Abstract
1. Whole-cell patch clamp studies were carried out on Schwann cells in organotypic cultures of dorsal root ganglia (DRG) from OF1 mice embryos (18-19 days). 2. In standard external solution, from a holding potential of -70 mV, two types of voltage-dependent K+ currents were recorded: a fast transient current and a delayed sustained current. With a holding potential of -30 mV, only the delayed sustained current could be evoked. 3. Both K+ currents were inhibited by tetraethylammonium chloride (TEA) and 4-aminopyridine (4-AP) in a dose-dependent manner. For the transient current the half-maximal effective dose was 100 mM for TEA and 1.3 mM for 4-AP. For the delayed sustained current the half-maximal effective dose was 11 mM for TEA and 4 mM for 4-AP. Both currents were insensitive to external Ca2+. 4. The delayed sustained current, isolated by use of a holding potential of -30 mV displayed a 'cumulative inactivation' which was removed by hyperpolarizing the membrane to -70 mV between each test pulse. 5. In K(+)-free external and pipette solutions, with 10 mM-external Ca2+, from a holding potential of -70 mV voltage-dependent Ca2+ channel currents were recorded. The threshold for activation was -45.3 +/- 5.4 mV (mean +/- S.D., n = 5) and the current inactivated fully at the end of the test potential. The current was unaffected by 2 microM-tetrodotoxin (TTX) and totally blocked by 5 mM-Co2+. 6. Equimolar replacement of external Ca2+ by Ba2+ did not significantly modify the voltage dependence (threshold for activation -42.8 +/- 6.4 mV, n = 7) or the magnitude of the inward current. Ca2+ and Ba2+ were equally permeant. The fully inactivating current was insensitive to both nifedipine and Bay K 8644 (1 microM each). Increasing the external Ba2+ concentration from 10 to 89 mM enhanced the Ba2+ current and shifted the voltage dependence of the current (threshold for activation, -30.5 +/- 7.3 mV, n = 9) along the voltage axis as expected for altered external surface potential. 7. In 89 mM-external Ba2+ solution, some cells displayed an additional slowly decaying current which was totally blocked by nifedipine (1 microM). 8. Ca2+ channel currents were recorded only when DRG neurons were present in the culture, as excision of explants and subsequent axonal degeneration led to loss of detectable Ca2+ channel currents. This phenomenon was never observed for K+ currents. 9. We conclude that mouse Schwann cells in organotypic culture possess voltage-dependent K+ and Ca2+ channels.(ABSTRACT TRUNCATED AT 400 WORDS)
Full text
PDF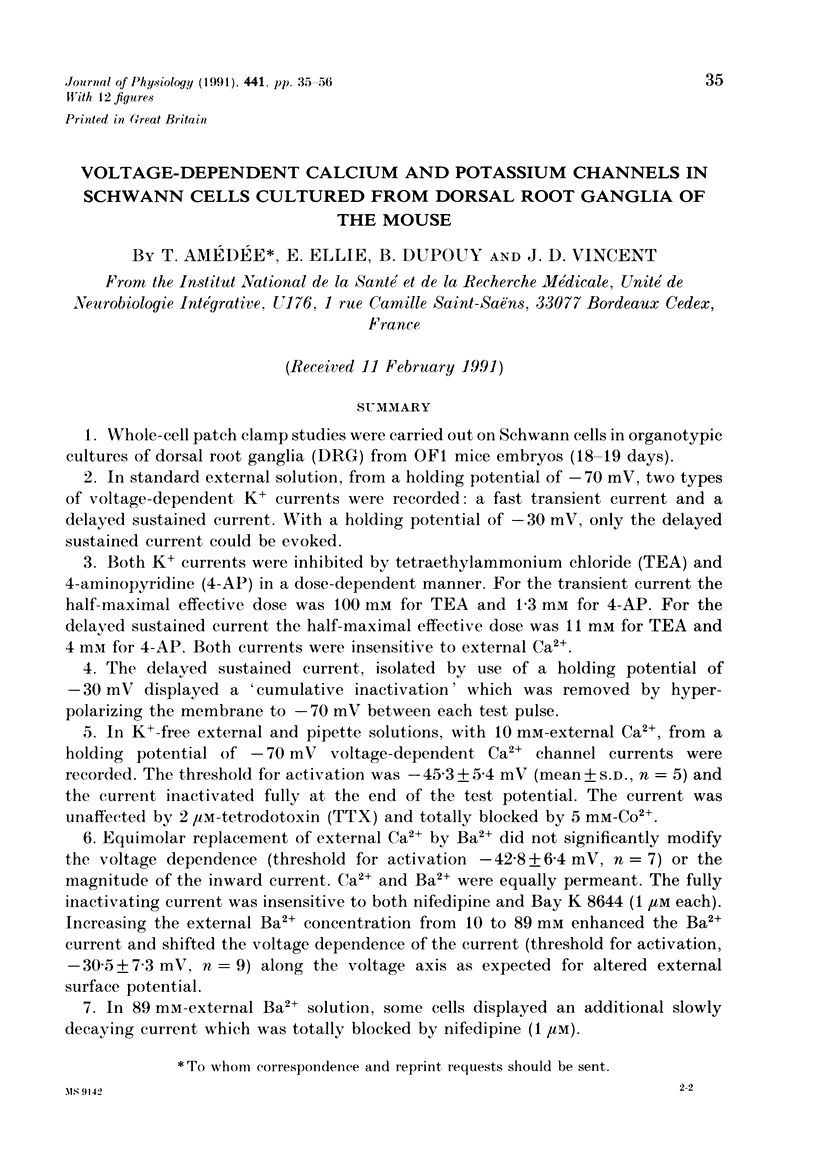
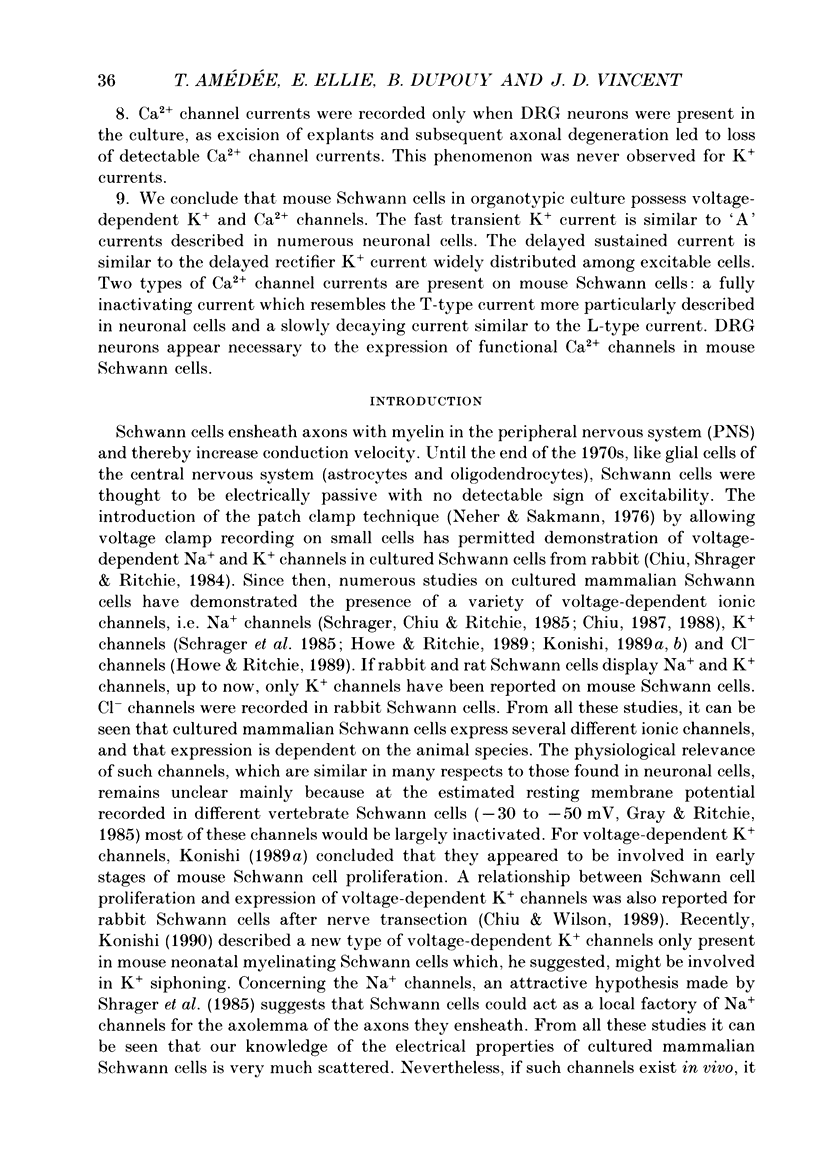
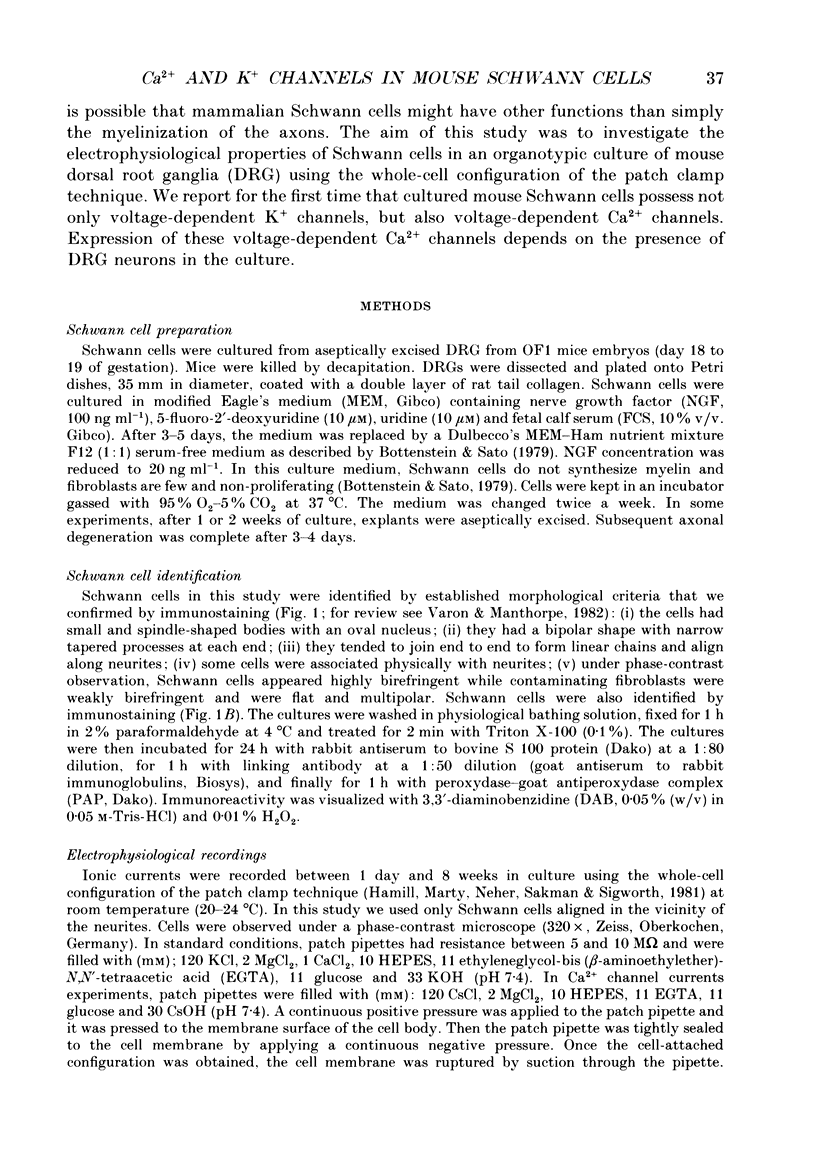
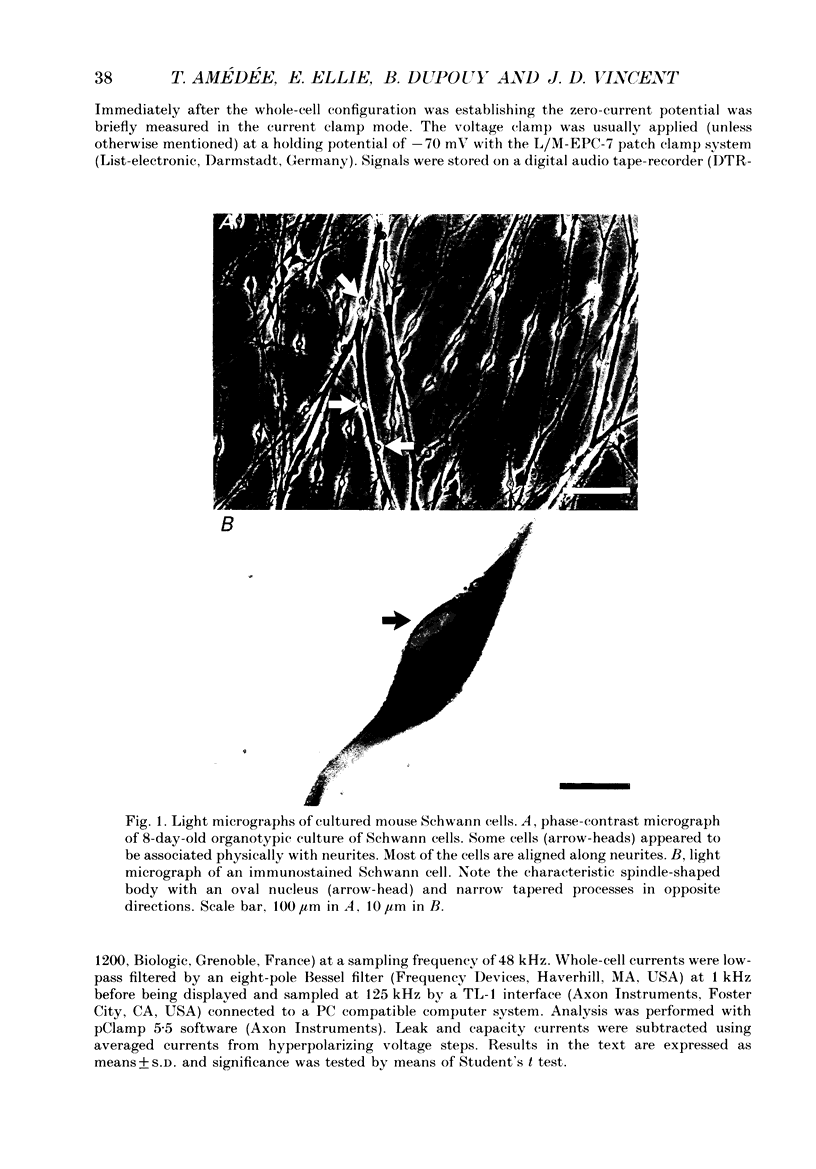
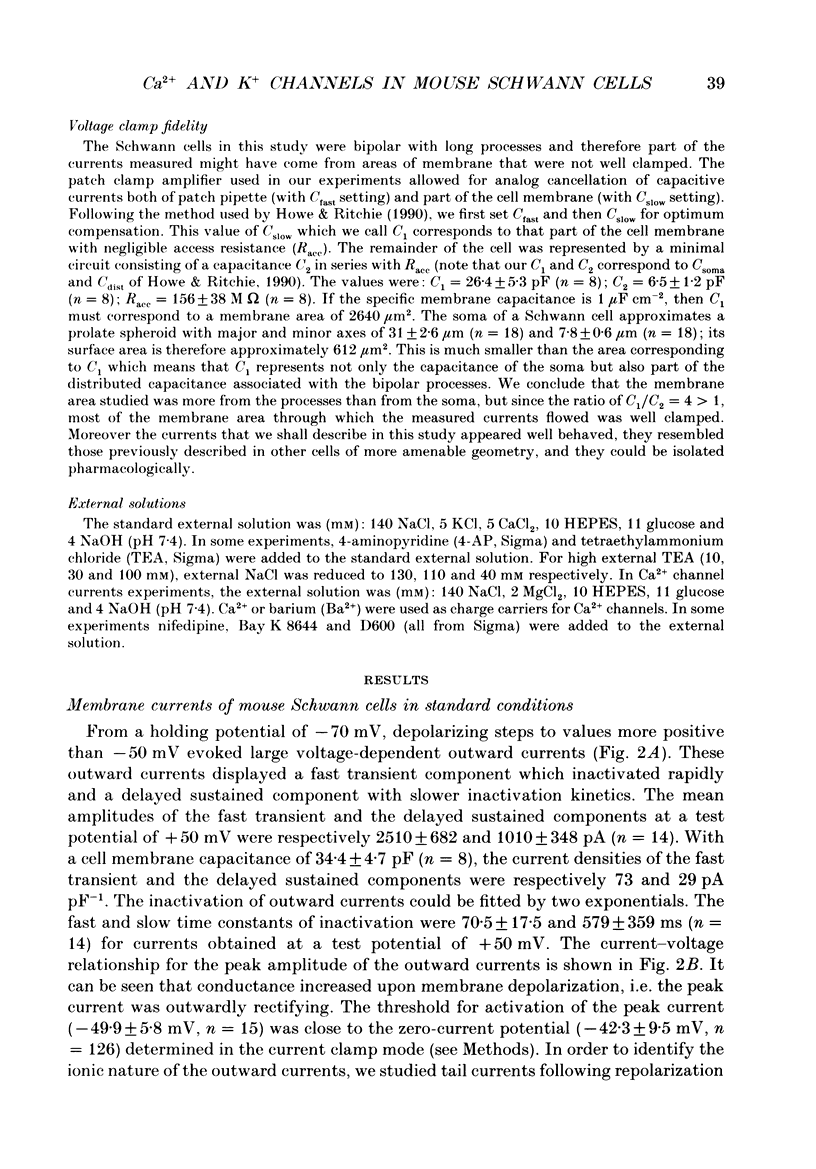
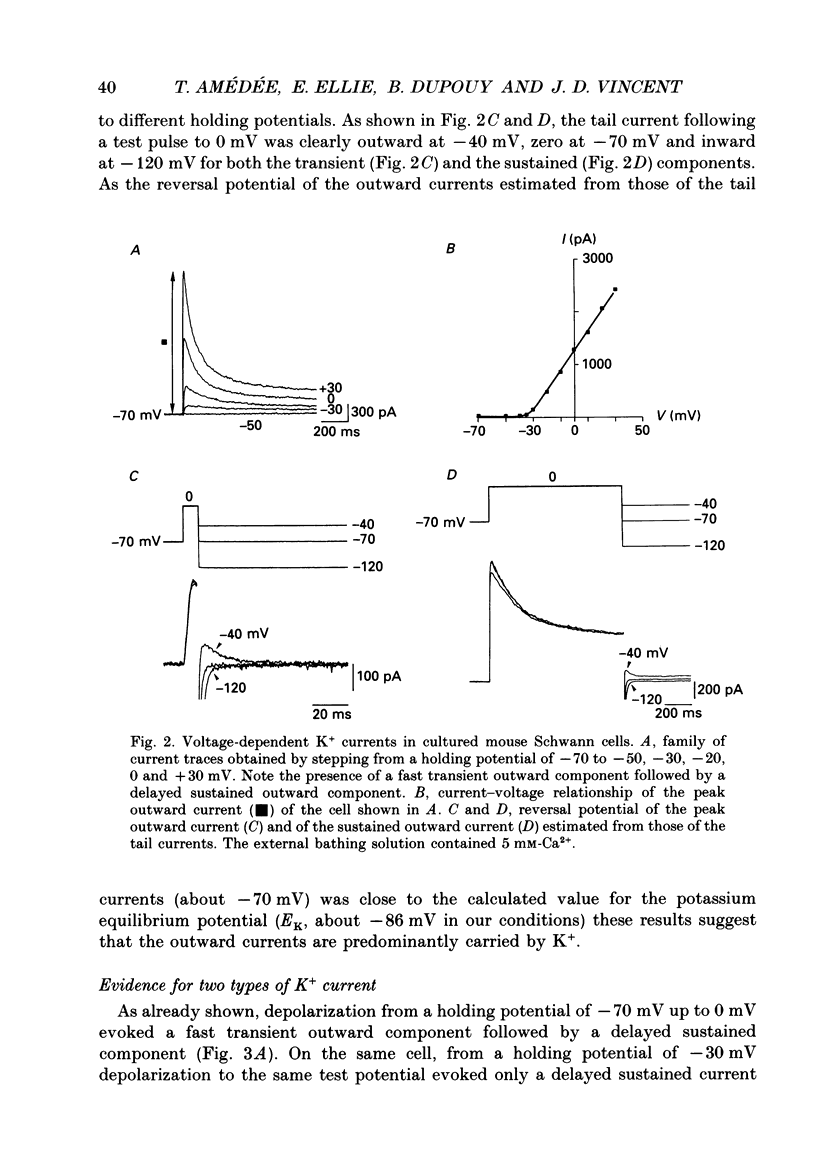
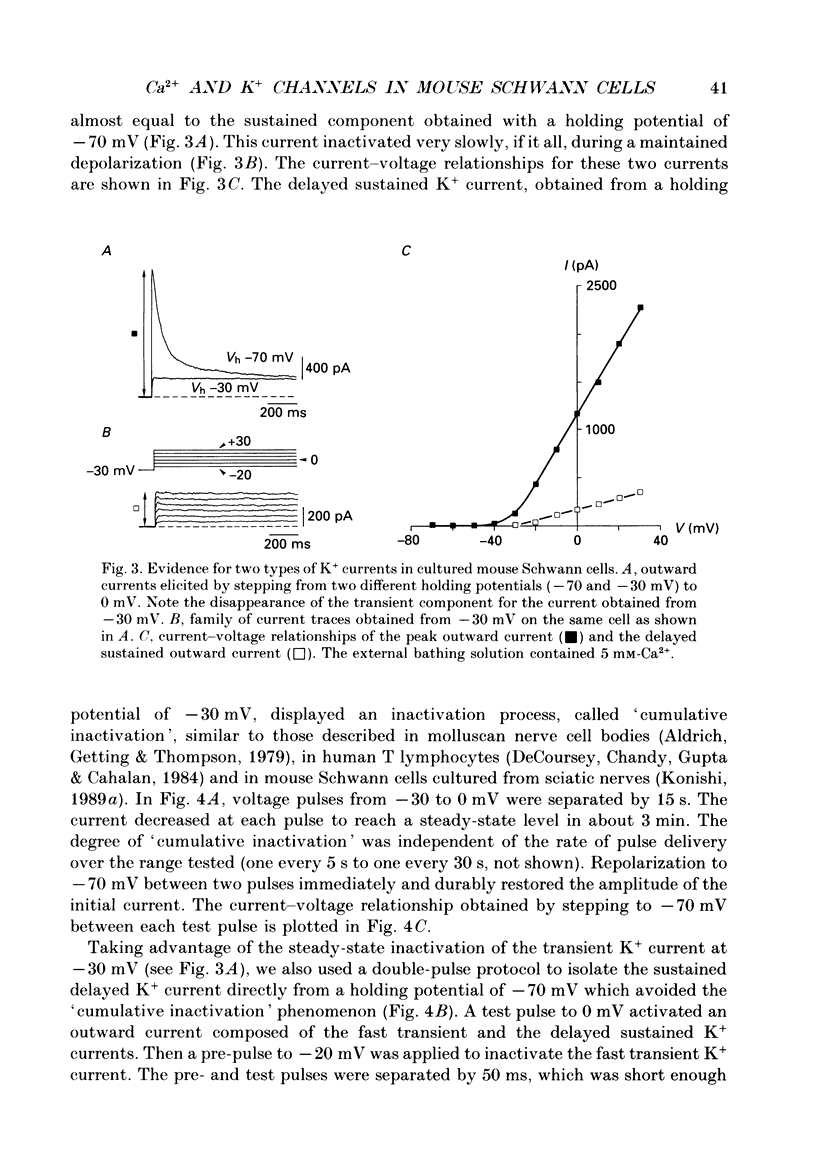
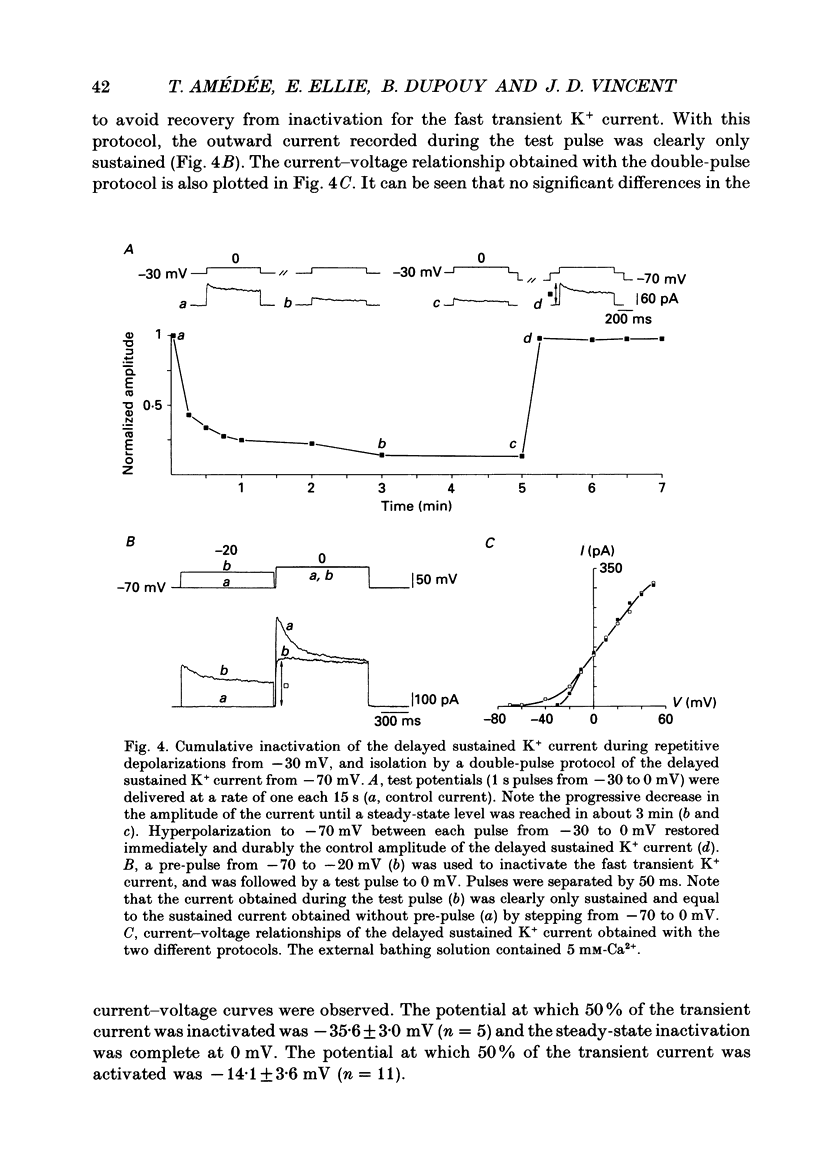
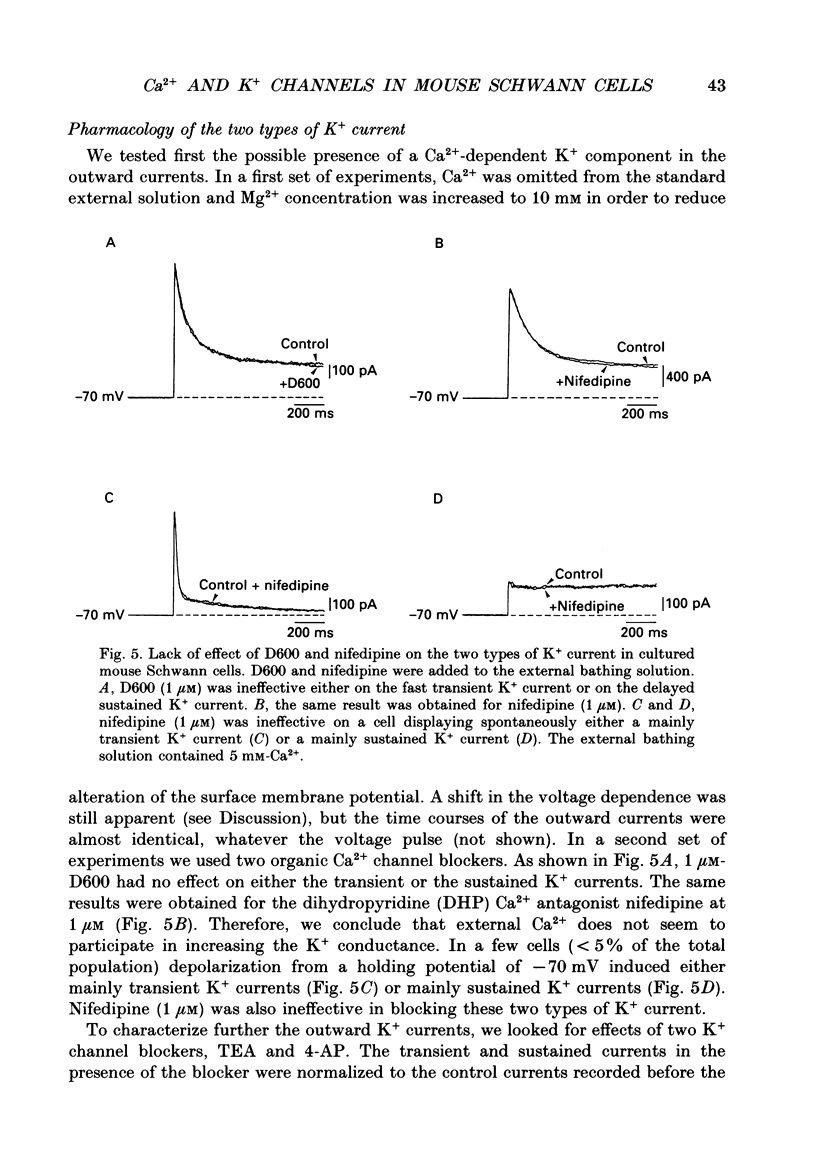
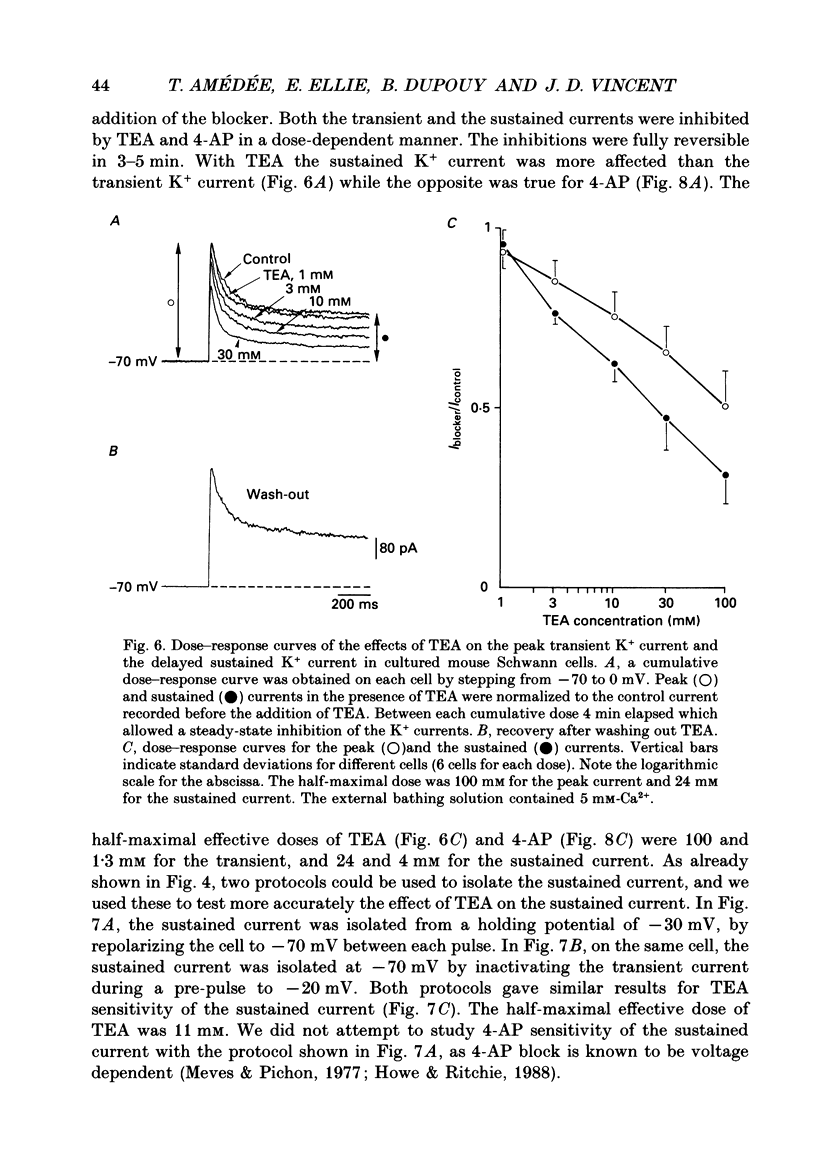
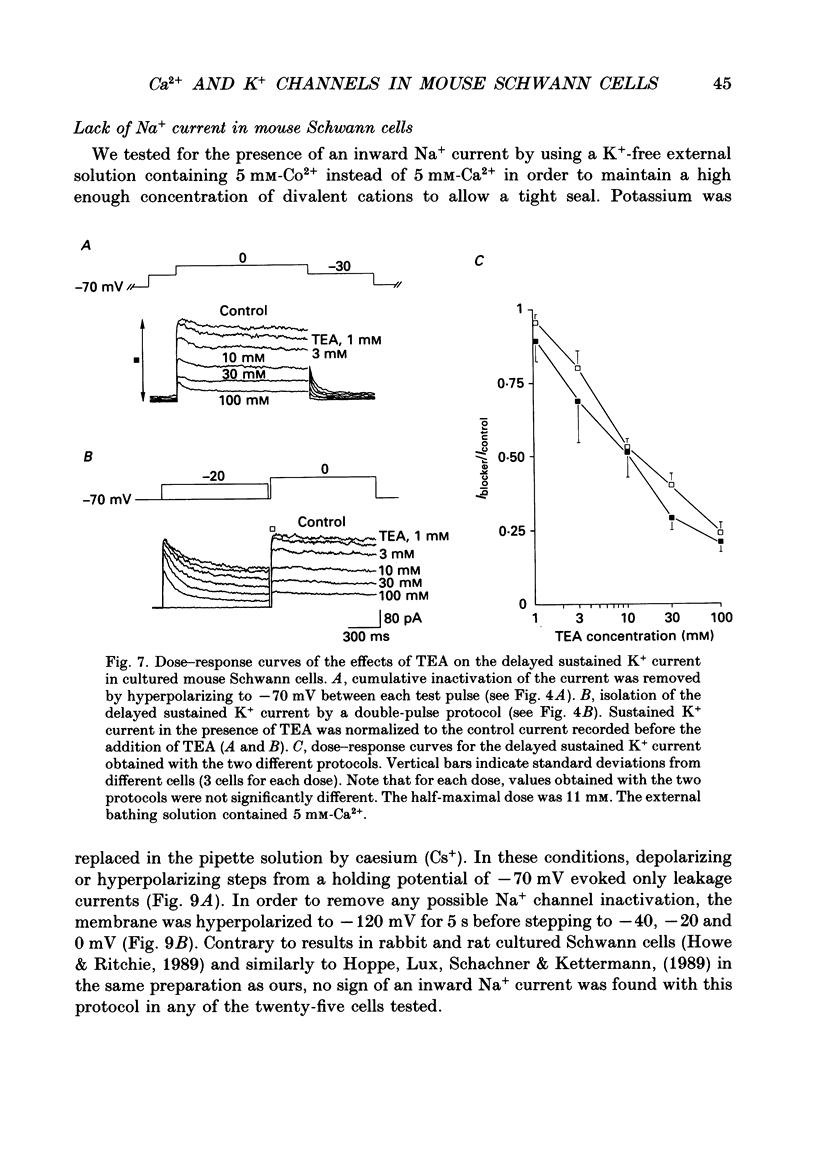
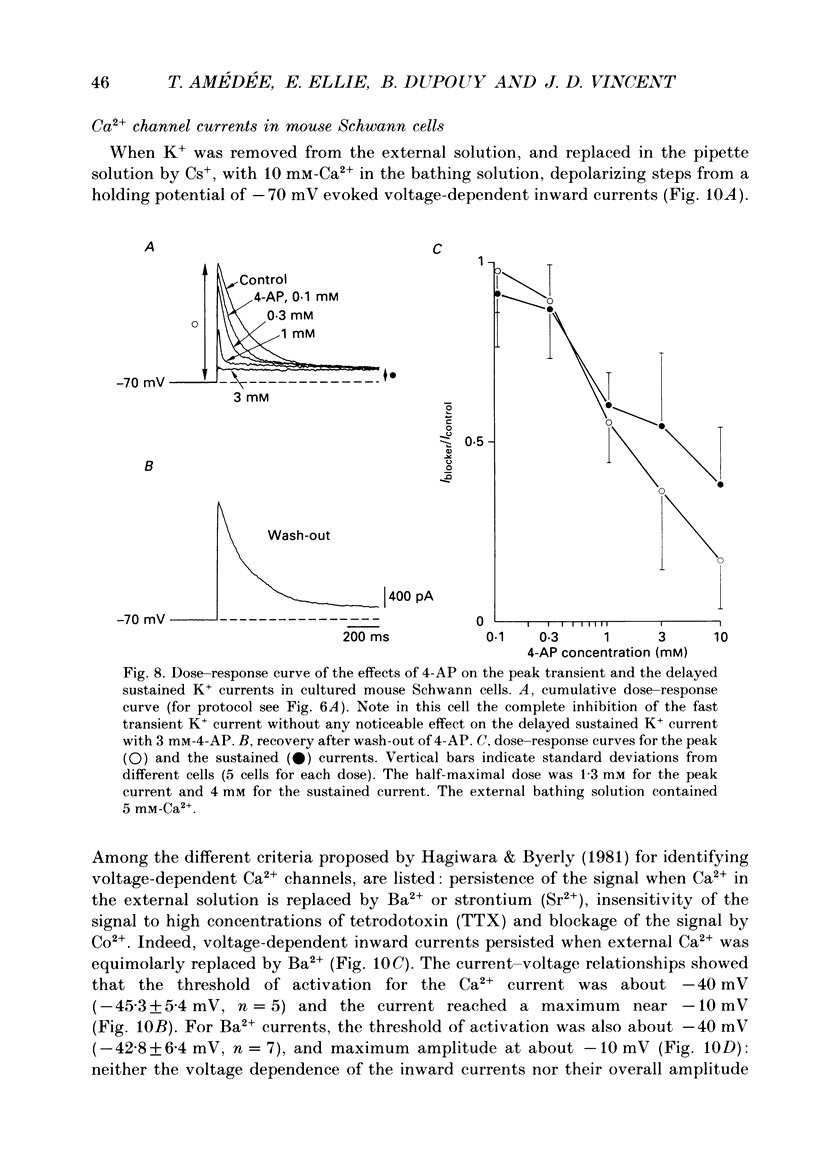
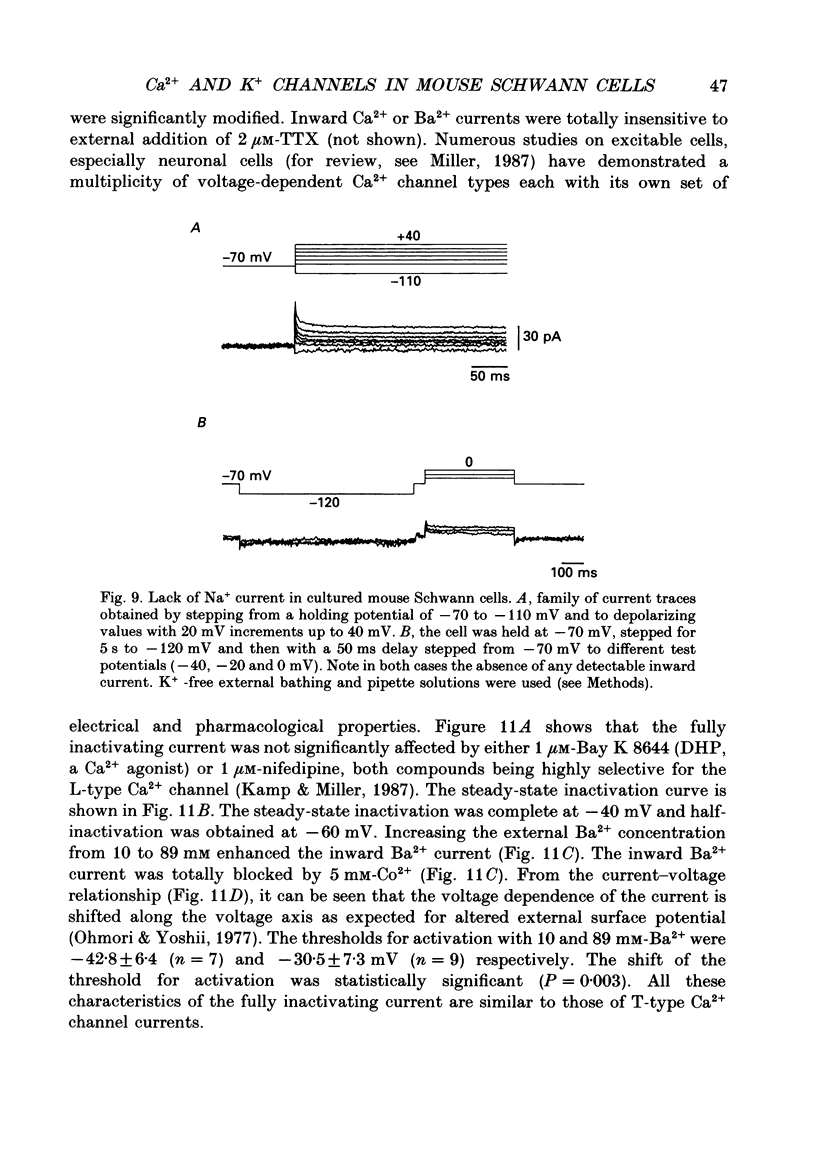
