Abstract
1. Double-barrelled Cl(-)-sensitive microelectrodes have been used to measure the intracellular Cl- activity (aCli) and membrane potential (Em) in rat lumbrical muscles. The mean Cl- equilibrium potential (ECl), calculated from the measured aCli in sixty fibres, was 2.9 +/- 2.5 mV (S.D. of an observation) less negative than Em. The value of aCli was higher than would be expected for a passive distribution, by a mean 1.4 +/- 1.2 mM. The mean Em was -59.5 +/- 8.2 mV. 2. Removal of external Cl- (Cl-(o)) resulted in a rapid fall in aCli and a transient depolarization. aCli stabilized at an apparent level of 1.7 +/- 1.0 mM (n = 24) while Em became substantially more negative than in normal Krebs solution (mean, -80.1 +/- 12.4 mV). Readdition of Cl-(o) caused a rapid rise in aCli and transient hyperpolarization. ECl quickly became less negative than Em and both then fell in parallel towards the levels previously recorded in normal Krebs solution. 3. If lack of selectivity of the Cl(-)-sensitive ion exchanger and the intracellular presence of interfering anions, assumed to be responsible for the apparent aCli recorded in Cl(-)-depleted fibres, were also responsible for the apparently non-passive Cl- distribution recorded under normal conditions, the difference between the calculated ECl and Em would increase at more negative potentials. This was not observed over a range of Em values between -46 and -84 mV. 4. Inhibition of the Cl- permeability by application of 9-anthracene carboxylic acid (9-AC) resulted in an immediate rise in aCli and hyperpolarization. An aCli up to 40 mM higher, or eleven times higher, than that predicted by a passive