Abstract
1. The effects of the Na+ electrochemical potential gradient on gamma-aminobutyric acid (GABA)-induced Cl- currents (ICl) in frog sensory neurones were studied, using a suction pipette technique with which internal perfusion can be accomplished under current- and voltage-clamp conditions. 2. Under current clamp, the depolarizing response to GABA decreased in the presence of external Na+. A similar external Na+-dependent reduction in the GABA-induced inward ICl was observed under voltage clamp. The reversal potential of GABA-induced ICl (EGABA) was nearly equal to the Cl- equilibrium potential (ECl), irrespective of the presence or absence of external Na+. 3. Varying the Na+ influx by changing the holding membrane potential (VH) altered the GABA response: the GABA-induced ICl decreased progressively as VH became more negative. 4. The effects of changing the external and internal Na+ concentrations ([Na+]o and [Na+]i) on the GABA-induced ICl were also studied. Increasing [Na+]o at a constant [Na+]i reduced this current while increasing [Na+]i at a fixed [Na+]o facilitated it. 5. A high temperature coefficient of about 3 was estimated with respect to the percentage reduction in GABA-induced ICl due to [Na+]o. 6. These results indicate that the [Na+]o-dependent suppression of GABA-induced ICl was mediated chiefly by the uptake of GABA subserved by a Na-GABA co-transport mechanism. 7. GABA dose-response measurements were made with and without external Na+. The [Na+]o-induced suppression was more