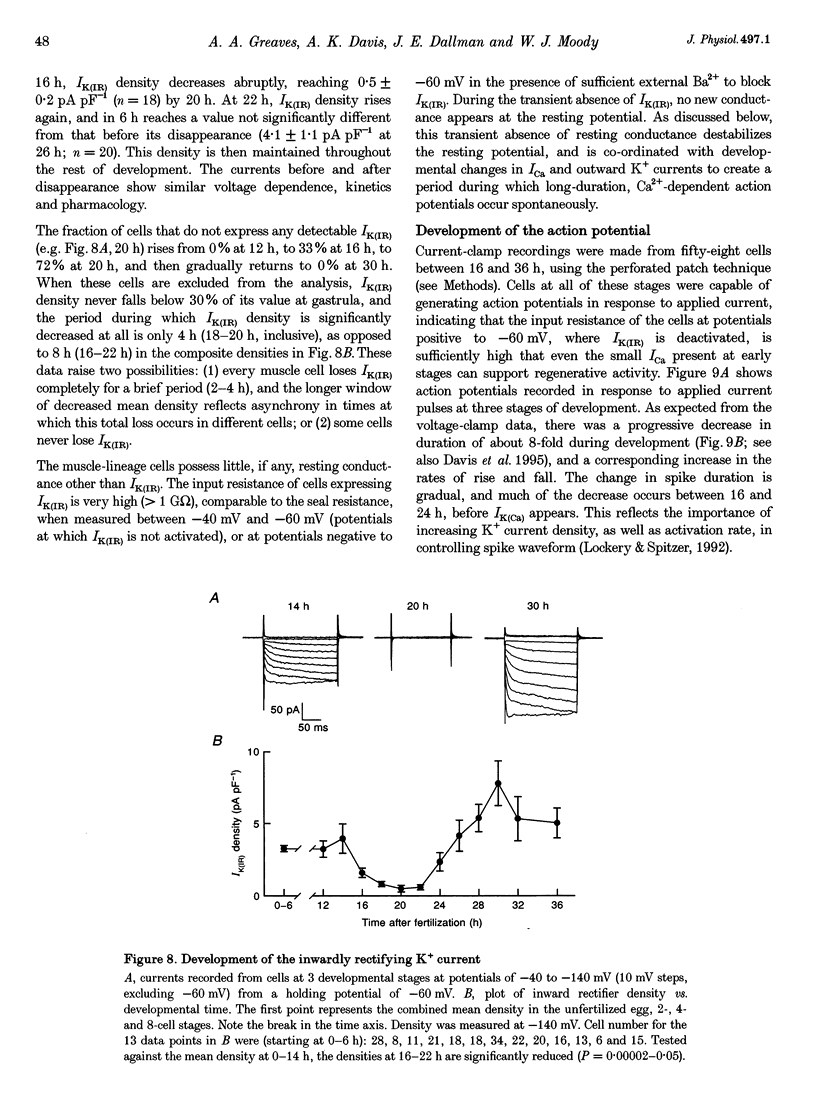
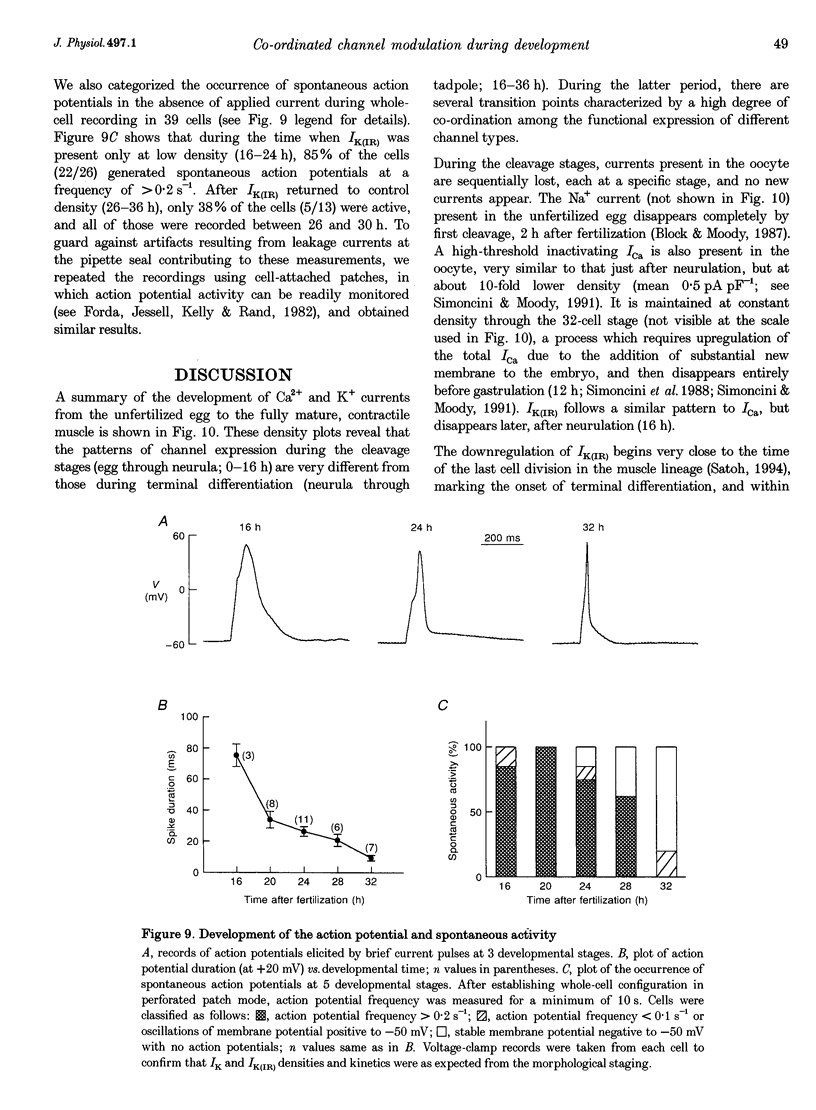