distribution was recorded. Application of 9-AC after depletion of intracellular Cl- in Cl(-)-free solution had no effect on either the apparent aCli or Em. 5. It is concluded that Cl- ions are actively accumulated by the skeletal muscle fibre and that the Cl- distribution therefore normally exerts a depolarizing influence. 6. In the presence of 9-AC and nominal absence of CO2 and HCO3-, readdition of Cl-(o) to Cl(-)-depleted fibres resulted in a substantial rise in aCli and a small, maintained depolarization. This clear demonstration of active accumulation was used to investigate the mechanism responsible for inward transport of Cl- ions. 7. Neither application of CO2 and HCO3- nor application of DIDS (4,4'-diisothiocyanostilbene-2,2'-disulphonic acid) had any effect on the accumulation of Cl- ions. This suggests that Cl(-)-HCO3- exchange is not involved.(ABSTRACT TRUNCATED AT 400 WORDS)
Full text
PDF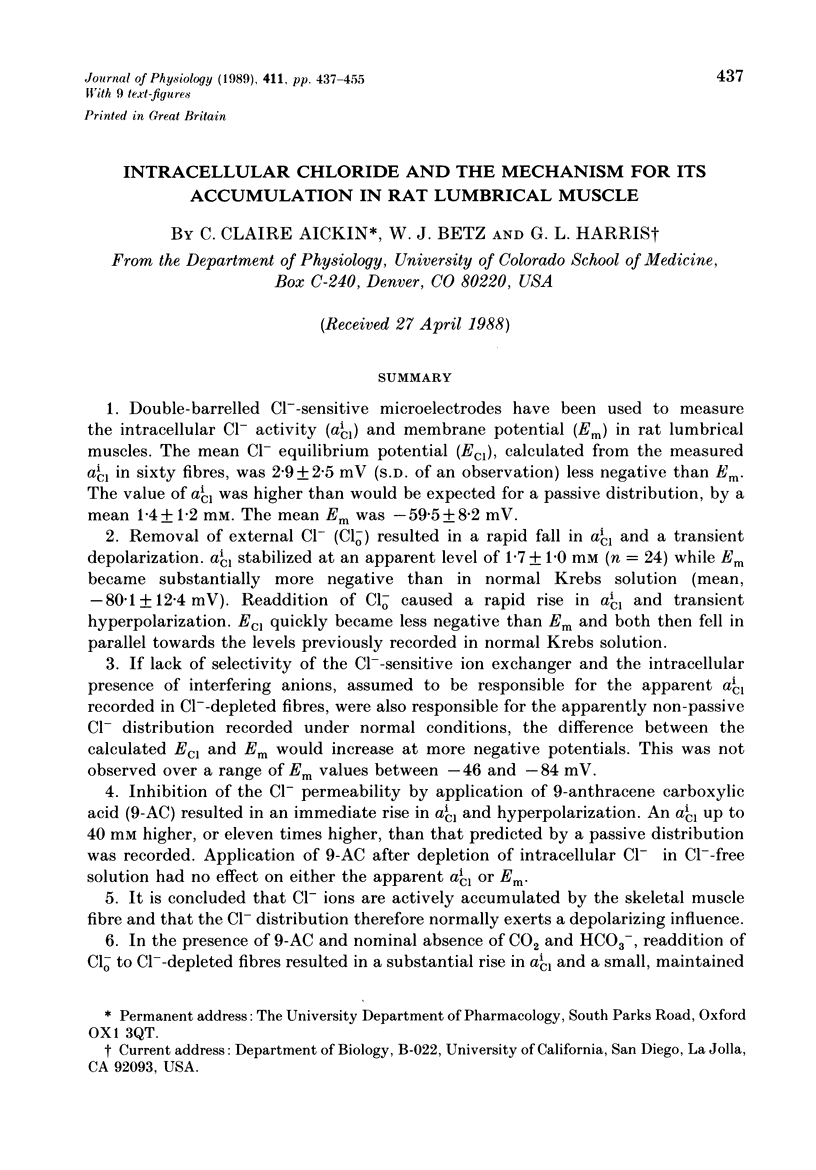
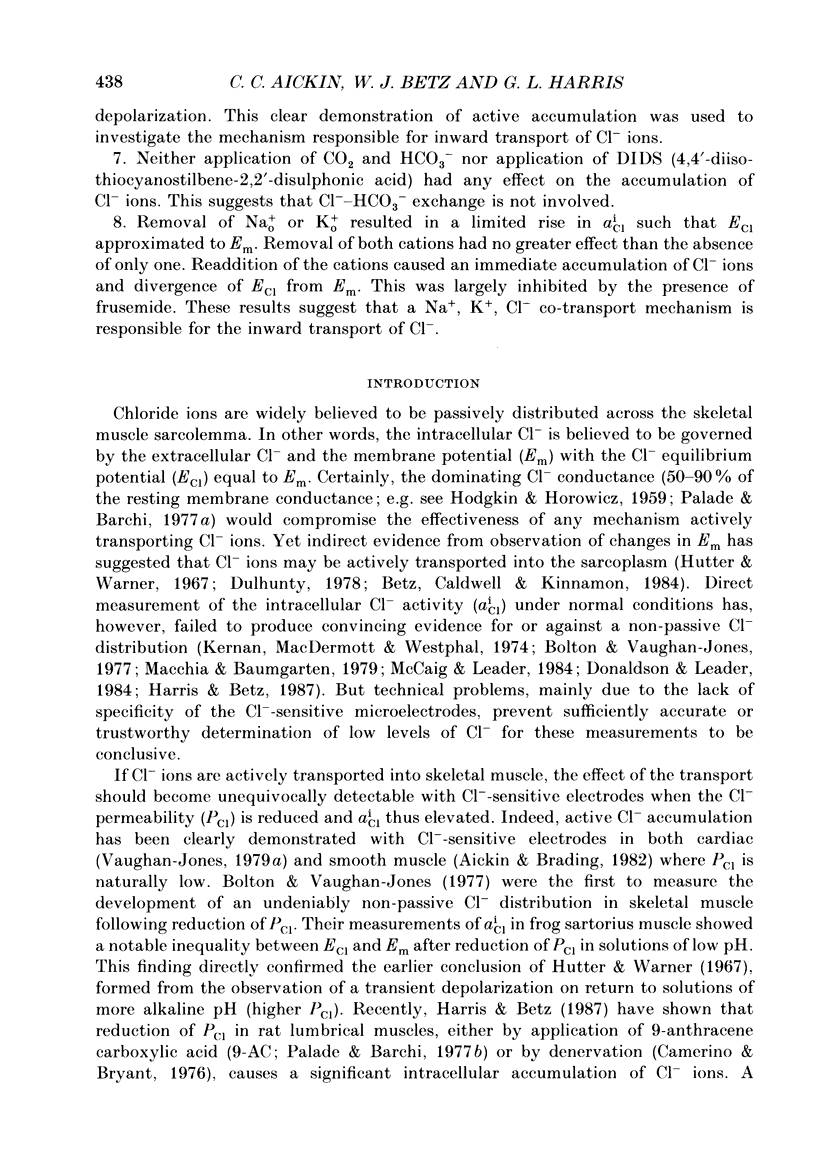
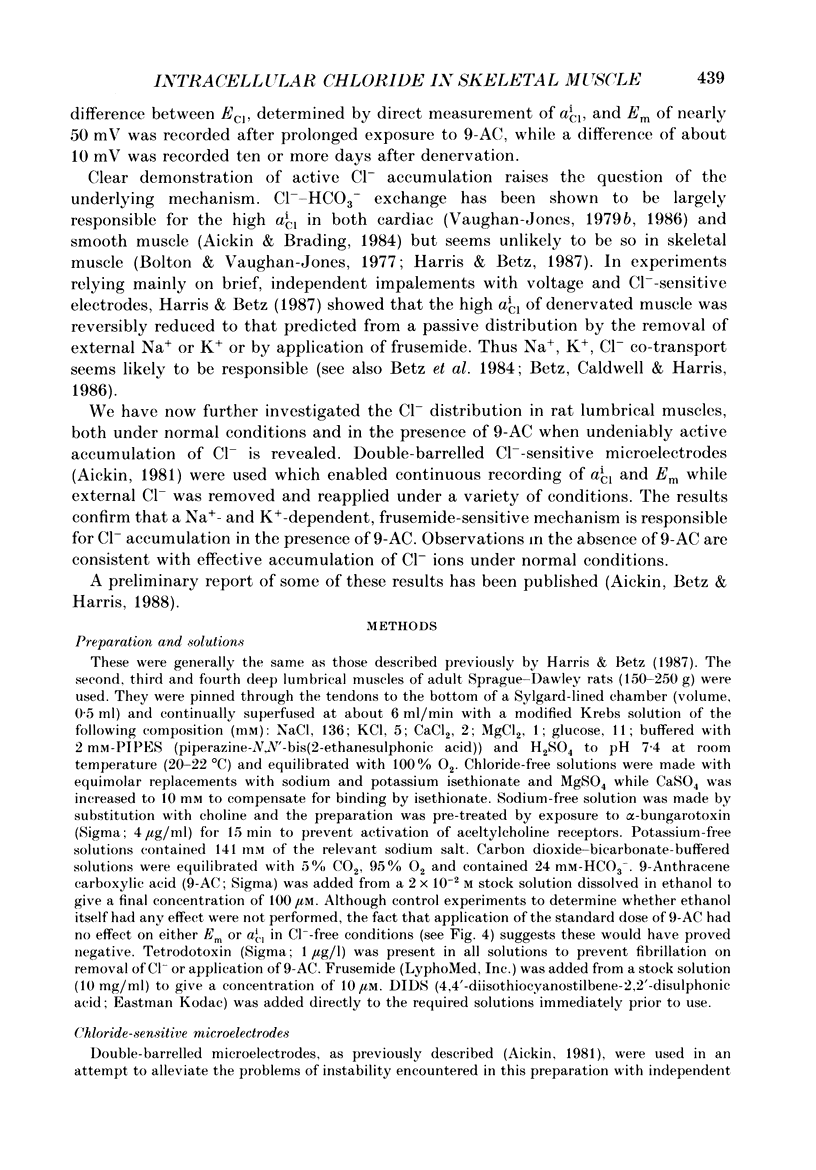
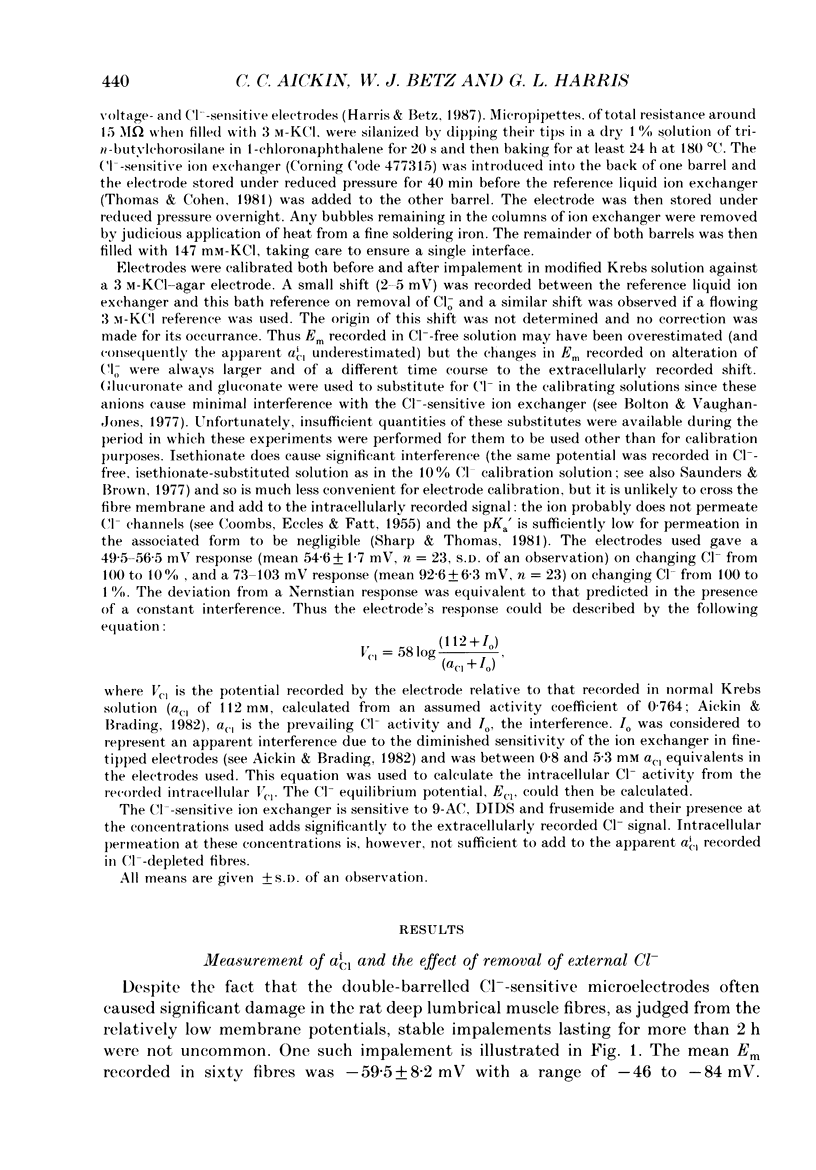
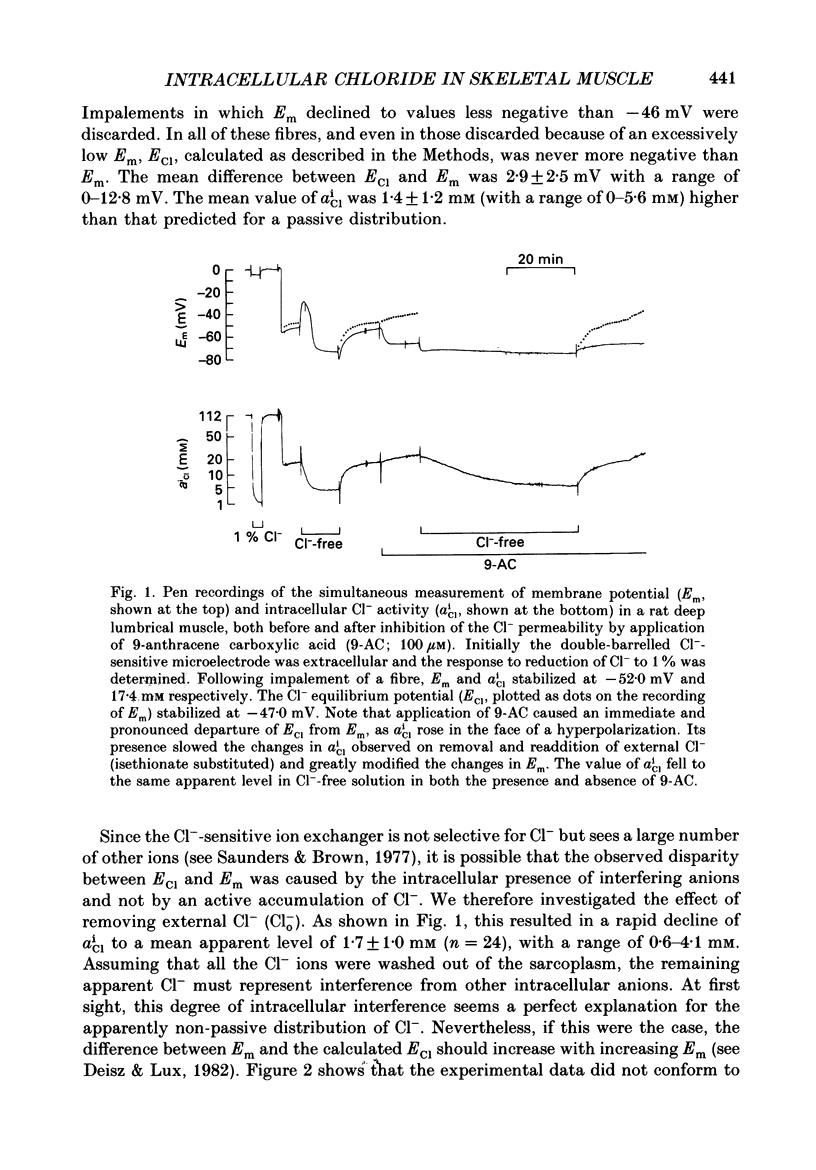
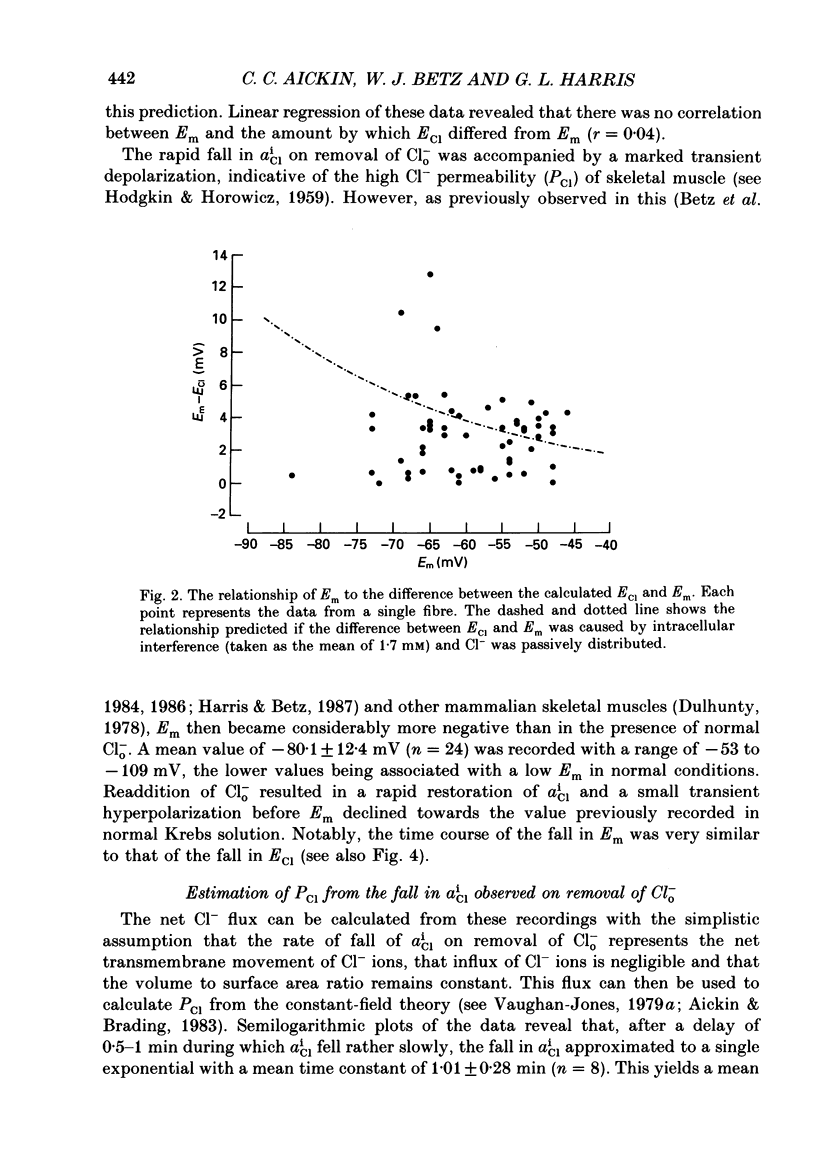
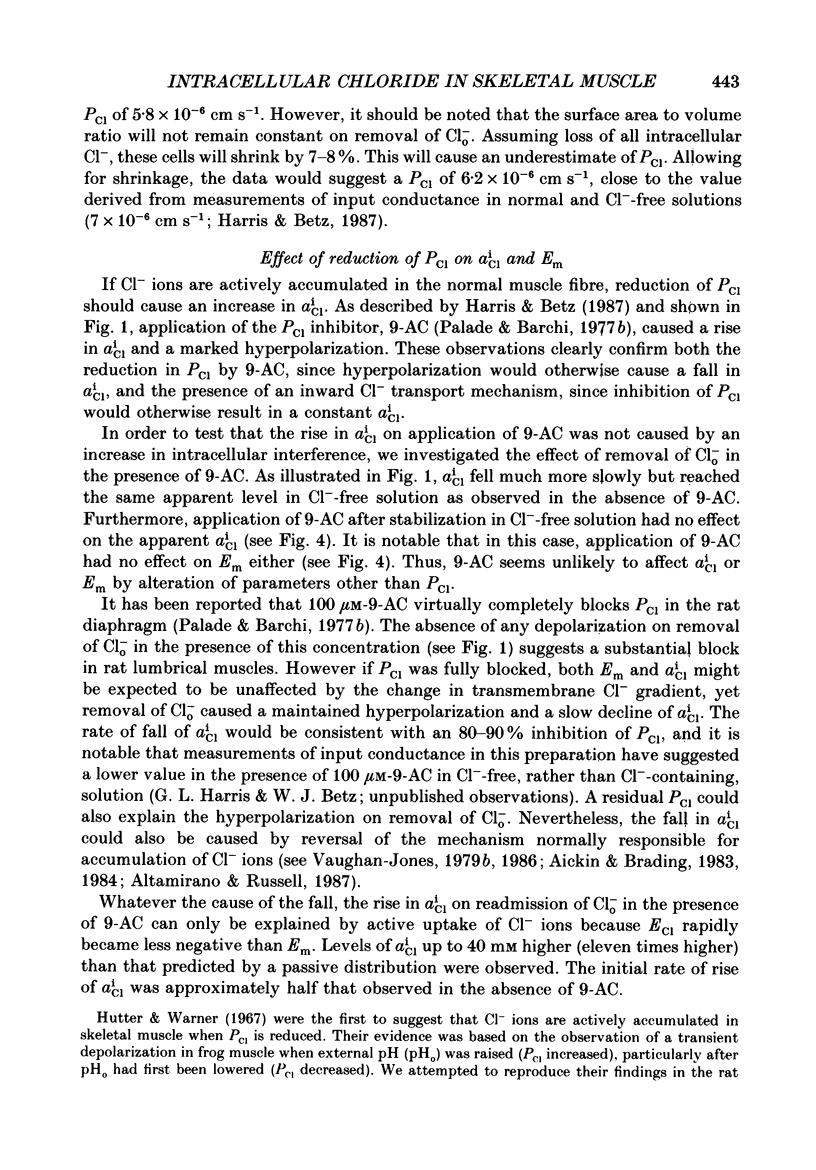
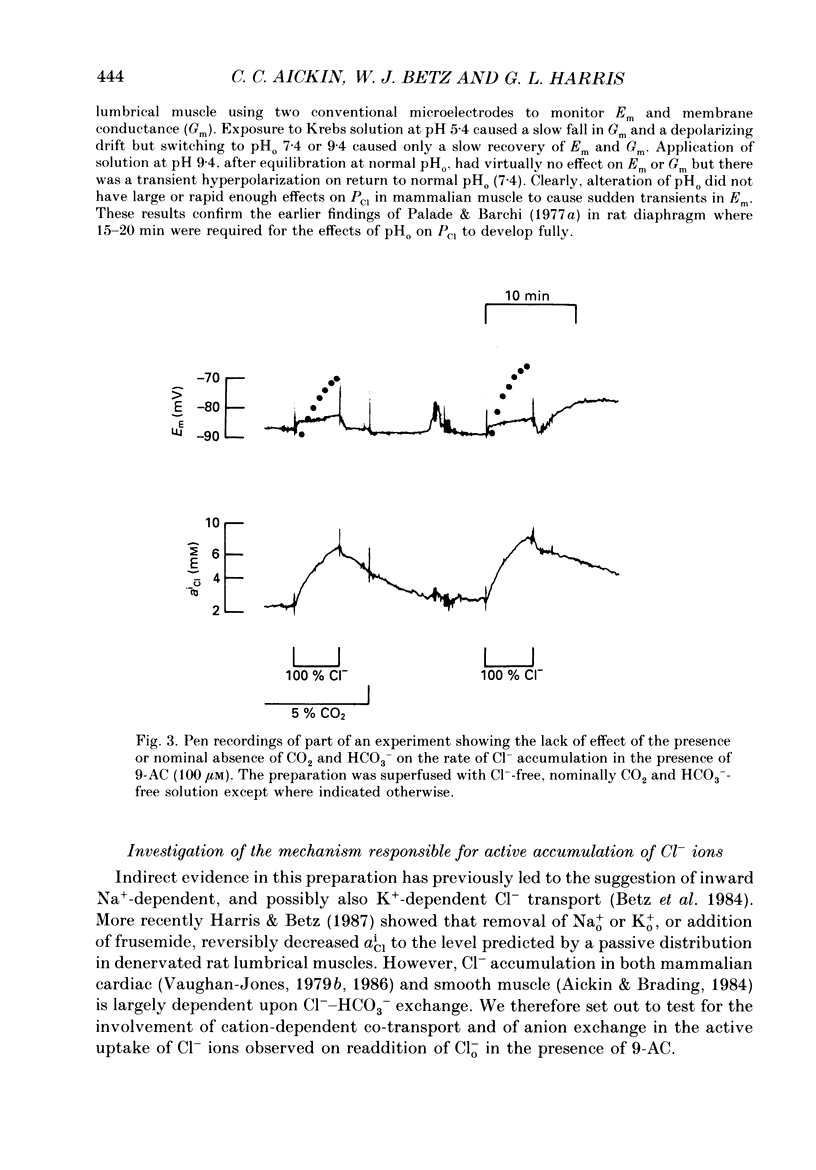
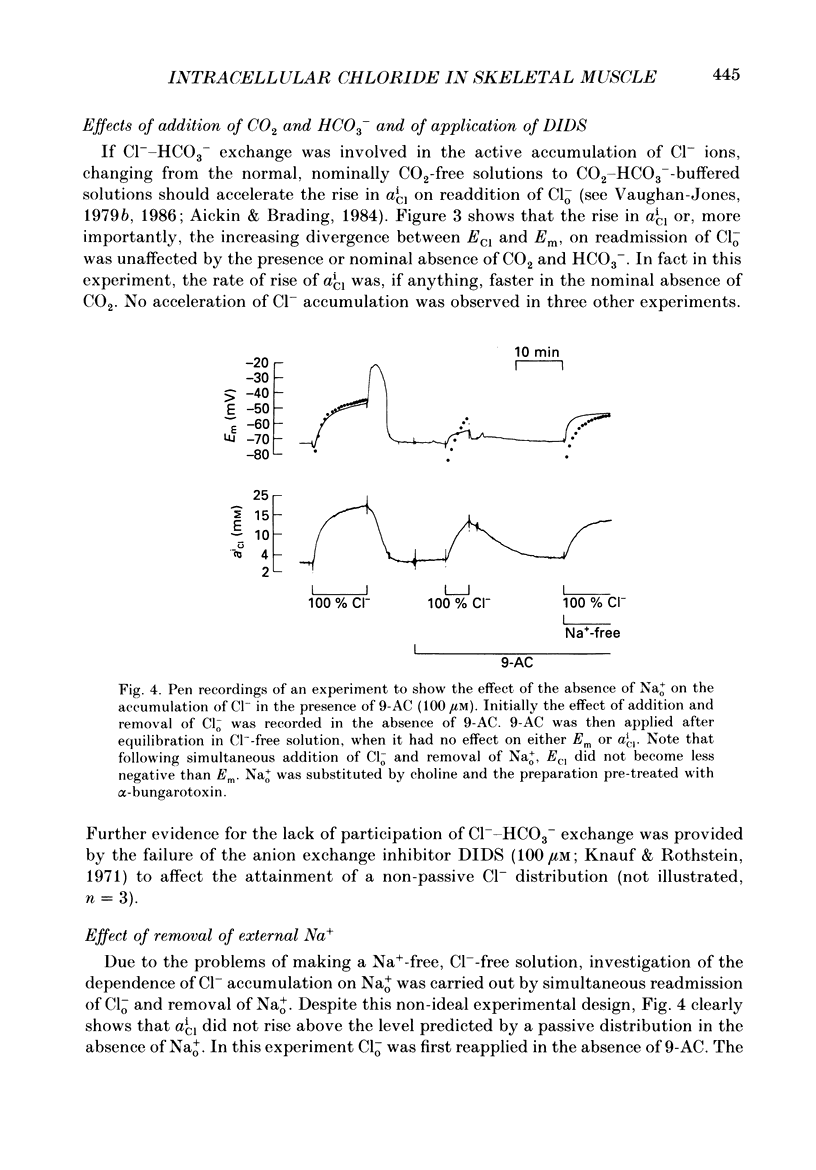
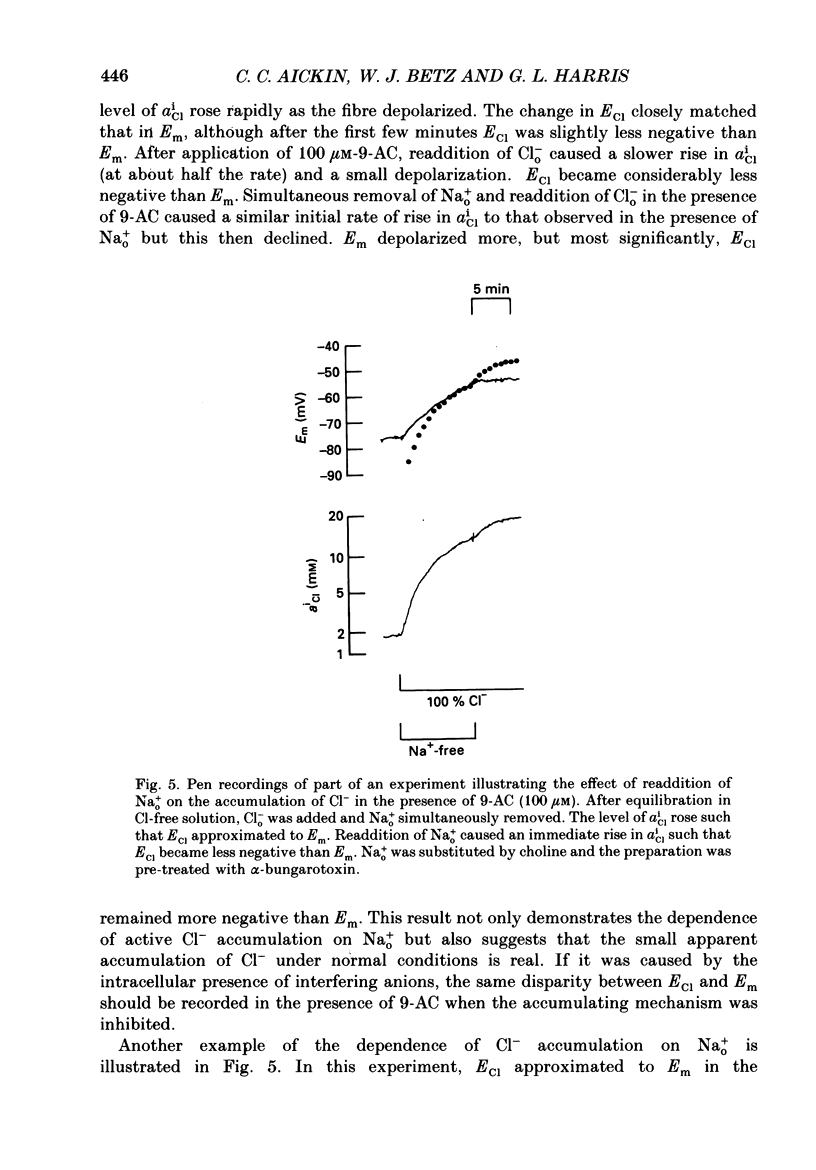
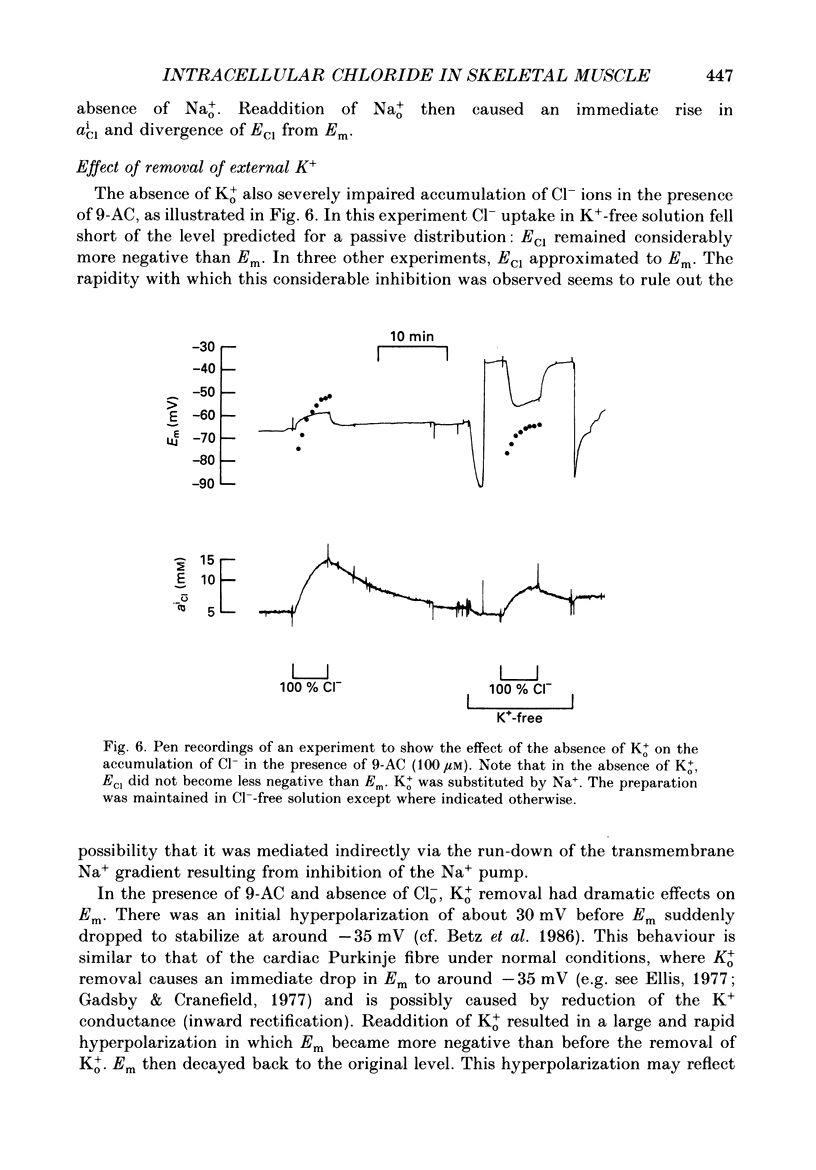
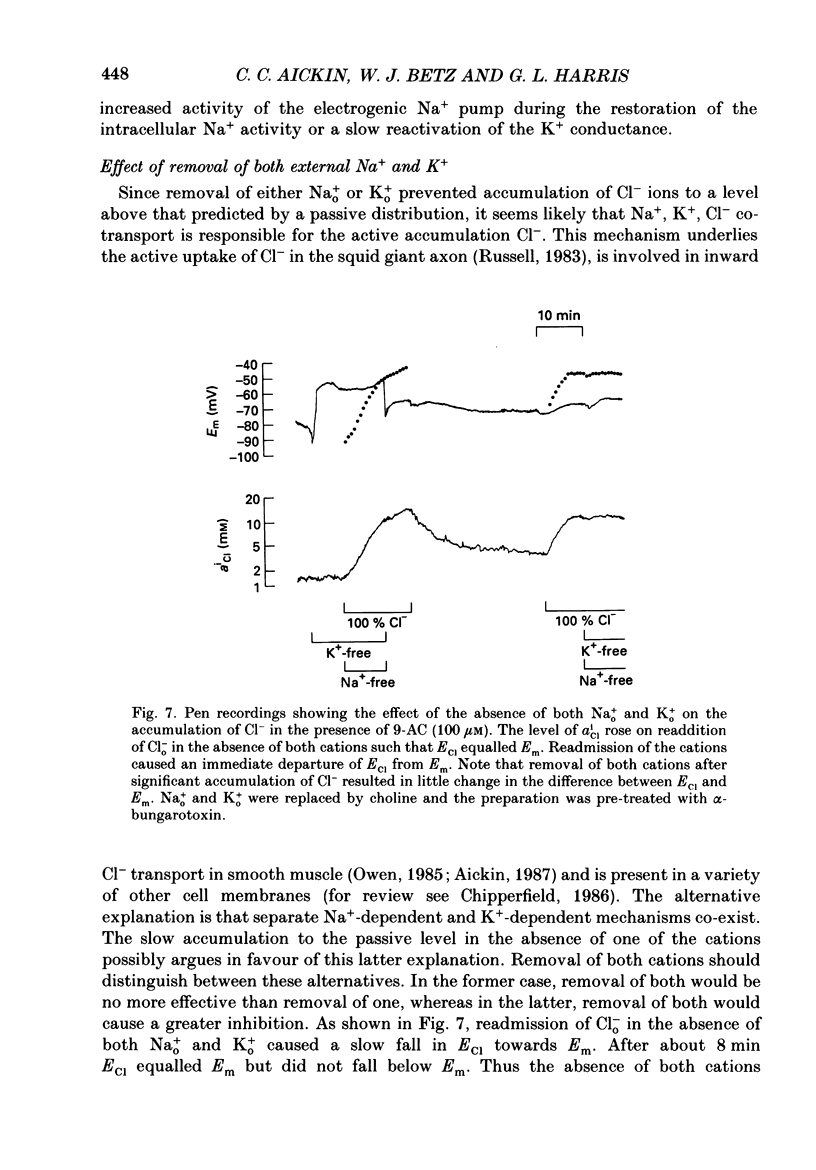
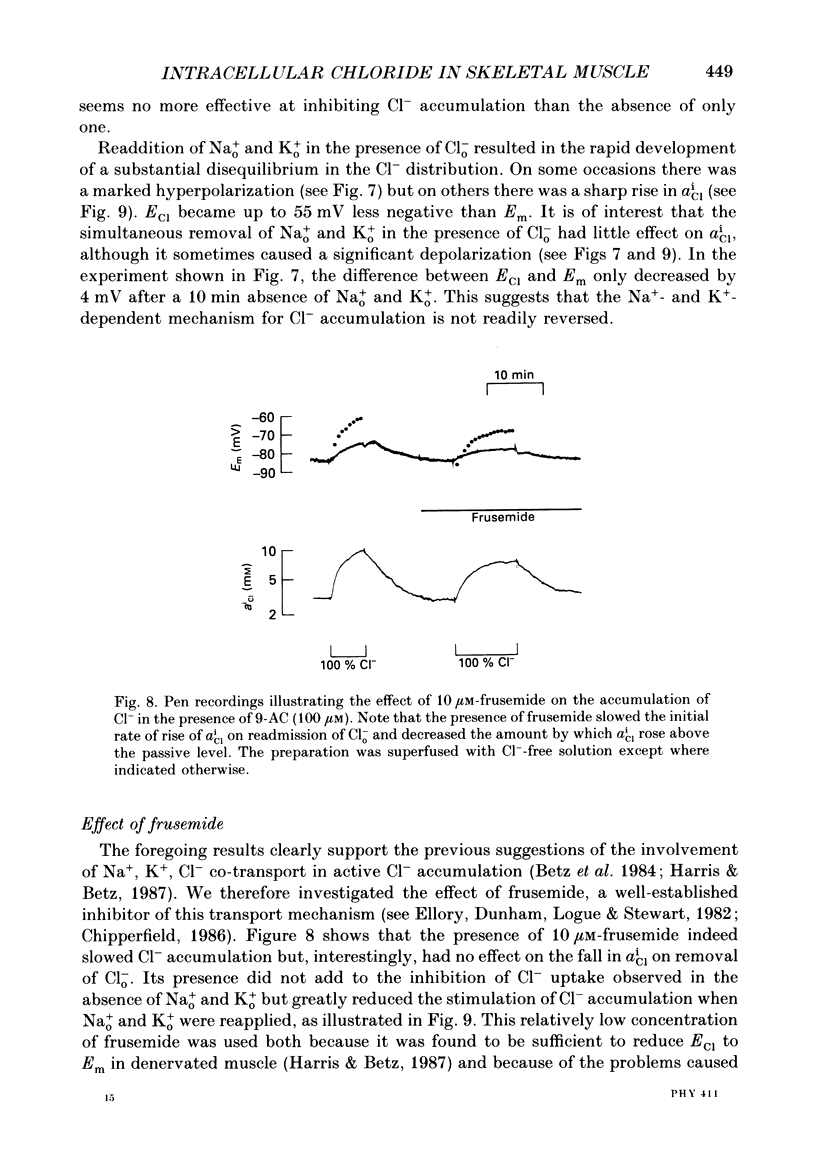
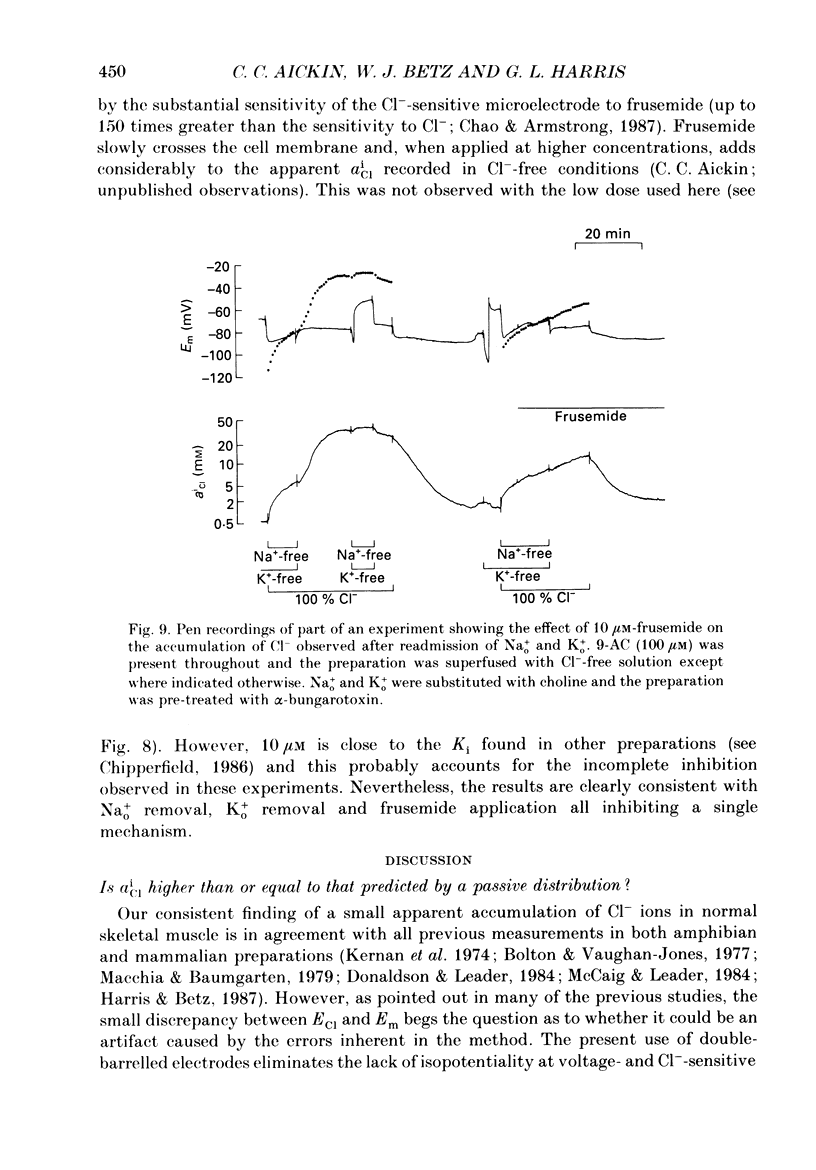
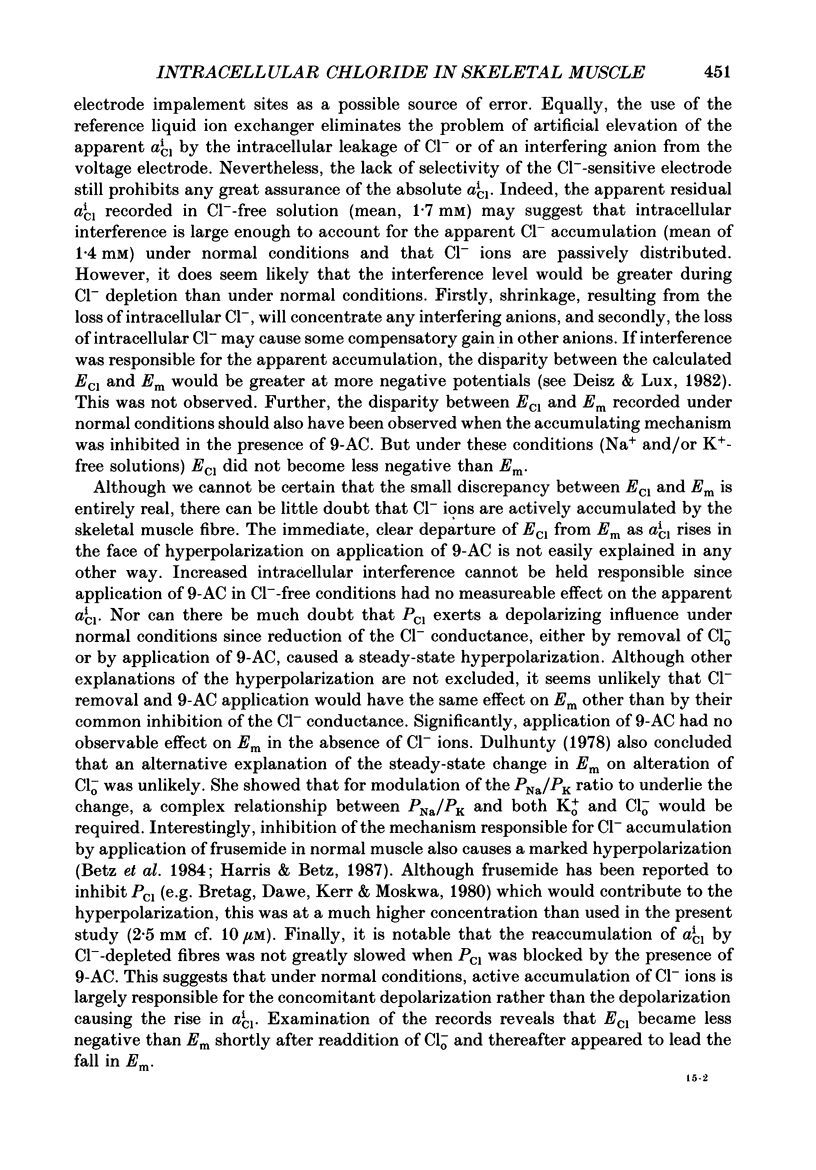
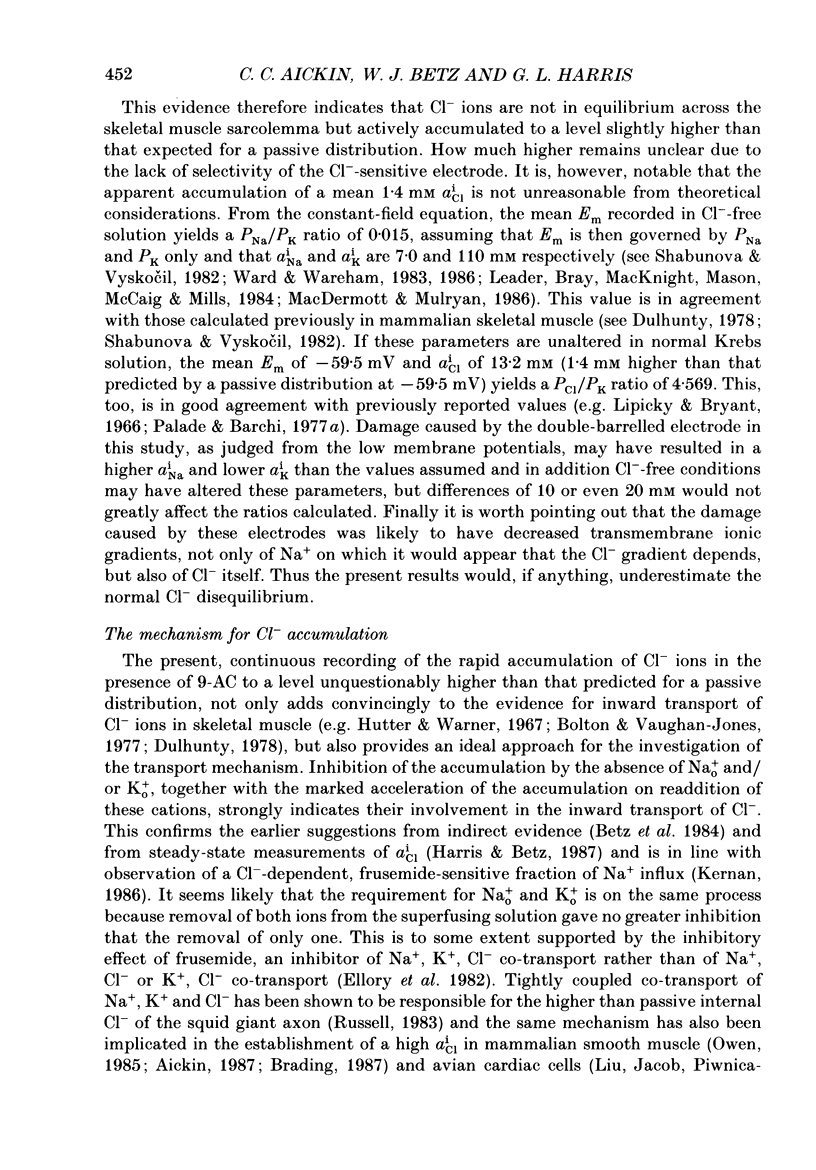
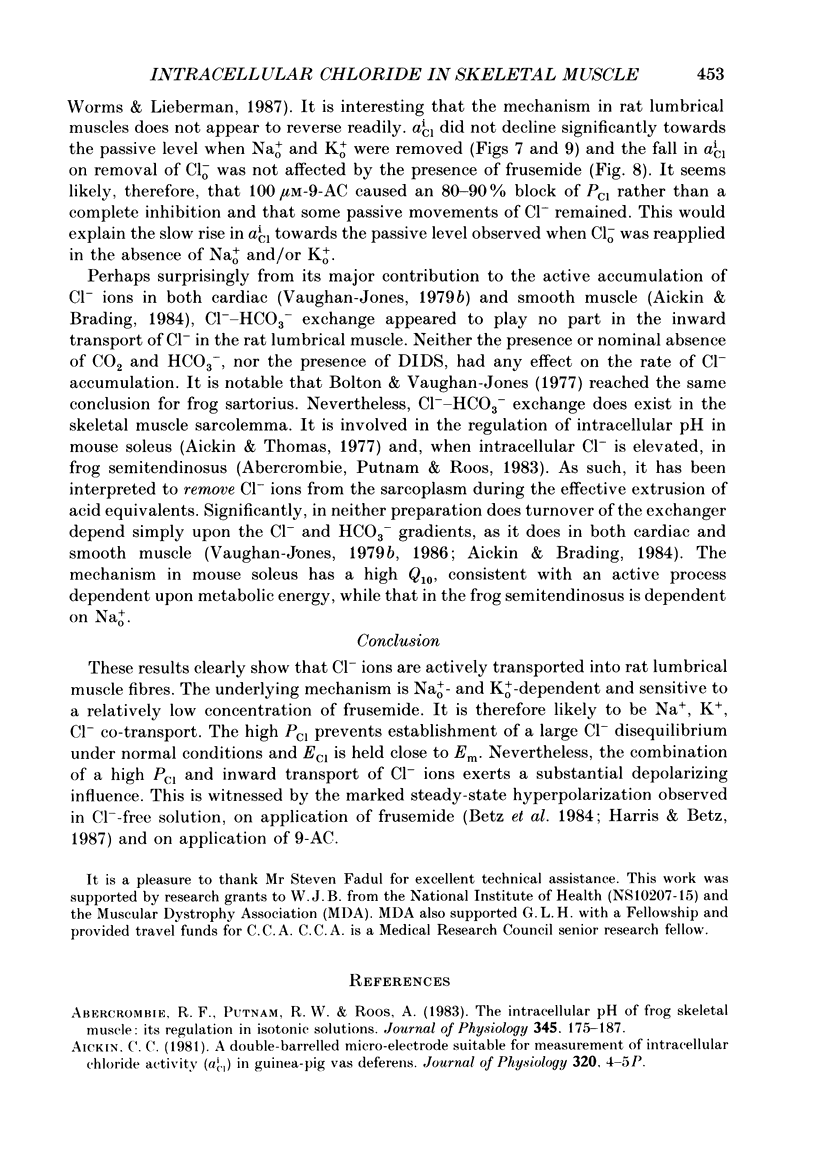
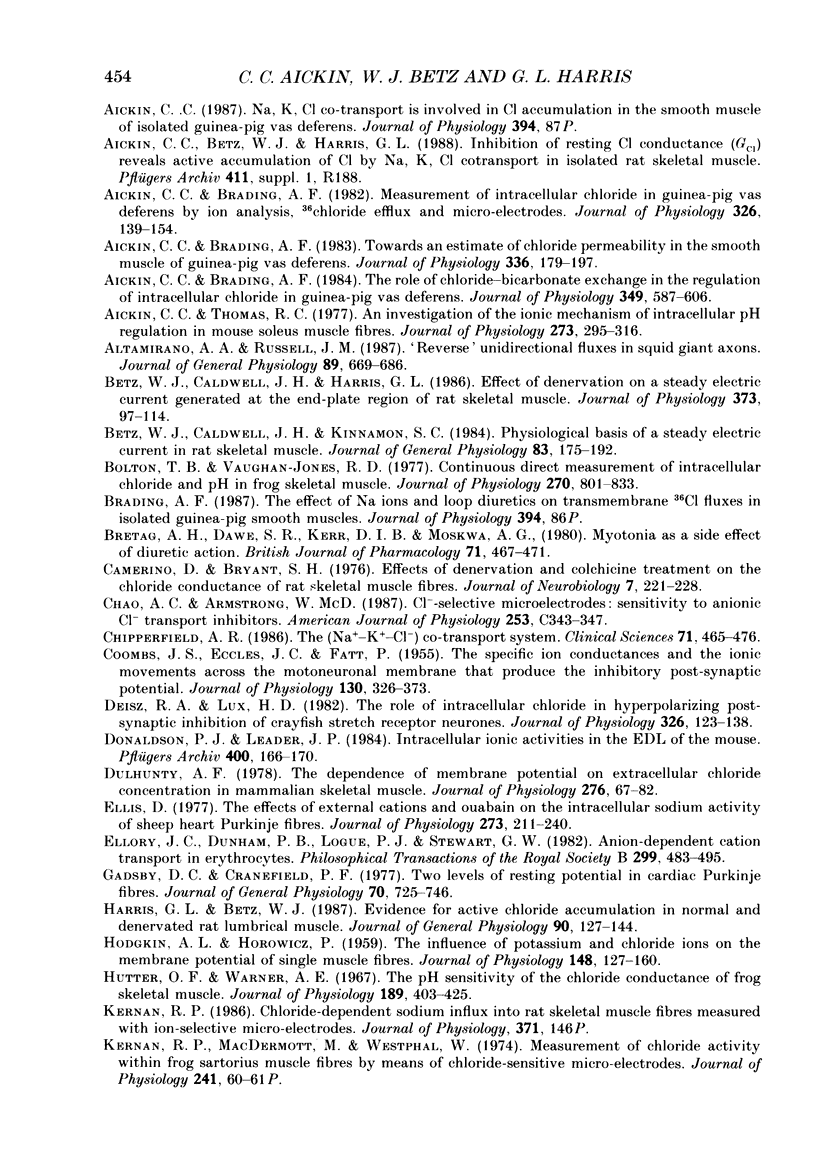