pronounced in relative amount at lower concentrations and in absolute amount at intermediate concentrations. Analysis of these data indicates, however, that the Na+-coupled GABA influx kept increasing at GABA concentrations high enough to nearly saturate GABA-induced ICl, and the same saturating level was observed as in the Na+-free case. This indicates that the electrogenic co-transport current was much smaller so that our measurements of GABA-induced ICl' were contaminated very little. Thus, the present method based on recording of GABA-induced ICl was legitimate for the analysis of the Na-GABA co-transport. 8. By analysing the [Na+]o-dependent suppression of GABA-induced ICl, the stoichiometric ratio of the underlying co-transport was estimated to be one: one Na+ ion per GABA molecule. 9. The ICl induced by GABA agonists such as beta-alanine, taurine, l-GABOB (l-gamma-amino-beta-hydroxybutyric acid) and muscimol was not affected by the amount of external Na+ present, suggesting difference in the affinity between receptor and transport carrier.
Full text
PDF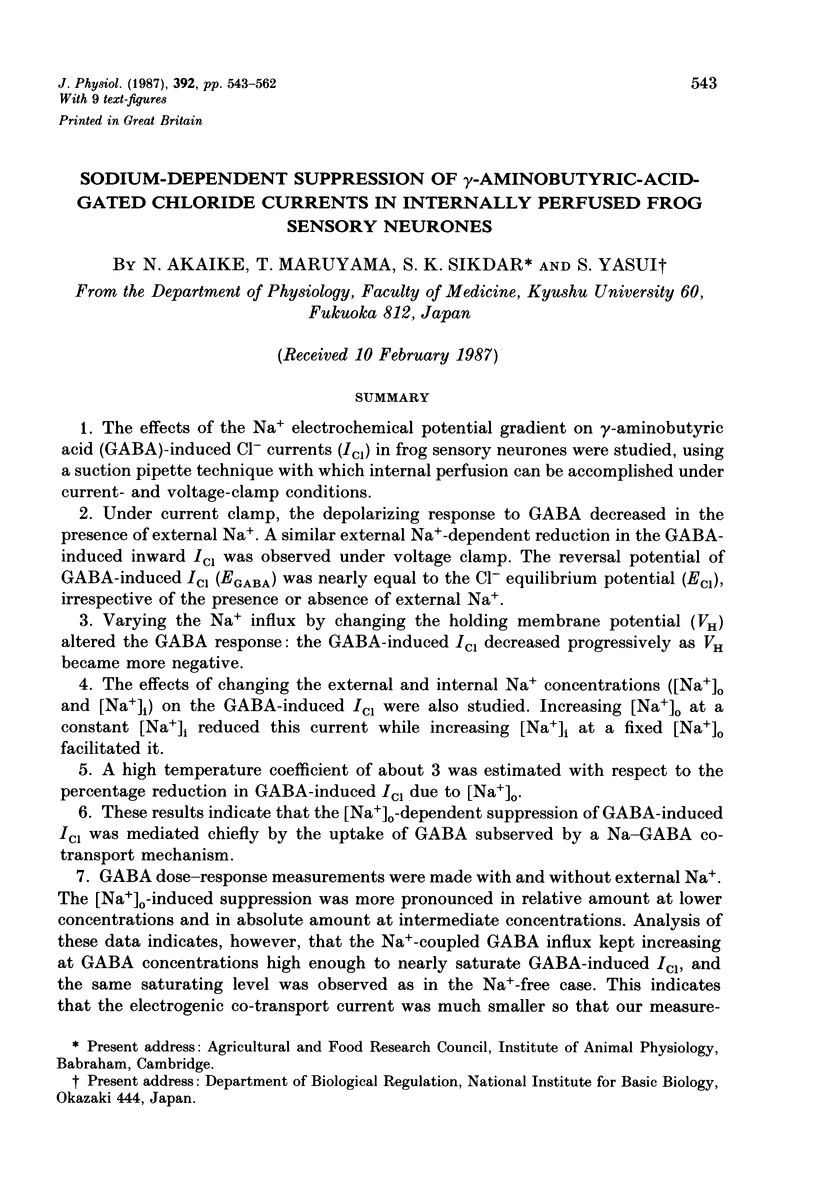
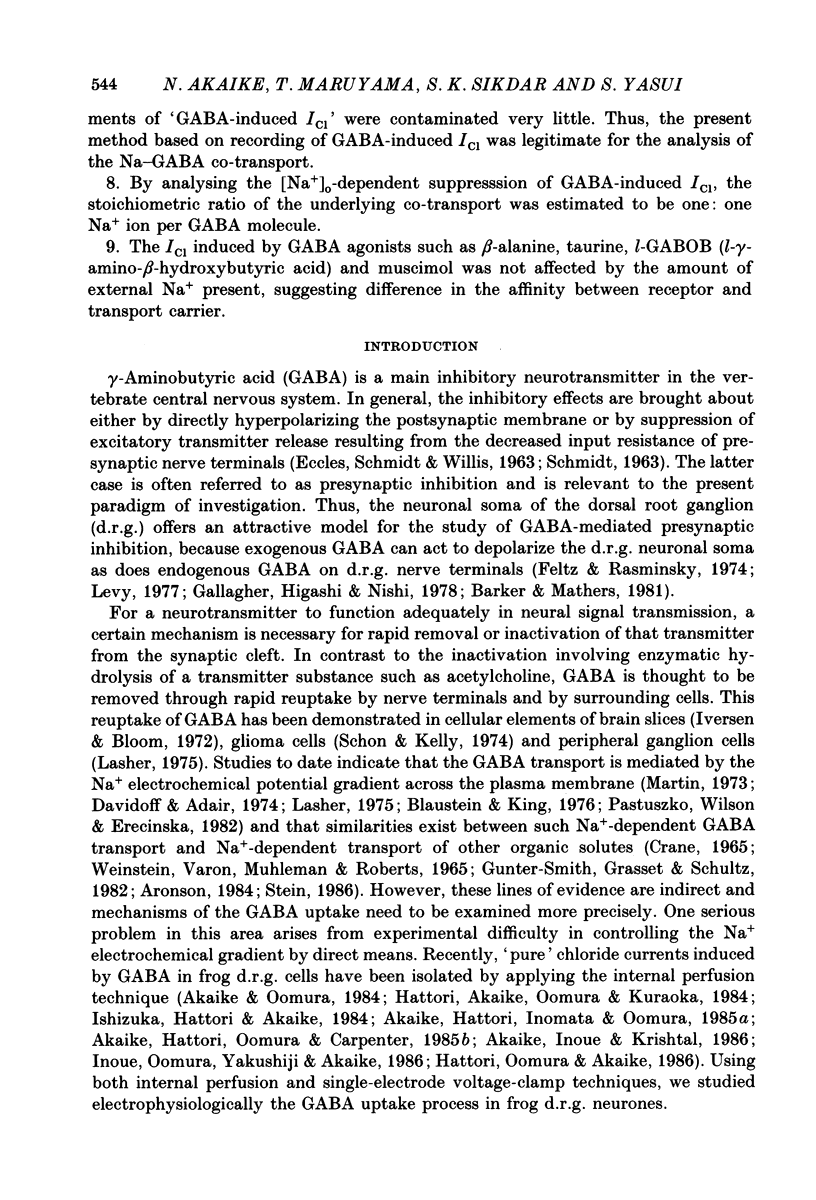