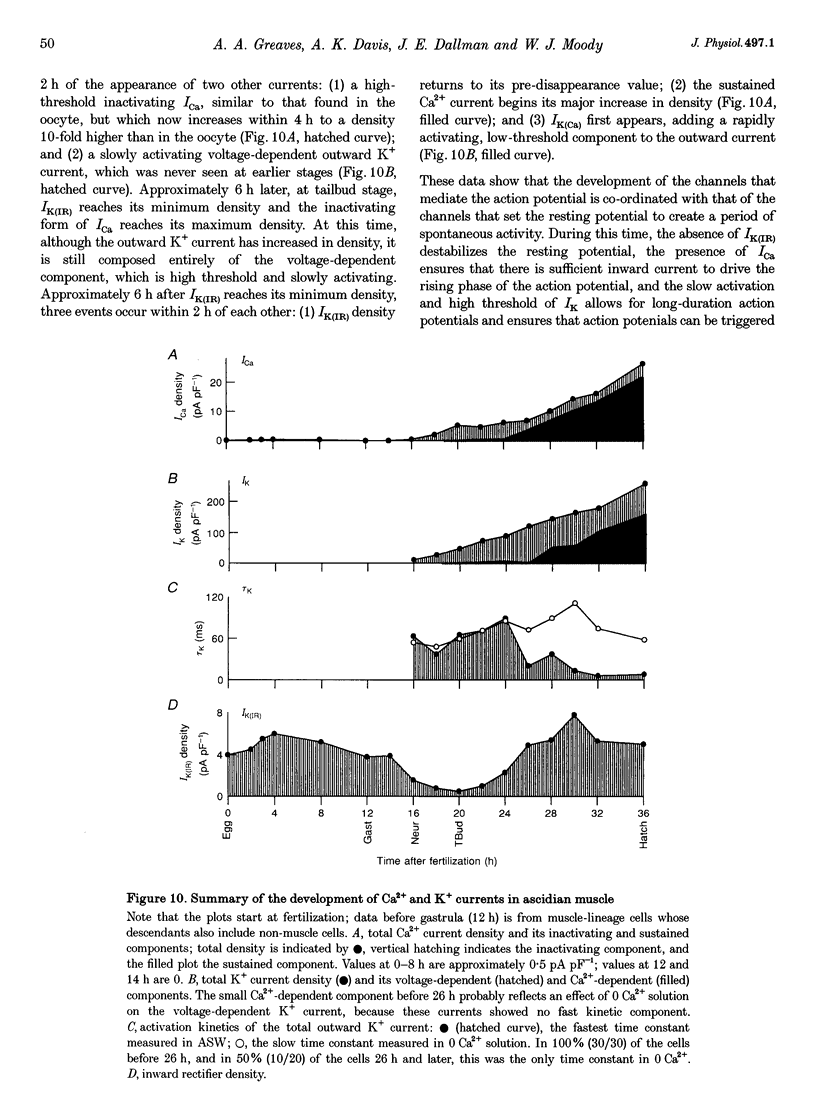
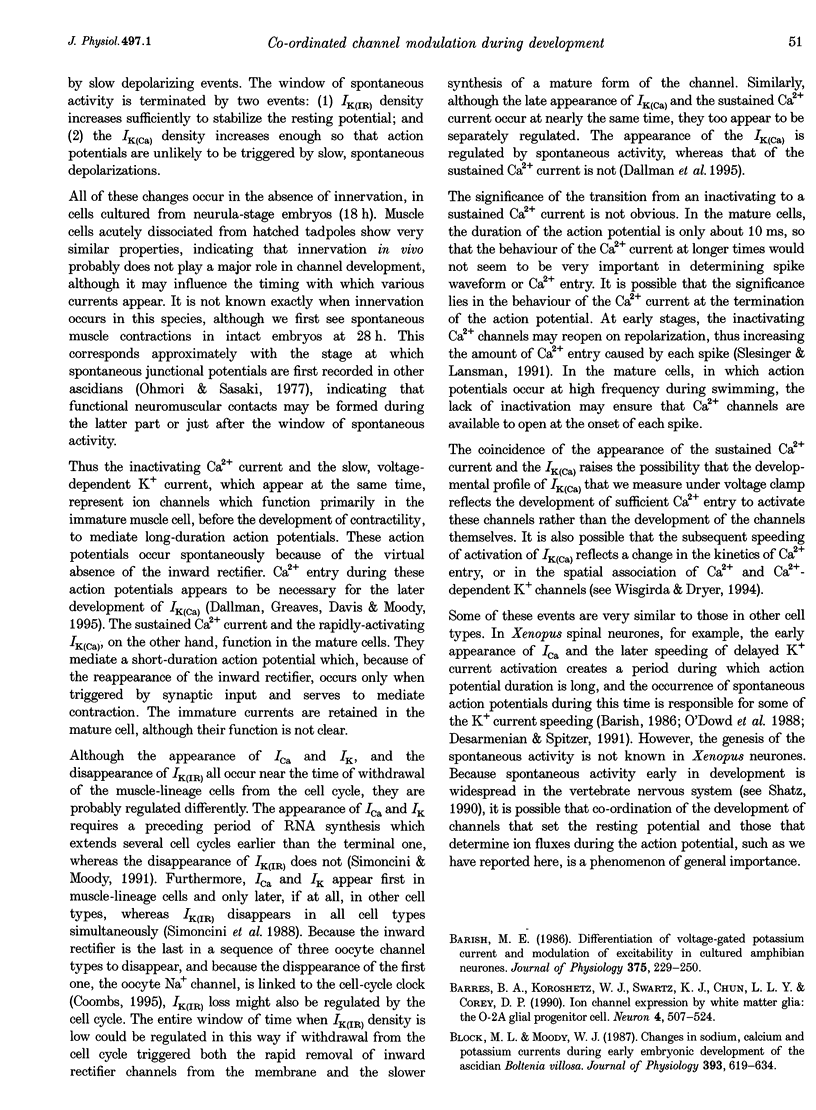