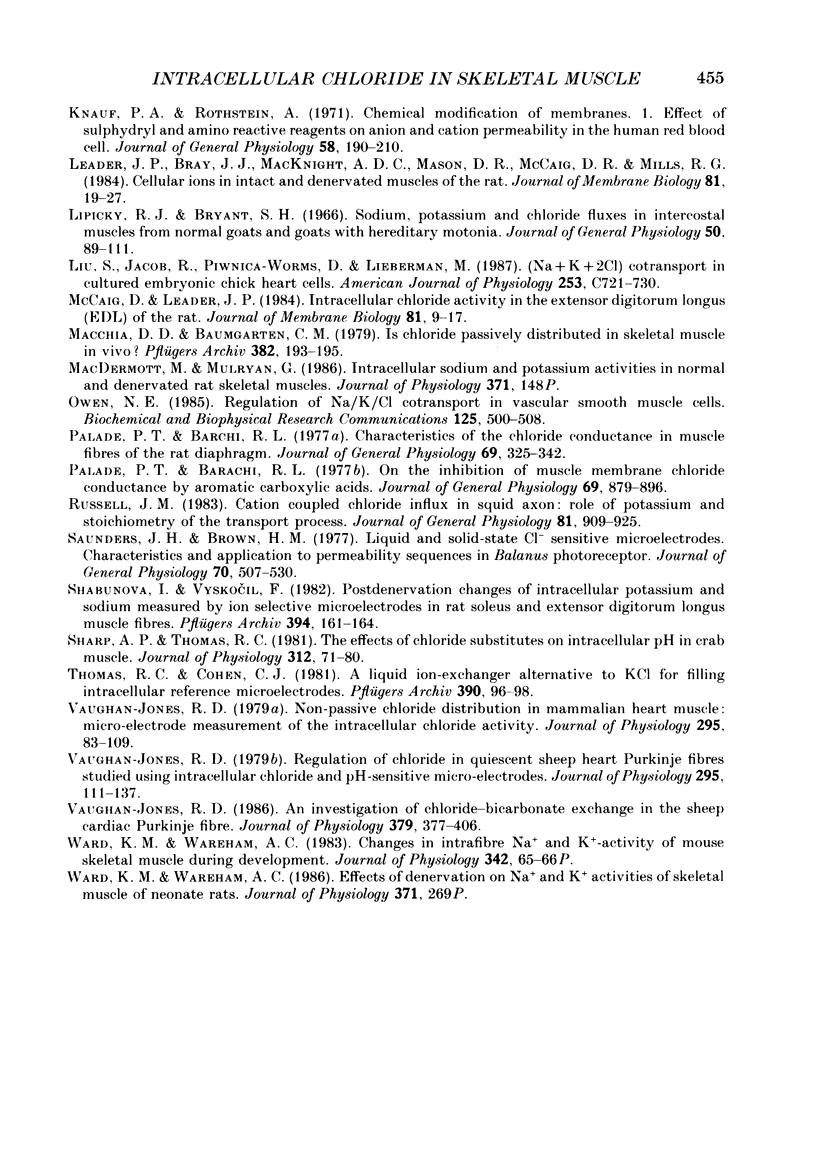
Selected References
These references are in PubMed. This may not be the complete list of references from this article.
- Abercrombie R. F., Putnam R. W., Roos A. The intracellular pH of frog skeletal muscle: its regulation in isotonic solutions. J Physiol. 1983 Dec;345:175–187. doi: 10.1113/jphysiol.1983.sp014973. [DOI] [PMC free article] [PubMed] [Google Scholar]
- Aickin C. C., Brading A. F. Measurement of intracellular chloride in guinea-pig vas deferens by ion analysis, 36chloride efflux and micro-electrodes. J Physiol. 1982 May;326:139–154. doi: 10.1113/jphysiol.1982.sp014182. [DOI] [PMC free article] [PubMed] [Google Scholar]
- Aickin C. C., Brading A. F. The role of chloride-bicarbonate exchange in the regulation of intracellular chloride in guinea-pig vas deferens. J Physiol. 1984 Apr;349:587–606. doi: 10.1113/jphysiol.1984.sp015175. [DOI] [PMC free article] [PubMed] [Google Scholar]
- Aickin C. C., Brading A. F. Towards an estimate of chloride permeability in the smooth muscle of guinea-pig vas deferens. J Physiol. 1983 Mar;336:179–197. doi: 10.1113/jphysiol.1983.sp014575. [DOI] [PMC free article] [PubMed] [Google Scholar]
- Aickin C. C., Thomas R. C. An investigation of the ionic mechanism of intracellular pH regulation in mouse soleus muscle fibres. J Physiol. 1977 Dec;273(1):295–316. doi: 10.1113/jphysiol.1977.sp012095. [DOI] [PMC free article] [PubMed] [Google Scholar]
- Altamirano A. A., Russell J. M. Coupled Na/K/Cl efflux. "Reverse" unidirectional fluxes in squid giant axons. J Gen Physiol. 1987 May;89(5):669–686. doi: 10.1085/jgp.89.5.669. [DOI] [PMC free article] [PubMed] [Google Scholar]