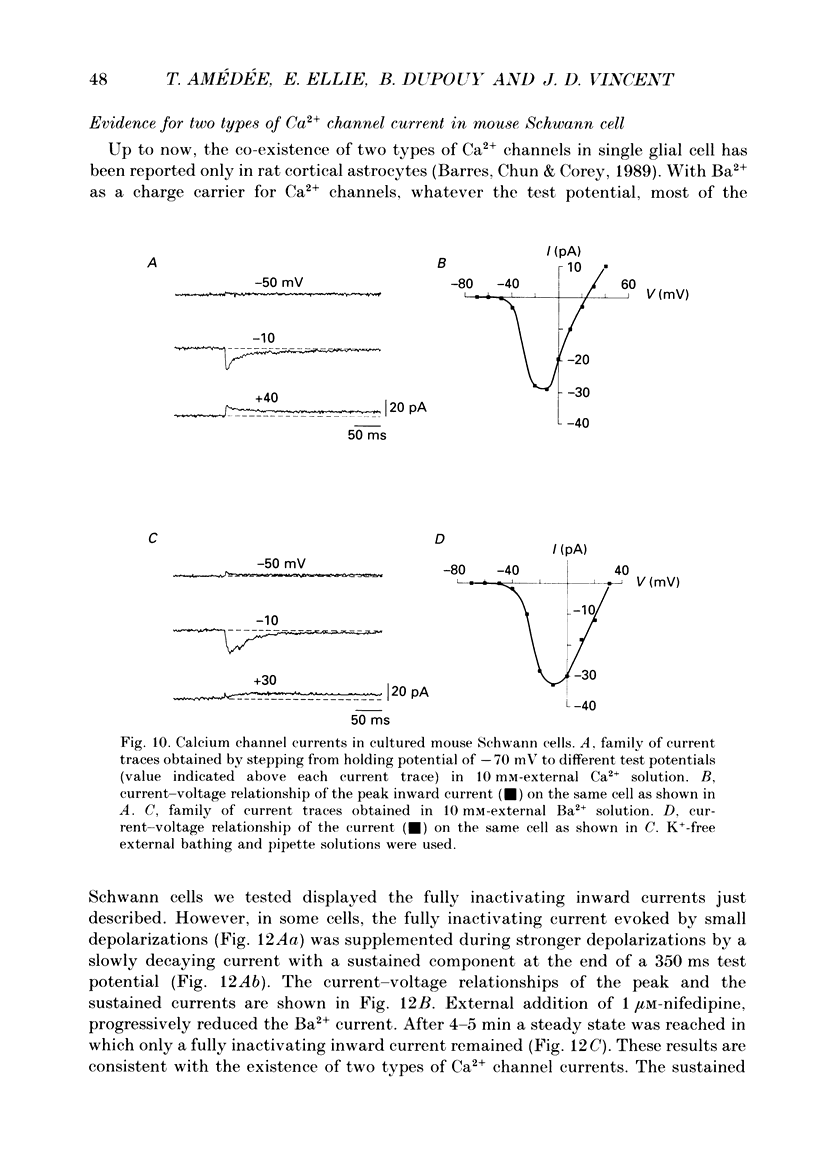
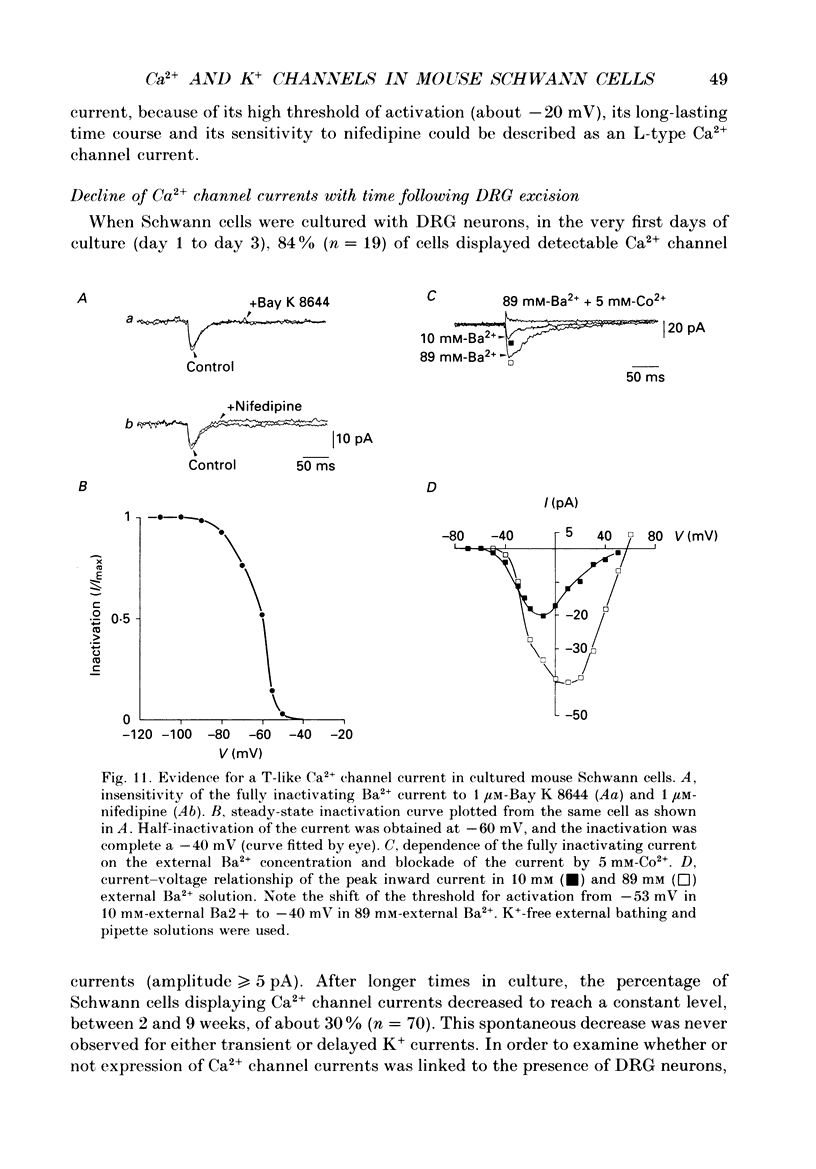
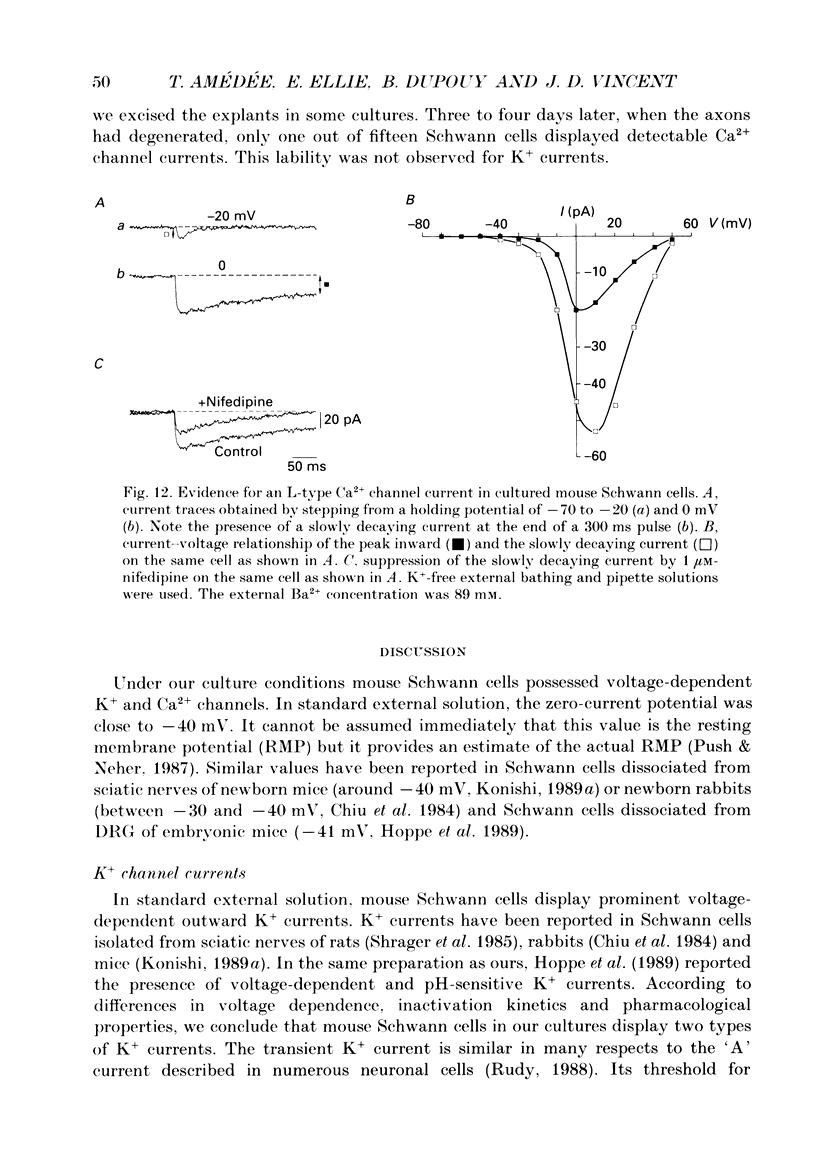
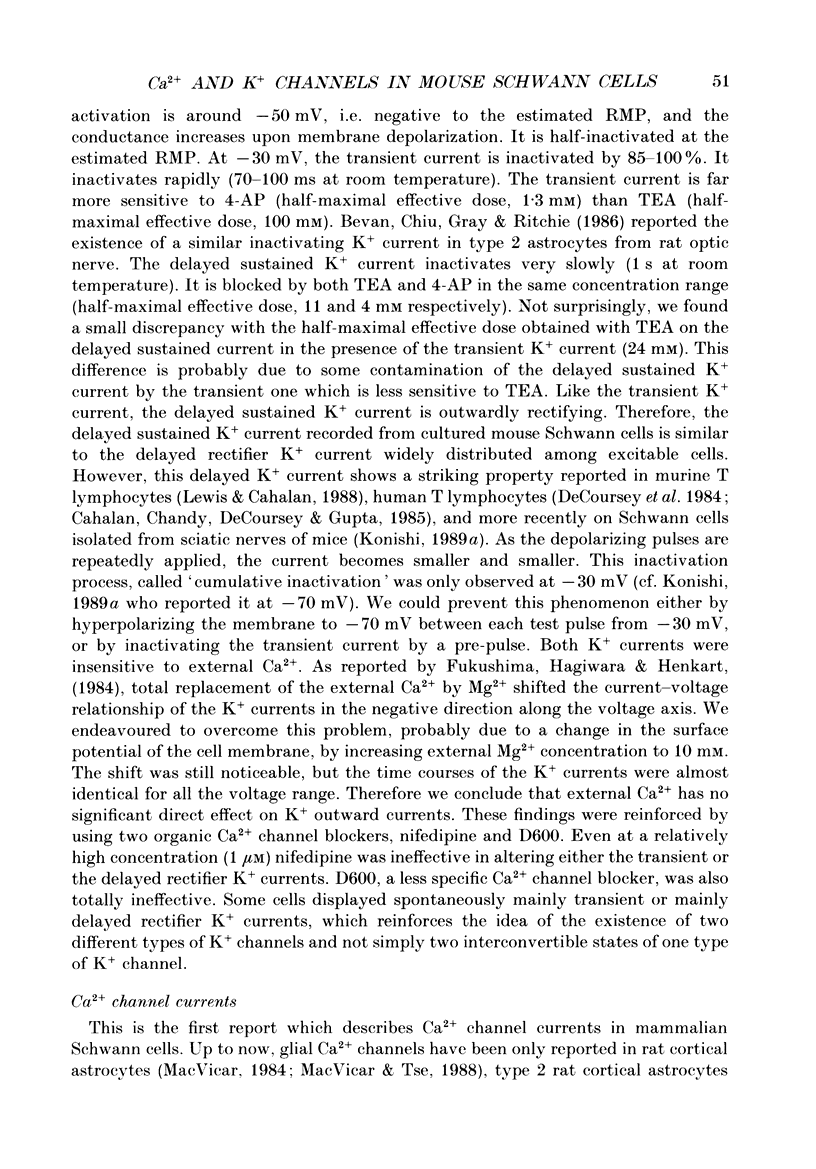
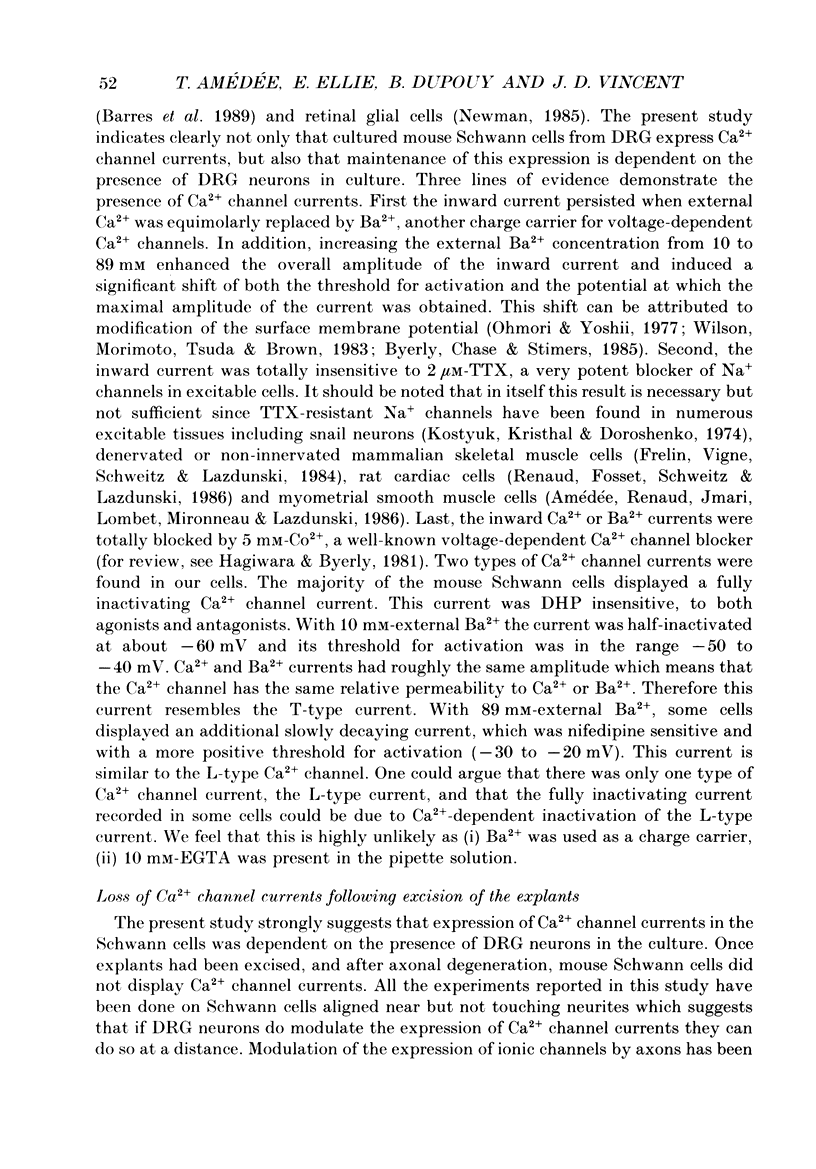
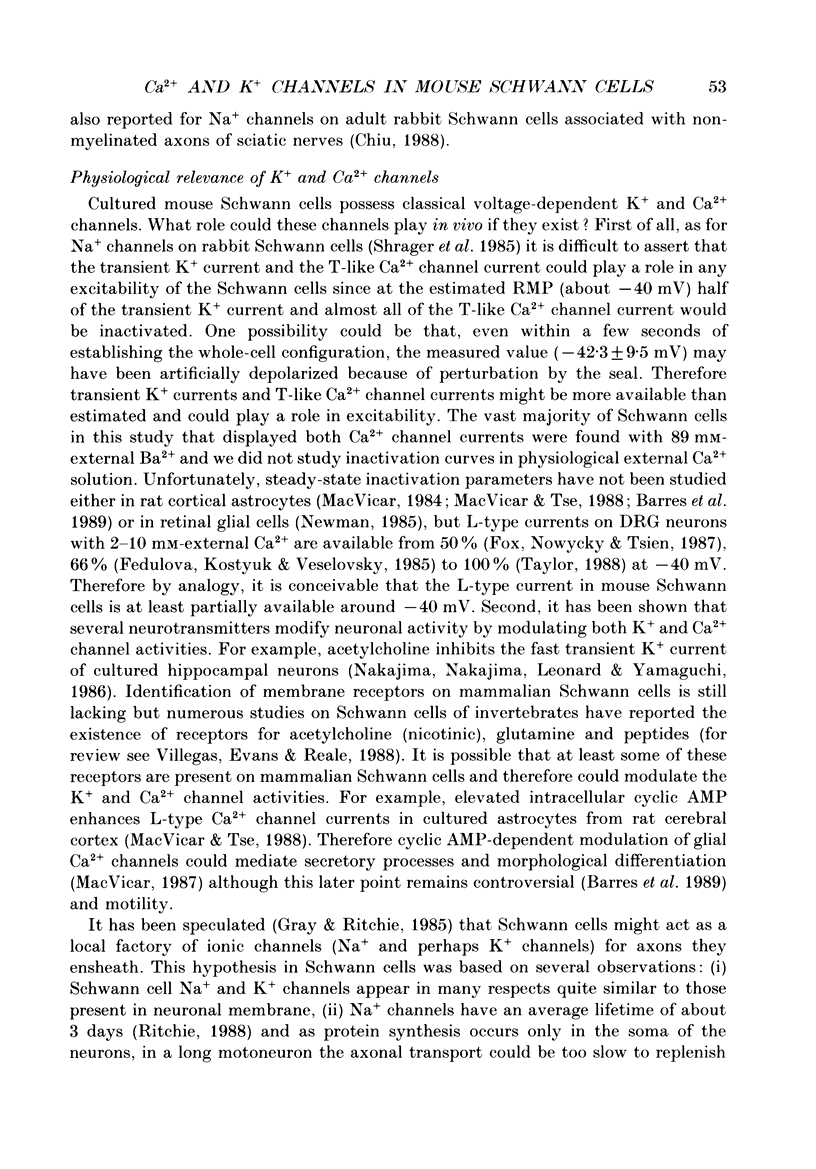
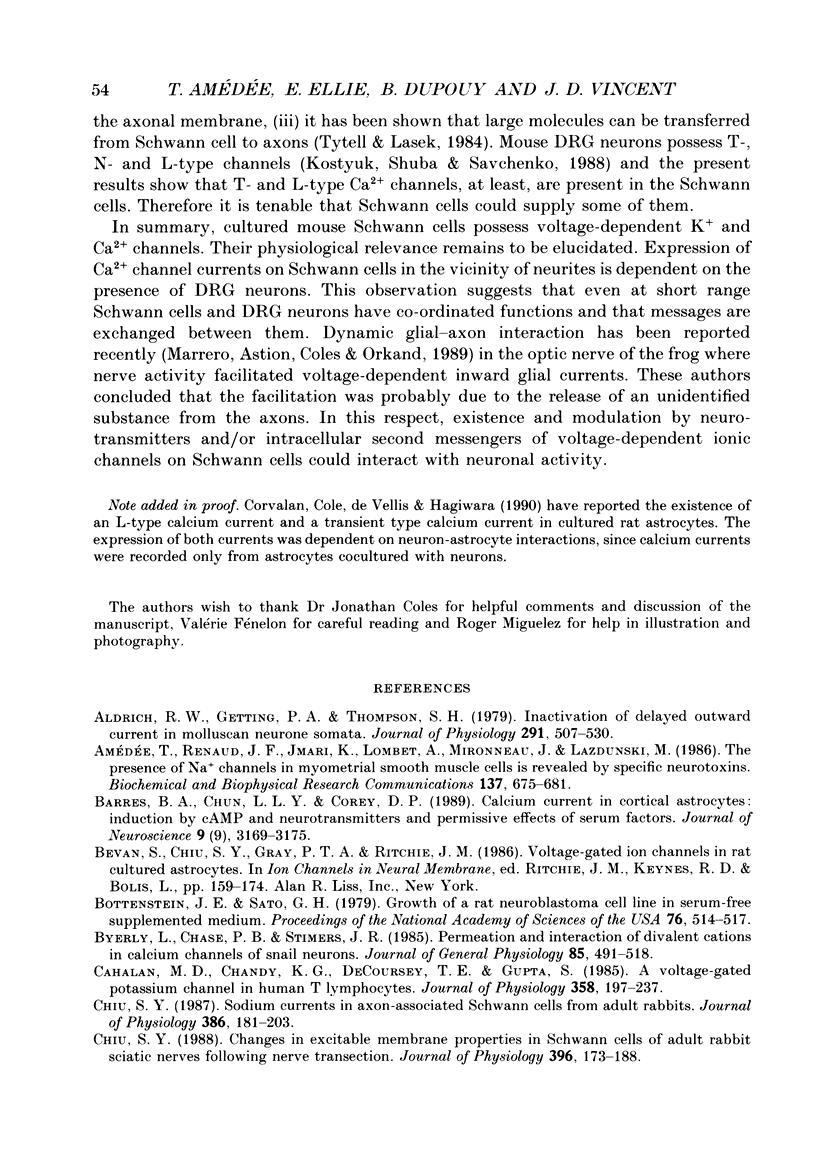
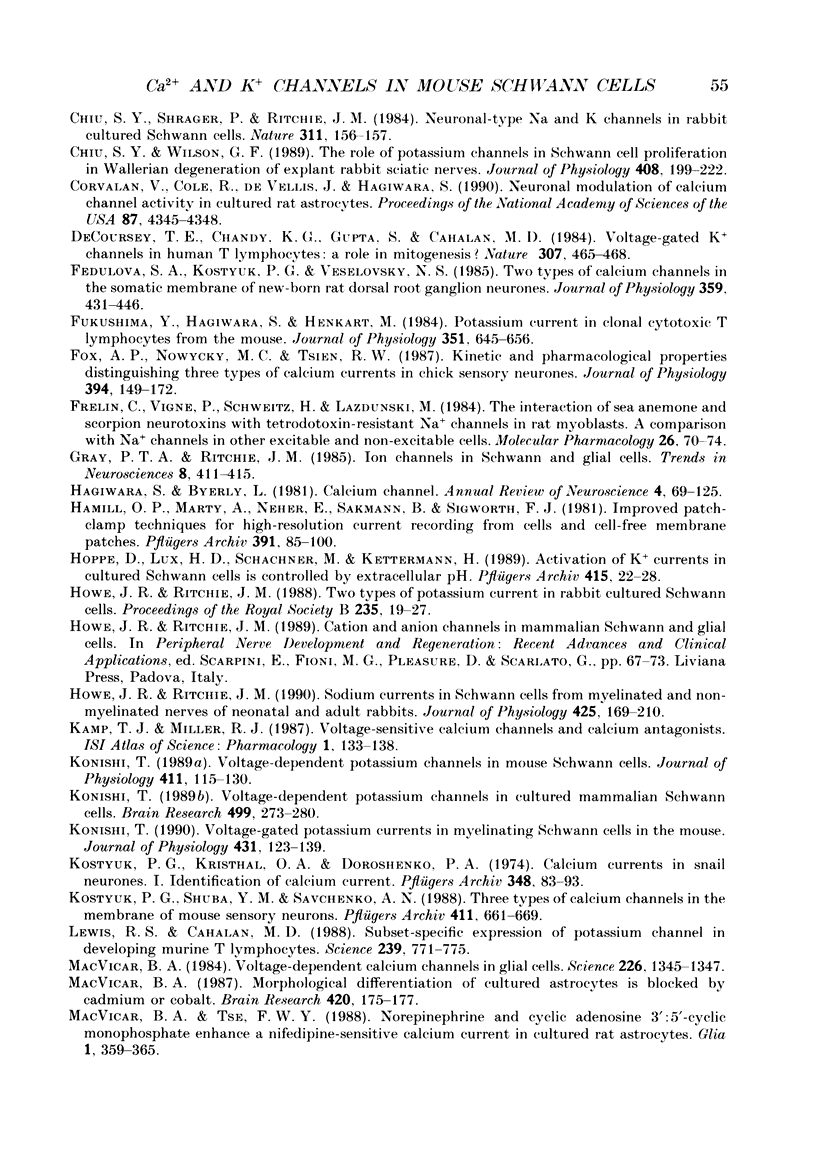
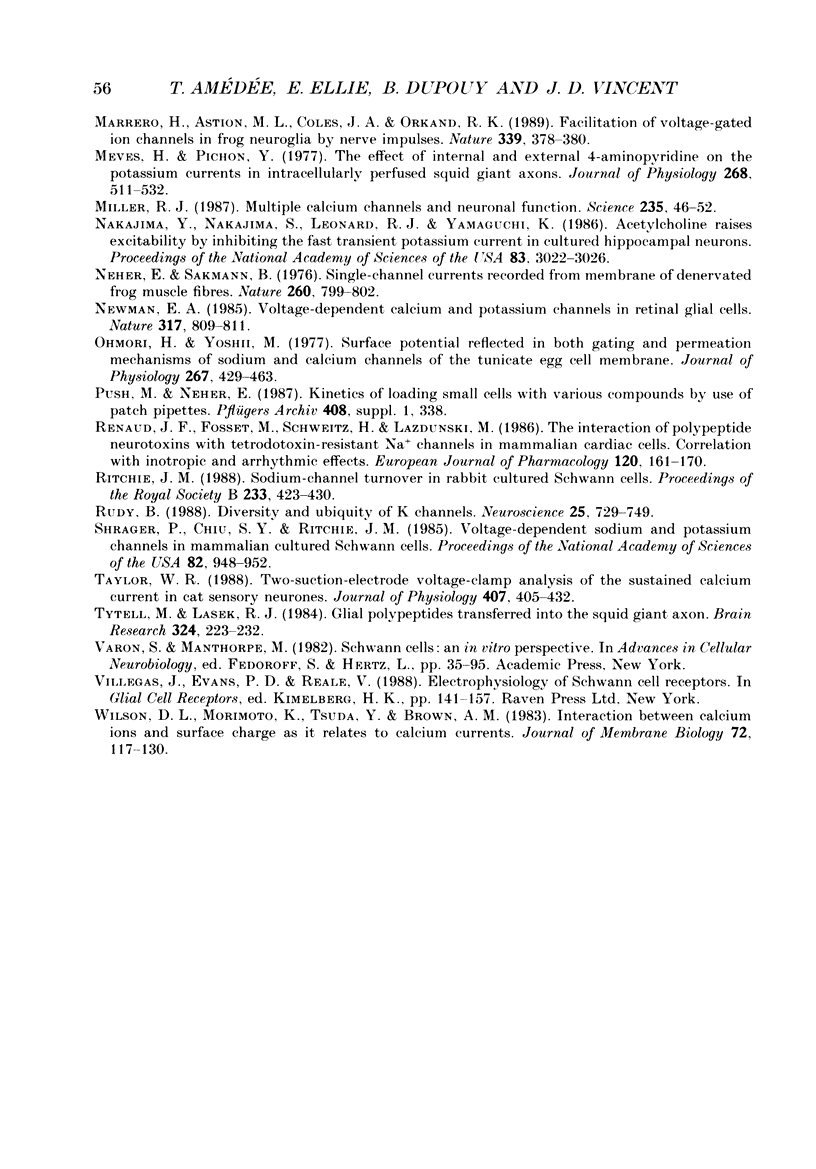
Images in this article
Selected References
These references are in PubMed. This may not be the complete list of references from this article.