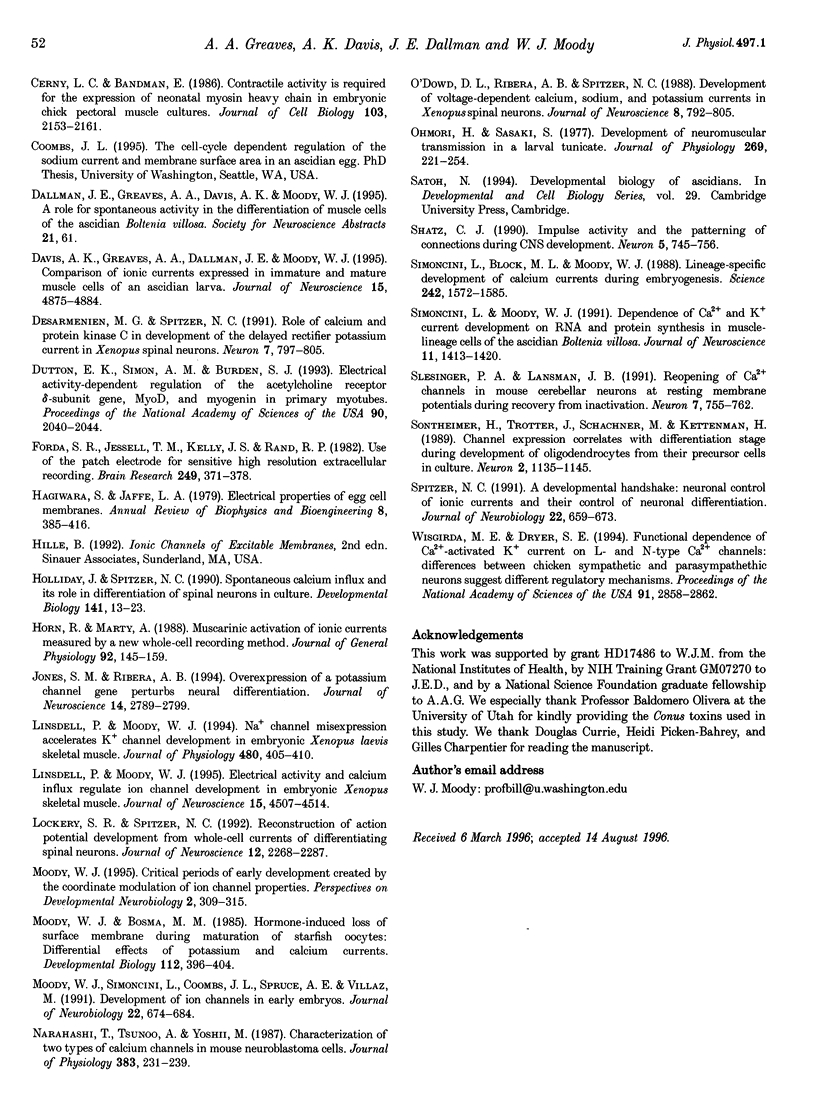
Images in this article
Selected References
These references are in PubMed. This may not be the complete list of references from this article.