- Betz W. J., Caldwell J. H., Harris G. L. Effect of denervation on a steady electric current generated at the end-plate region of rat skeletal muscle. J Physiol. 1986 Apr;373:97–114. doi: 10.1113/jphysiol.1986.sp016037. [DOI] [PMC free article] [PubMed] [Google Scholar]
- Betz W. J., Caldwell J. H., Kinnamon S. C. Physiological basis of a steady endogenous current in rat lumbrical muscle. J Gen Physiol. 1984 Feb;83(2):175–192. doi: 10.1085/jgp.83.2.175. [DOI] [PMC free article] [PubMed] [Google Scholar]
- Bolton T. B., Vaughan-Jones R. D. Continuous direct measurement of intracellular chloride and pH in frog skeletal muscle. J Physiol. 1977 Sep;270(3):801–833. doi: 10.1113/jphysiol.1977.sp011983. [DOI] [PMC free article] [PubMed] [Google Scholar]
- Bretag A. H., Dawe S. R., Kerr D. I., Moskwa A. G. Myotonia as a side effect of diuretic action. Br J Pharmacol. 1980;71(2):467–471. doi: 10.1111/j.1476-5381.1980.tb10959.x. [DOI] [PMC free article] [PubMed] [Google Scholar]
- COOMBS J. S., ECCLES J. C., FATT P. The specific ionic conductances and the ionic movements across the motoneuronal membrane that produce the inhibitory post-synaptic potential. J Physiol. 1955 Nov 28;130(2):326–374. doi: 10.1113/jphysiol.1955.sp005412. [DOI] [PMC free article] [PubMed] [Google Scholar]
- Camerino D., Bryant S. H. Effects of denervation and colchicine treatment on the chloride conductance of rat skeletal muscle fibers. J Neurobiol. 1976 May;7(3):221–228. doi: 10.1002/neu.480070305. [DOI] [PubMed] [Google Scholar]
- Chao A. C., Armstrong W. M. Cl(-)-selective microelectrodes: sensitivity to anionic Cl- transport inhibitors. Am J Physiol. 1987 Aug;253(2 Pt 1):C343–C347. doi: 10.1152/ajpcell.1987.253.2.C343. [DOI] [PubMed] [Google Scholar]