- Aldrich R. W., Jr, Getting P. A., Thompson S. H. Inactivation of delayed outward current in molluscan neurone somata. J Physiol. 1979 Jun;291:507–530. doi: 10.1113/jphysiol.1979.sp012828. [DOI] [PMC free article] [PubMed] [Google Scholar]
- Amédée T., Renaud J. F., Jmari K., Lombet A., Mironneau J., Lazdunski M. The presence of Na+ channels in myometrial smooth muscle cells is revealed by specific neurotoxins. Biochem Biophys Res Commun. 1986 Jun 13;137(2):675–681. doi: 10.1016/0006-291x(86)91131-9. [DOI] [PubMed] [Google Scholar]
- Barres B. A., Chun L. L., Corey D. P. Calcium current in cortical astrocytes: induction by cAMP and neurotransmitters and permissive effect of serum factors. J Neurosci. 1989 Sep;9(9):3169–3175. doi: 10.1523/JNEUROSCI.09-09-03169.1989. [DOI] [PMC free article] [PubMed] [Google Scholar]
- Bottenstein J. E., Sato G. H. Growth of a rat neuroblastoma cell line in serum-free supplemented medium. Proc Natl Acad Sci U S A. 1979 Jan;76(1):514–517. doi: 10.1073/pnas.76.1.514. [DOI] [PMC free article] [PubMed] [Google Scholar]
- Byerly L., Chase P. B., Stimers J. R. Permeation and interaction of divalent cations in calcium channels of snail neurons. J Gen Physiol. 1985 Apr;85(4):491–518. doi: 10.1085/jgp.85.4.491. [DOI] [PMC free article] [PubMed] [Google Scholar]
- Cahalan M. D., Chandy K. G., DeCoursey T. E., Gupta S. A voltage-gated potassium channel in human T lymphocytes. J Physiol. 1985 Jan;358:197–237. doi: 10.1113/jphysiol.1985.sp015548. [DOI] [PMC free article] [PubMed] [Google Scholar]
- Chiu S. Y. Changes in excitable membrane properties in Schwann cells of adult rabbit sciatic nerves following nerve transection. J Physiol. 1988 Feb;396:173–188. doi: 10.1113/jphysiol.1988.sp016957. [DOI] [PMC free article] [PubMed] [Google Scholar]
- Chiu S. Y., Schrager P., Ritchie J. M. Neuronal-type Na+ and K+ channels in rabbit cultured Schwann cells. Nature. 1984 Sep 13;311(5982):156–157. doi: 10.1038/311156a0. [DOI] [PubMed] [Google Scholar]
- Chiu S. Y. Sodium currents in axon-associated Schwann cells from adult rabbits. J Physiol. 1987 May;386:181–203. doi: 10.1113/jphysiol.1987.sp016529. [DOI] [PMC free article] [PubMed] [Google Scholar]
- Chiu S. Y., Wilson G. F. The role of potassium channels in Schwann cell proliferation in Wallerian degeneration of explant rabbit sciatic nerves. J Physiol. 1989 Jan;408:199–222. doi: 10.1113/jphysiol.1989.sp017455. [DOI] [PMC free article] [PubMed] [Google Scholar]
- Corvalan V., Cole R., de Vellis J., Hagiwara S. Neuronal modulation of calcium channel activity in cultured rat astrocytes. Proc Natl Acad Sci U S A. 1990 Jun;87(11):4345–4348. doi: 10.1073/pnas.87.11.4345. [DOI] [PMC free article] [PubMed] [Google Scholar]
- DeCoursey T. E., Chandy K. G., Gupta S., Cahalan M. D. Voltage-gated K+ channels in human T lymphocytes: a role in mitogenesis? Nature. 1984 Feb 2;307(5950):465–468. doi: 10.1038/307465a0. [DOI] [PubMed] [Google Scholar]
- Fedulova S. A., Kostyuk P. G., Veselovsky N. S. Two types of calcium channels in the somatic membrane of new-born rat dorsal root ganglion neurones. J Physiol. 1985 Feb;359:431–446. doi: 10.1113/jphysiol.1985.sp015594. [DOI] [PMC free article] [PubMed] [Google Scholar]
- Fox A. P., Nowycky M. C., Tsien R. W. Kinetic and pharmacological properties distinguishing three types of calcium currents in chick sensory neurones. J Physiol. 1987 Dec;394:149–172. doi: 10.1113/jphysiol.1987.sp016864. [DOI] [PMC free article] [PubMed] [Google Scholar]
- Frelin C., Vigne P., Schweitz H., Lazdunski M. The interaction of sea anemone and scorpion neurotoxins with tetrodotoxin-resistant Na+ channels in rat myoblasts. A comparison with Na+ channels in other excitable and non-excitable cells. Mol Pharmacol. 1984 Jul;26(1):70–74. [PubMed] [Google Scholar]
- Fukushima Y., Hagiwara S., Henkart M. Potassium current in clonal cytotoxic T lymphocytes from the mouse. J Physiol. 1984 Jun;351:645–656. doi: 10.1113/jphysiol.1984.sp015268. [DOI] [PMC free article] [PubMed] [Google Scholar]
- Hagiwara S., Byerly L. Calcium channel. Annu Rev Neurosci. 1981;4:69–125. doi: 10.1146/annurev.ne.04.030181.000441. [DOI] [PubMed] [Google Scholar]
- Hamill O. P., Marty A., Neher E., Sakmann B., Sigworth F. J. Improved patch-clamp techniques for high-resolution current recording from cells and cell-free membrane patches. Pflugers Arch. 1981 Aug;391(2):85–100. doi: 10.1007/BF00656997. [DOI] [PubMed] [Google Scholar]
- Hoppe D., Lux H. D., Schachner M., Kettenmann H. Activation of K+ currents in cultured Schwann cells is controlled by extracellular pH. Pflugers Arch. 1989 Oct;415(1):22–28. doi: 10.1007/BF00373137. [DOI] [PubMed] [Google Scholar]
- Howe J. R., Ritchie J. M. Sodium currents in Schwann cells from myelinated and non-myelinated nerves of neonatal and adult rabbits. J Physiol. 1990 Jun;425:169–210. doi: 10.1113/jphysiol.1990.sp018098. [DOI] [PMC free article] [PubMed] [Google Scholar]
- Howe J. R., Ritchie J. M. Two types of potassium current in rabbit cultured Schwann cells. Proc R Soc Lond B Biol Sci. 1988 Oct 22;235(1278):19–27. doi: 10.1098/rspb.1988.0061. [DOI] [PubMed] [Google Scholar]
- Konishi T. Voltage-dependent potassium channels in cultured mammalian Schwann cells. Brain Res. 1989 Oct 16;499(2):273–280. doi: 10.1016/0006-8993(89)90775-0. [DOI] [PubMed] [Google Scholar]
- Konishi T. Voltage-dependent potassium channels in mouse Schwann cells. J Physiol. 1989 Apr;411:115–130. doi: 10.1113/jphysiol.1989.sp017564. [DOI] [PMC free article] [PubMed] [Google Scholar]
- Konishi T. Voltage-gated potassium currents in myelinating Schwann cells in the mouse. J Physiol. 1990 Dec;431:123–139. doi: 10.1113/jphysiol.1990.sp018323. [DOI] [PMC free article] [PubMed] [Google Scholar]
- Kostyuk P. G., Krishtal O. A., Doroshenko P. A. Calcium currents in snail neurones. I. Identification of calcium current. Pflugers Arch. 1974 Apr 11;348(2):83–93. doi: 10.1007/BF00586471. [DOI] [PubMed] [Google Scholar]
- Kostyuk P. G., Shuba YaM, Savchenko A. N. Three types of calcium channels in the membrane of mouse sensory neurons. Pflugers Arch. 1988 Jun;411(6):661–669. doi: 10.1007/BF00580863. [DOI] [PubMed] [Google Scholar]
- Lewis R. S., Cahalan M. D. Subset-specific expression of potassium channels in developing murine T lymphocytes. Science. 1988 Feb 12;239(4841 Pt 1):771–775. doi: 10.1126/science.2448877. [DOI] [PubMed] [Google Scholar]
- MacVicar B. A. Morphological differentiation of cultured astrocytes is blocked by cadmium or cobalt. Brain Res. 1987 Sep 8;420(1):175–177. doi: 10.1016/0006-8993(87)90256-3. [DOI] [PubMed] [Google Scholar]
- MacVicar B. A., Tse F. W. Norepinephrine and cyclic adenosine 3':5'-cyclic monophosphate enhance a nifedipine-sensitive calcium current in cultured rat astrocytes. Glia. 1988;1(6):359–365. doi: 10.1002/glia.440010602. [DOI] [PubMed] [Google Scholar]
- MacVicar B. A. Voltage-dependent calcium channels in glial cells. Science. 1984 Dec 14;226(4680):1345–1347. doi: 10.1126/science.6095454. [DOI] [PubMed] [Google Scholar]
- Marrero H., Astion M. L., Coles J. A., Orkand R. K. Facilitation of voltage-gated ion channels in frog neuroglia by nerve impulses. Nature. 1989 Jun 1;339(6223):378–380. doi: 10.1038/339378a0. [DOI] [PubMed] [Google Scholar]
- Meves H., Pichon Y. The effect of internal and external 4-aminopyridine on the potassium currents in intracellularly perfused squid giant axons. J Physiol. 1977 Jun;268(2):511–532. doi: 10.1113/jphysiol.1977.sp011869. [DOI] [PMC free article] [PubMed] [Google Scholar]
- Miller R. J. Multiple calcium channels and neuronal function. Science. 1987 Jan 2;235(4784):46–52. doi: 10.1126/science.2432656. [DOI] [PubMed] [Google Scholar]
- Nakajima Y., Nakajima S., Leonard R. J., Yamaguchi K. Acetylcholine raises excitability by inhibiting the fast transient potassium current in cultured hippocampal neurons. Proc Natl Acad Sci U S A. 1986 May;83(9):3022–3026. doi: 10.1073/pnas.83.9.3022. [DOI] [PMC free article] [PubMed] [Google Scholar]
- Neher E., Sakmann B. Single-channel currents recorded from membrane of denervated frog muscle fibres. Nature. 1976 Apr 29;260(5554):799–802. doi: 10.1038/260799a0. [DOI] [PubMed] [Google Scholar]
- Newman E. A. Voltage-dependent calcium and potassium channels in retinal glial cells. 1985 Oct 31-Nov 6Nature. 317(6040):809–811. doi: 10.1038/317809a0. [DOI] [PMC free article] [PubMed] [Google Scholar]
- Ohmori H., Yoshii M. Surface potential reflected in both gating and permeation mechanisms of sodium and calcium channels of the tunicate egg cell membrane. J Physiol. 1977 May;267(2):429–463. doi: 10.1113/jphysiol.1977.sp011821. [DOI] [PMC free article] [PubMed] [Google Scholar]
- Renaud J. F., Fosset M., Schweitz H., Lazdunski M. The interaction of polypeptide neurotoxins with tetrodotoxin-resistant Na+ channels in mammalian cardiac cells. Correlation with inotropic and arrhythmic effects. Eur J Pharmacol. 1986 Jan 21;120(2):161–170. doi: 10.1016/0014-2999(86)90536-4. [DOI] [PubMed] [Google Scholar]
- Ritchie J. M. Sodium-channel turnover in rabbit cultured Schwann cells. Proc R Soc Lond B Biol Sci. 1988 May 23;233(1273):423–430. doi: 10.1098/rspb.1988.0031. [DOI] [PubMed] [Google Scholar]
- Rudy B. Diversity and ubiquity of K channels. Neuroscience. 1988 Jun;25(3):729–749. doi: 10.1016/0306-4522(88)90033-4. [DOI] [PubMed] [Google Scholar]
- Shrager P., Chiu S. Y., Ritchie J. M. Voltage-dependent sodium and potassium channels in mammalian cultured Schwann cells. Proc Natl Acad Sci U S A. 1985 Feb;82(3):948–952. doi: 10.1073/pnas.82.3.948. [DOI] [PMC free article] [PubMed] [Google Scholar]
- Tytell M., Lasek R. J. Glial polypeptides transferred into the squid giant axon. Brain Res. 1984 Dec 24;324(2):223–232. doi: 10.1016/0006-8993(84)90032-5. [DOI] [PubMed] [Google Scholar]
- Wilson D. L., Morimoto K., Tsuda Y., Brown A. M. Interaction between calcium ions and surface charge as it relates to calcium currents. J Membr Biol. 1983;72(1-2):117–130. doi: 10.1007/BF01870319. [DOI] [PubMed] [Google Scholar]