- Barish M. E. Differentiation of voltage-gated potassium current and modulation of excitability in cultured amphibian spinal neurones. J Physiol. 1986 Jun;375:229–250. doi: 10.1113/jphysiol.1986.sp016114. [DOI] [PMC free article] [PubMed] [Google Scholar]
- Barres B. A., Koroshetz W. J., Swartz K. J., Chun L. L., Corey D. P. Ion channel expression by white matter glia: the O-2A glial progenitor cell. Neuron. 1990 Apr;4(4):507–524. doi: 10.1016/0896-6273(90)90109-s. [DOI] [PubMed] [Google Scholar]
- Block M. L., Moody W. J. Changes in sodium, calcium and potassium currents during early embryonic development of the ascidian Boltenia villosa. J Physiol. 1987 Dec;393:619–634. doi: 10.1113/jphysiol.1987.sp016844. [DOI] [PMC free article] [PubMed] [Google Scholar]
- Cerny L. C., Bandman E. Contractile activity is required for the expression of neonatal myosin heavy chain in embryonic chick pectoral muscle cultures. J Cell Biol. 1986 Dec;103(6 Pt 1):2153–2161. doi: 10.1083/jcb.103.6.2153. [DOI] [PMC free article] [PubMed] [Google Scholar]
- Davis A. K., Greaves A. A., Dallman J. E., Moody W. J. Comparison of ionic currents expressed in immature and mature muscle cells of an ascidian larva. J Neurosci. 1995 Jul;15(7 Pt 1):4875–4884. doi: 10.1523/JNEUROSCI.15-07-04875.1995. [DOI] [PMC free article] [PubMed] [Google Scholar]
- Desarmenien M. G., Spitzer N. C. Role of calcium and protein kinase C in development of the delayed rectifier potassium current in Xenopus spinal neurons. Neuron. 1991 Nov;7(5):797–805. doi: 10.1016/0896-6273(91)90282-5. [DOI] [PubMed] [Google Scholar]
- Dutton E. K., Simon A. M., Burden S. J. Electrical activity-dependent regulation of the acetylcholine receptor delta-subunit gene, MyoD, and myogenin in primary myotubes. Proc Natl Acad Sci U S A. 1993 Mar 1;90(5):2040–2044. doi: 10.1073/pnas.90.5.2040. [DOI] [PMC free article] [PubMed] [Google Scholar]
- Forda S. R., Jessell T. M., Kelly J. S., Rand R. P. Use of the patch electrode for sensitive high resolution extracellular recording. Brain Res. 1982 Oct 14;249(2):371–378. doi: 10.1016/0006-8993(82)90071-3. [DOI] [PubMed] [Google Scholar]
- Hagiwara S., Jaffe L. A. Electrical properties of egg cell membranes. Annu Rev Biophys Bioeng. 1979;8:385–416. doi: 10.1146/annurev.bb.08.060179.002125. [DOI] [PubMed] [Google Scholar]
- Holliday J., Spitzer N. C. Spontaneous calcium influx and its roles in differentiation of spinal neurons in culture. Dev Biol. 1990 Sep;141(1):13–23. doi: 10.1016/0012-1606(90)90098-4. [DOI] [PubMed] [Google Scholar]
- Horn R., Marty A. Muscarinic activation of ionic currents measured by a new whole-cell recording method. J Gen Physiol. 1988 Aug;92(2):145–159. doi: 10.1085/jgp.92.2.145. [DOI] [PMC free article] [PubMed] [Google Scholar]
- Jones S. M., Ribera A. B. Overexpression of a potassium channel gene perturbs neural differentiation. J Neurosci. 1994 May;14(5 Pt 1):2789–2799. doi: 10.1523/JNEUROSCI.14-05-02789.1994. [DOI] [PMC free article] [PubMed] [Google Scholar]
- Linsdell P., Moody W. J. Electrical activity and calcium influx regulate ion channel development in embryonic Xenopus skeletal muscle. J Neurosci. 1995 Jun;15(6):4507–4514. doi: 10.1523/JNEUROSCI.15-06-04507.1995. [DOI] [PMC free article] [PubMed] [Google Scholar]
- Linsdell P., Moody W. J. Na+ channel mis-expression accelerates K+ channel development in embryonic Xenopus laevis skeletal muscle. J Physiol. 1994 Nov 1;480(Pt 3):405–410. doi: 10.1113/jphysiol.1994.sp020370. [DOI] [PMC free article] [PubMed] [Google Scholar]