- Chipperfield A. R. The (Na+-K+-Cl-) co-transport system. Clin Sci (Lond) 1986 Nov;71(5):465–476. doi: 10.1042/cs0710465. [DOI] [PubMed] [Google Scholar]
- Deisz R. A., Lux H. D. The role of intracellular chloride in hyperpolarizing post-synaptic inhibition of crayfish stretch receptor neurones. J Physiol. 1982 May;326:123–138. doi: 10.1113/jphysiol.1982.sp014181. [DOI] [PMC free article] [PubMed] [Google Scholar]
- Donaldson P. J., Leader J. P. Intracellular ionic activities in the EDL muscle of the mouse. Pflugers Arch. 1984 Feb;400(2):166–170. doi: 10.1007/BF00585034. [DOI] [PubMed] [Google Scholar]
- Dulhunty A. F. The dependence of membrane potential on extracellular chloride concentration in mammalian skeletal muscle fibres. J Physiol. 1978 Mar;276:67–82. doi: 10.1113/jphysiol.1978.sp012220. [DOI] [PMC free article] [PubMed] [Google Scholar]
- Ellis D. The effects of external cations and ouabain on the intracellular sodium activity of sheep heart Purkinje fibres. J Physiol. 1977 Dec;273(1):211–240. doi: 10.1113/jphysiol.1977.sp012090. [DOI] [PMC free article] [PubMed] [Google Scholar]
- Ellory J. C., Dunham P. B., Logue P. J., Stewart G. W. Anion-dependent cation transport in erythrocytes. Philos Trans R Soc Lond B Biol Sci. 1982 Dec 1;299(1097):483–495. doi: 10.1098/rstb.1982.0146. [DOI] [PubMed] [Google Scholar]
- Gadsby D. C., Cranefield P. F. Two levels of resting potential in cardiac Purkinje fibers. J Gen Physiol. 1977 Dec;70(6):725–746. doi: 10.1085/jgp.70.6.725. [DOI] [PMC free article] [PubMed] [Google Scholar]
- HODGKIN A. L., HOROWICZ P. The influence of potassium and chloride ions on the membrane potential of single muscle fibres. J Physiol. 1959 Oct;148:127–160. doi: 10.1113/jphysiol.1959.sp006278. [DOI] [PMC free article] [PubMed] [Google Scholar]
- Harris G. L., Betz W. J. Evidence for active chloride accumulation in normal and denervated rat lumbrical muscle. J Gen Physiol. 1987 Jul;90(1):127–144. doi: 10.1085/jgp.90.1.127. [DOI] [PMC free article] [PubMed] [Google Scholar]
- Hutter O. F., Warner A. E. The pH sensitivity of the chloride conductance of frog skeletal muscle. J Physiol. 1967 Apr;189(3):403–425. doi: 10.1113/jphysiol.1967.sp008176. [DOI] [PMC free article] [PubMed] [Google Scholar]
- Kernan R. P., MacDermott M., Westphal W. Proceedings: Measurement of chloride activity within frog sartorius muscle fibres by means of chloride-sensitive micro-electrodes. J Physiol. 1974 Aug;241(1):60P–61P. [PubMed] [Google Scholar]
- Knauf P. A., Rothstein A. Chemical modification of membranes. I. Effects of sulfhydryl and amino reactive reagents on anion and cation permeability of the human red blood cell. J Gen Physiol. 1971 Aug;58(2):190–210. doi: 10.1085/jgp.58.2.190. [DOI] [PMC free article] [PubMed] [Google Scholar]
- Leader J. P., Bray J. J., Macknight A. D., Mason D. R., McCaig D., Mills R. G. Cellular ions in intact and denervated muscles of the rat. J Membr Biol. 1984;81(1):19–27. doi: 10.1007/BF01868806. [DOI] [PubMed] [Google Scholar]
- Lipicky R. J., Bryant S. H. Sodium, potassium, and chloride fluxes in intercostal muscle from normal goats and goats with hereditary myotonia. J Gen Physiol. 1966 Sep;50(1):89–111. doi: 10.1085/jgp.50.1.89. [DOI] [PMC free article] [PubMed] [Google Scholar]