- Lockery S. R., Spitzer N. C. Reconstruction of action potential development from whole-cell currents of differentiating spinal neurons. J Neurosci. 1992 Jun;12(6):2268–2287. doi: 10.1523/JNEUROSCI.12-06-02268.1992. [DOI] [PMC free article] [PubMed] [Google Scholar]
- Moody W. J., Bosma M. M. Hormone-induced loss of surface membrane during maturation of starfish oocytes: differential effects on potassium and calcium channels. Dev Biol. 1985 Dec;112(2):396–404. doi: 10.1016/0012-1606(85)90412-9. [DOI] [PubMed] [Google Scholar]
- Moody W. J. Critical periods of early development created by the coordinate modulation of ion channel properties. Perspect Dev Neurobiol. 1995;2(4):309–315. [PubMed] [Google Scholar]
- Moody W. J., Simoncini L., Coombs J. L., Spruce A. E., Villaz M. Development of ion channels in early embryos. J Neurobiol. 1991 Oct;22(7):674–684. doi: 10.1002/neu.480220703. [DOI] [PubMed] [Google Scholar]
- Narahashi T., Tsunoo A., Yoshii M. Characterization of two types of calcium channels in mouse neuroblastoma cells. J Physiol. 1987 Feb;383:231–249. doi: 10.1113/jphysiol.1987.sp016406. [DOI] [PMC free article] [PubMed] [Google Scholar]
- O'Dowd D. K., Ribera A. B., Spitzer N. C. Development of voltage-dependent calcium, sodium, and potassium currents in Xenopus spinal neurons. J Neurosci. 1988 Mar;8(3):792–805. doi: 10.1523/JNEUROSCI.08-03-00792.1988. [DOI] [PMC free article] [PubMed] [Google Scholar]
- Ohmori H., Sasaki S. Development of neuromuscular transmission in a larval tunicate. J Physiol. 1977 Jul;269(2):221–254. doi: 10.1113/jphysiol.1977.sp011900. [DOI] [PMC free article] [PubMed] [Google Scholar]
- Shatz C. J. Impulse activity and the patterning of connections during CNS development. Neuron. 1990 Dec;5(6):745–756. doi: 10.1016/0896-6273(90)90333-b. [DOI] [PubMed] [Google Scholar]
- Simoncini L., Block M. L., Moody W. J. Lineage-specific development of calcium currents during embryogenesis. Science. 1988 Dec 16;242(4885):1572–1575. doi: 10.1126/science.2849207. [DOI] [PubMed] [Google Scholar]
- Simoncini L., Moody W. J. Dependence of Ca2+ and K+ current development on RNA and protein synthesis in muscle-lineage cells of the ascidian Boltenia villosa. J Neurosci. 1991 May;11(5):1413–1420. doi: 10.1523/JNEUROSCI.11-05-01413.1991. [DOI] [PMC free article] [PubMed] [Google Scholar]
- Slesinger P. A., Lansman J. B. Reopening of Ca2+ channels in mouse cerebellar neurons at resting membrane potentials during recovery from inactivation. Neuron. 1991 Nov;7(5):755–762. doi: 10.1016/0896-6273(91)90278-8. [DOI] [PubMed] [Google Scholar]
- Sontheimer H., Trotter J., Schachner M., Kettenmann H. Channel expression correlates with differentiation stage during the development of oligodendrocytes from their precursor cells in culture. Neuron. 1989 Feb;2(2):1135–1145. doi: 10.1016/0896-6273(89)90180-3. [DOI] [PubMed] [Google Scholar]
- Spitzer N. C. A developmental handshake: neuronal control of ionic currents and their control of neuronal differentiation. J Neurobiol. 1991 Oct;22(7):659–673. doi: 10.1002/neu.480220702. [DOI] [PubMed] [Google Scholar]
- Wisgirda M. E., Dryer S. E. Functional dependence of Ca(2+)-activated K+ current on L- and N-type Ca2+ channels: differences between chicken sympathetic and parasympathetic neurons suggest different regulatory mechanisms. Proc Natl Acad Sci U S A. 1994 Mar 29;91(7):2858–2862. doi: 10.1073/pnas.91.7.2858. [DOI] [PMC free article] [PubMed] [Google Scholar]