- Liu S., Jacob R., Piwnica-Worms D., Lieberman M. (Na + K + 2Cl) cotransport in cultured embryonic chick heart cells. Am J Physiol. 1987 Nov;253(5 Pt 1):C721–C730. doi: 10.1152/ajpcell.1987.253.5.C721. [DOI] [PubMed] [Google Scholar]
- Macchia D. D., Baumgarten C. M. Is chloride passively distributed in skeletal muscle in vivo? Pflugers Arch. 1979 Nov;382(2):193–195. doi: 10.1007/BF00584222. [DOI] [PubMed] [Google Scholar]
- McCaig D., Leader J. P. Intracellular chloride activity in the extensor digitorum longus (EDL) muscle of the rat. J Membr Biol. 1984;81(1):9–17. doi: 10.1007/BF01868805. [DOI] [PubMed] [Google Scholar]
- Owen N. E. Regulation of Na/K/Cl cotransport in vascular smooth muscle cells. Biochem Biophys Res Commun. 1984 Dec 14;125(2):500–508. doi: 10.1016/0006-291x(84)90568-0. [DOI] [PubMed] [Google Scholar]
- Palade P. T., Barchi R. L. Characteristics of the chloride conductance in muscle fibers of the rat diaphragm. J Gen Physiol. 1977 Mar;69(3):325–342. doi: 10.1085/jgp.69.3.325. [DOI] [PMC free article] [PubMed] [Google Scholar]
- Palade P. T., Barchi R. L. On the inhibition of muscle membrane chloride conductance by aromatic carboxylic acids. J Gen Physiol. 1977 Jun;69(6):879–896. doi: 10.1085/jgp.69.6.879. [DOI] [PMC free article] [PubMed] [Google Scholar]
- Russell J. M. Cation-coupled chloride influx in squid axon. Role of potassium and stoichiometry of the transport process. J Gen Physiol. 1983 Jun;81(6):909–925. doi: 10.1085/jgp.81.6.909. [DOI] [PMC free article] [PubMed] [Google Scholar]
- Saunders J. H., Brown H. M. Liquid and solid-state Cl- -sensitive microelectrodes. Characteristics and application to intracellular Cl- activity in Balanus photoreceptor. J Gen Physiol. 1977 Oct;70(4):507–530. doi: 10.1085/jgp.70.4.507. [DOI] [PMC free article] [PubMed] [Google Scholar]
- Shabunova I., Vyskocil F. Postdenervation changes of intracellular potassium and sodium measured by ion selective microelectrodes in rat soleus and extensor digitorum longus muscle fibres. Pflugers Arch. 1982 Aug;394(2):161–164. doi: 10.1007/BF00582919. [DOI] [PubMed] [Google Scholar]
- Sharp A. P., Thomas R. C. The effects of chloride substitution on intracellular pH in crab muscle. J Physiol. 1981 Mar;312:71–80. doi: 10.1113/jphysiol.1981.sp013616. [DOI] [PMC free article] [PubMed] [Google Scholar]
- Thomas R. C., Cohen C. J. A liquid ion-exchanger alternative to KCl for filling intracellular reference microelectrodes. Pflugers Arch. 1981 Apr;390(1):96–98. doi: 10.1007/BF00582719. [DOI] [PubMed] [Google Scholar]
- Vaughan-Jones R. D. An investigation of chloride-bicarbonate exchange in the sheep cardiac Purkinje fibre. J Physiol. 1986 Oct;379:377–406. doi: 10.1113/jphysiol.1986.sp016259. [DOI] [PMC free article] [PubMed] [Google Scholar]
- Vaughan-Jones R. D. Non-passive chloride distribution in mammalian heart muscle: micro-electrode measurement of the intracellular chloride activity. J Physiol. 1979 Oct;295:83–109. doi: 10.1113/jphysiol.1979.sp012956. [DOI] [PMC free article] [PubMed] [Google Scholar]
- Vaughan-Jones R. D. Regulation of chloride in quiescent sheep-heart Purkinje fibres studied using intracellular chloride and pH-sensitive micro-electrodes. J Physiol. 1979 Oct;295:111–137. doi: 10.1113/jphysiol.1979.sp012957. [DOI] [PMC free article] [PubMed] [Google Scholar]