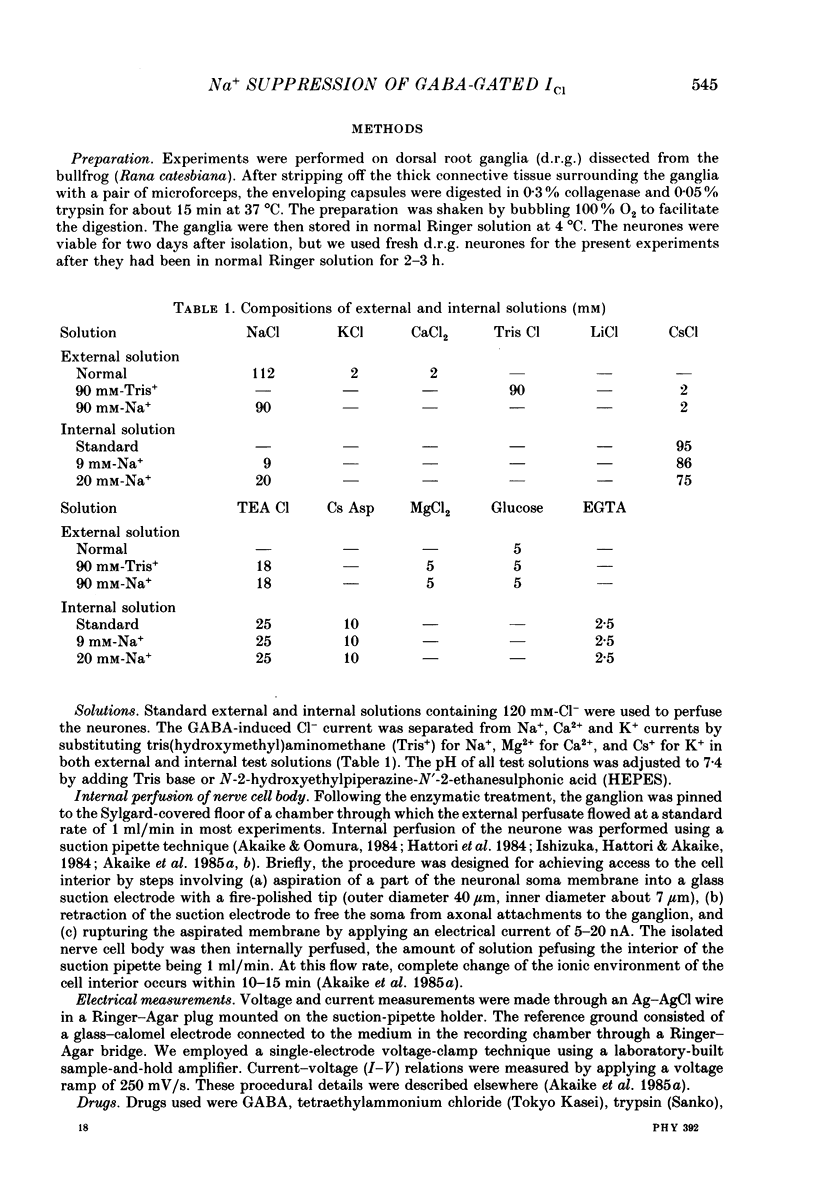
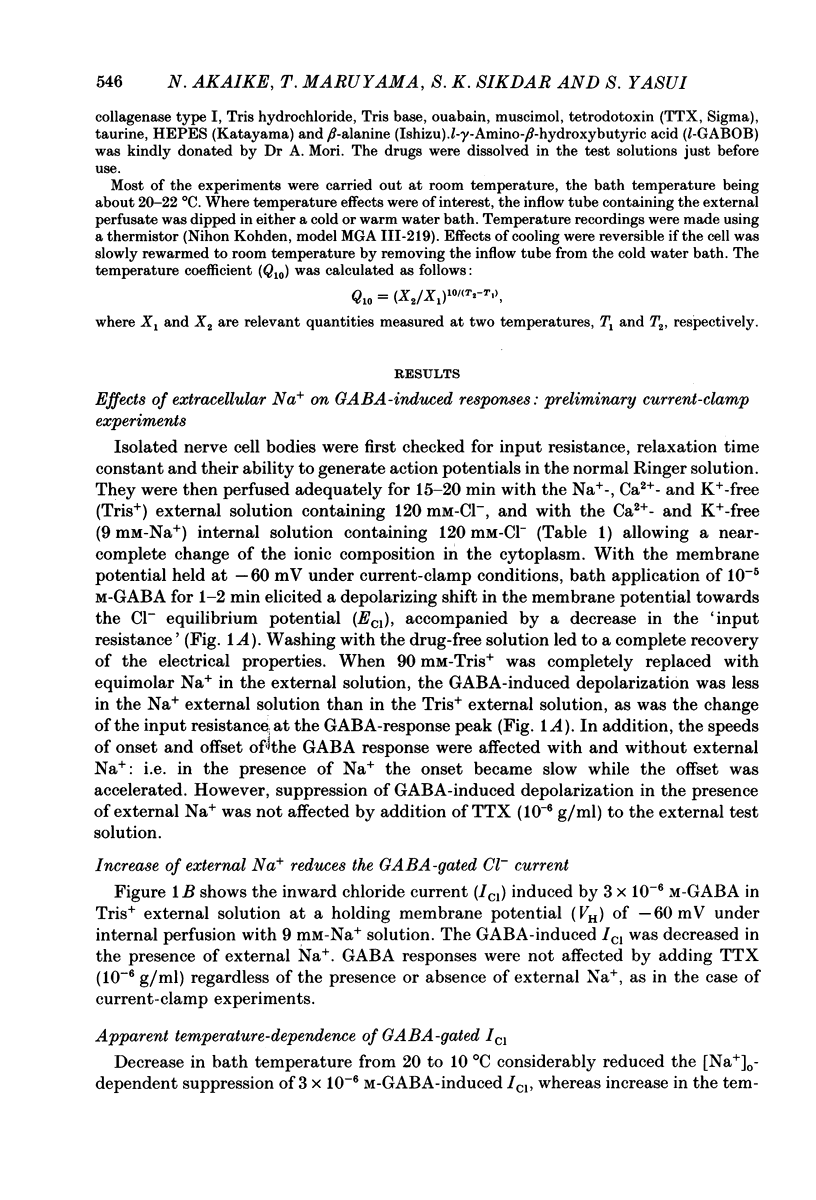
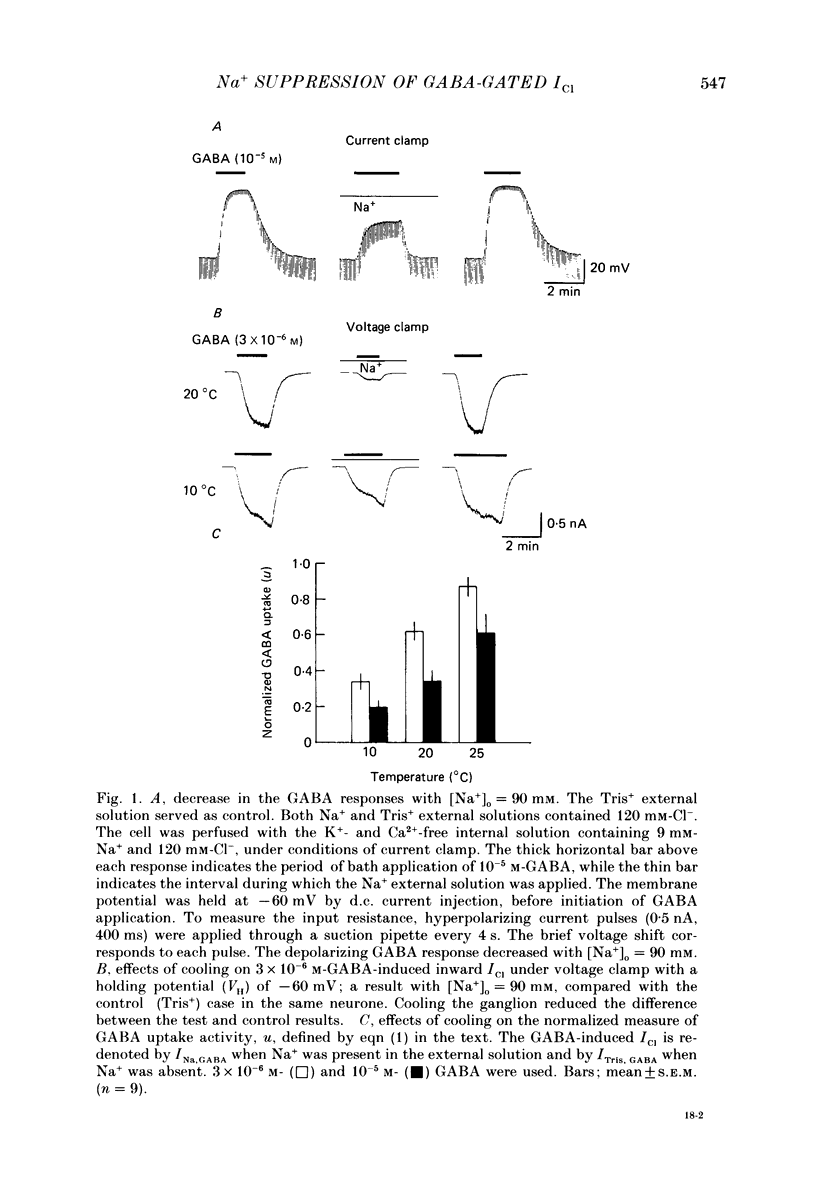
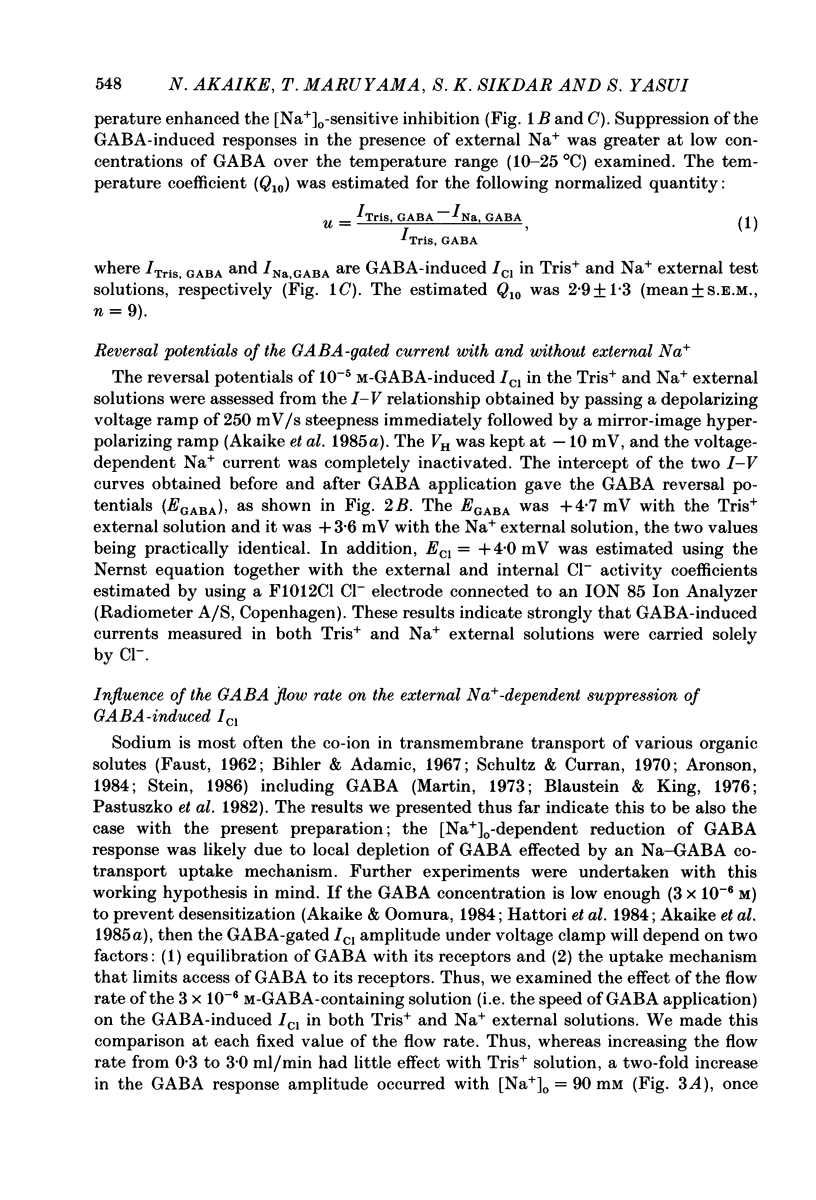
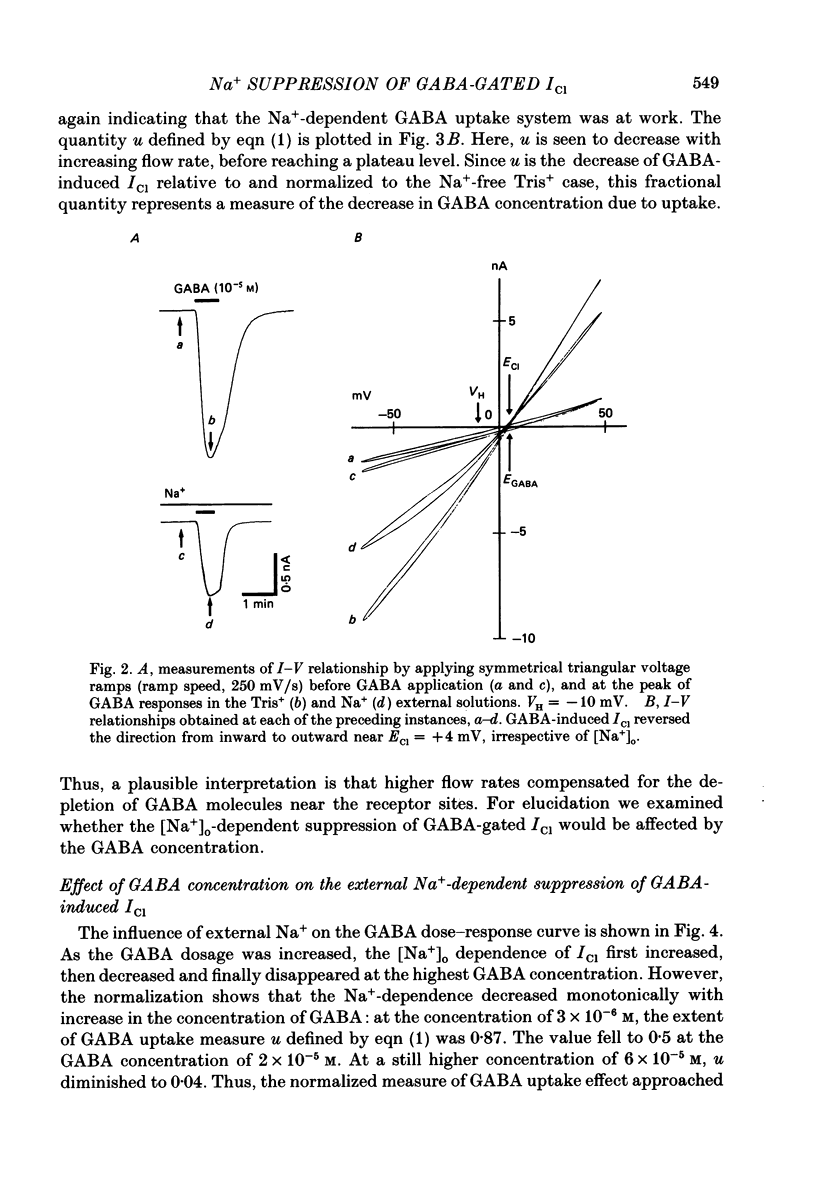
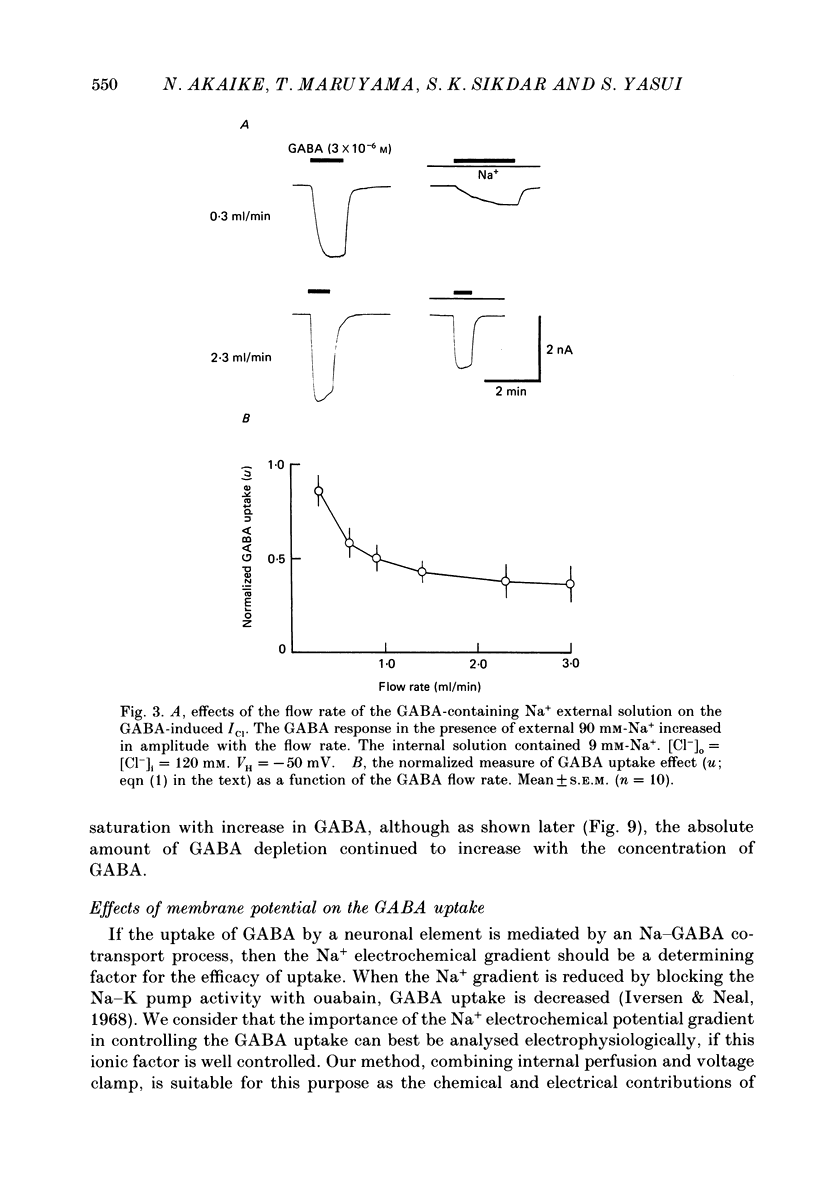
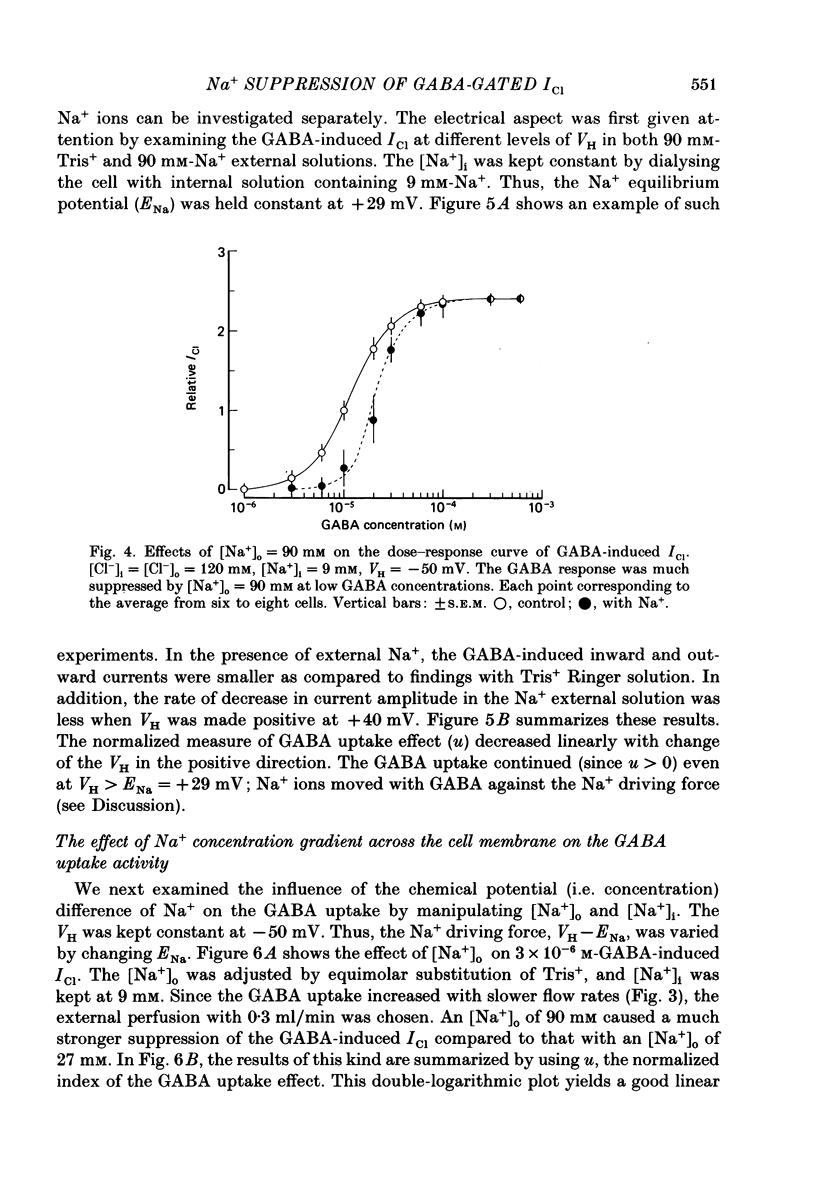
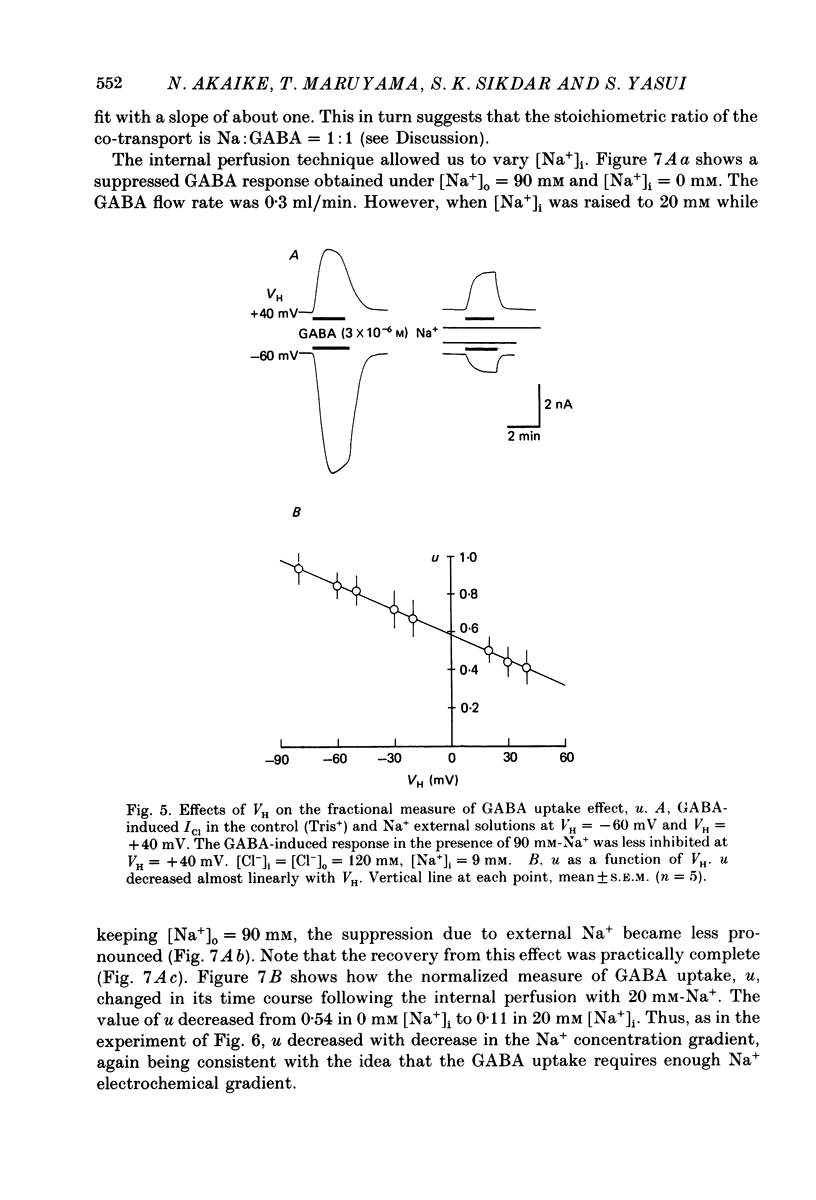
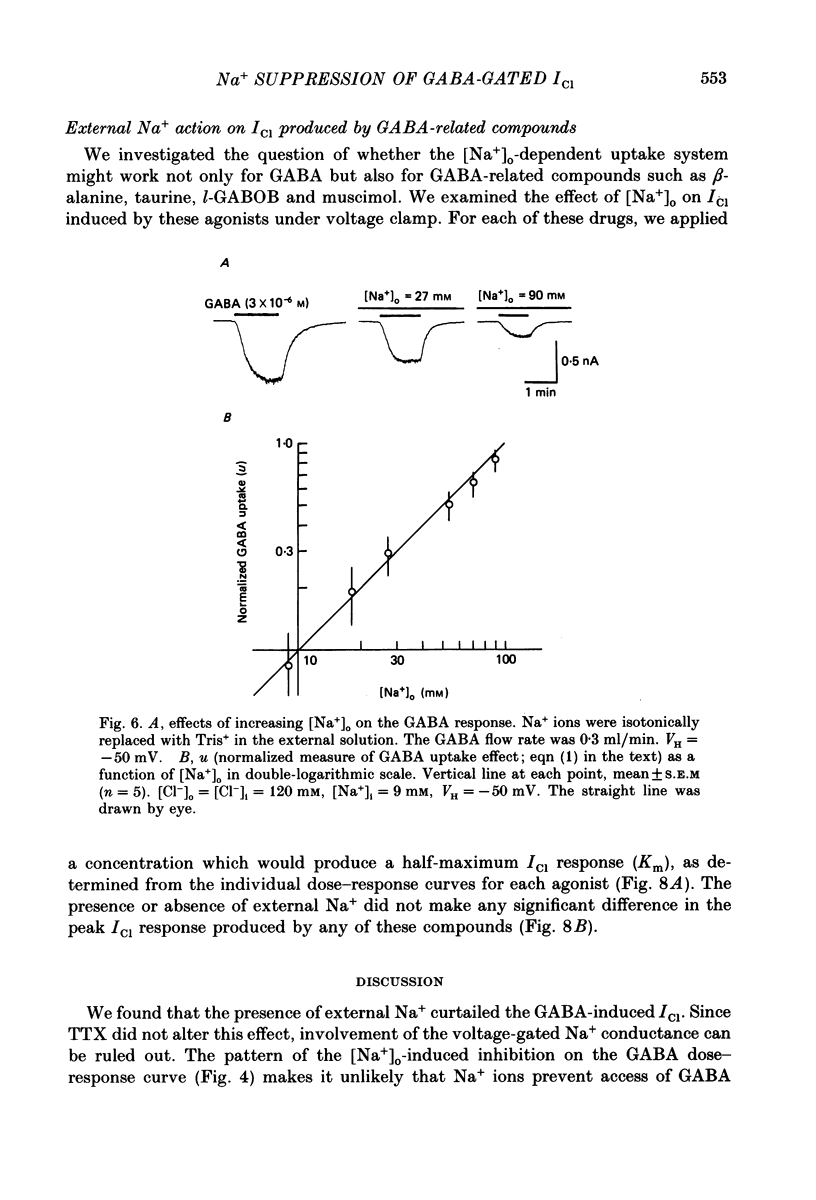
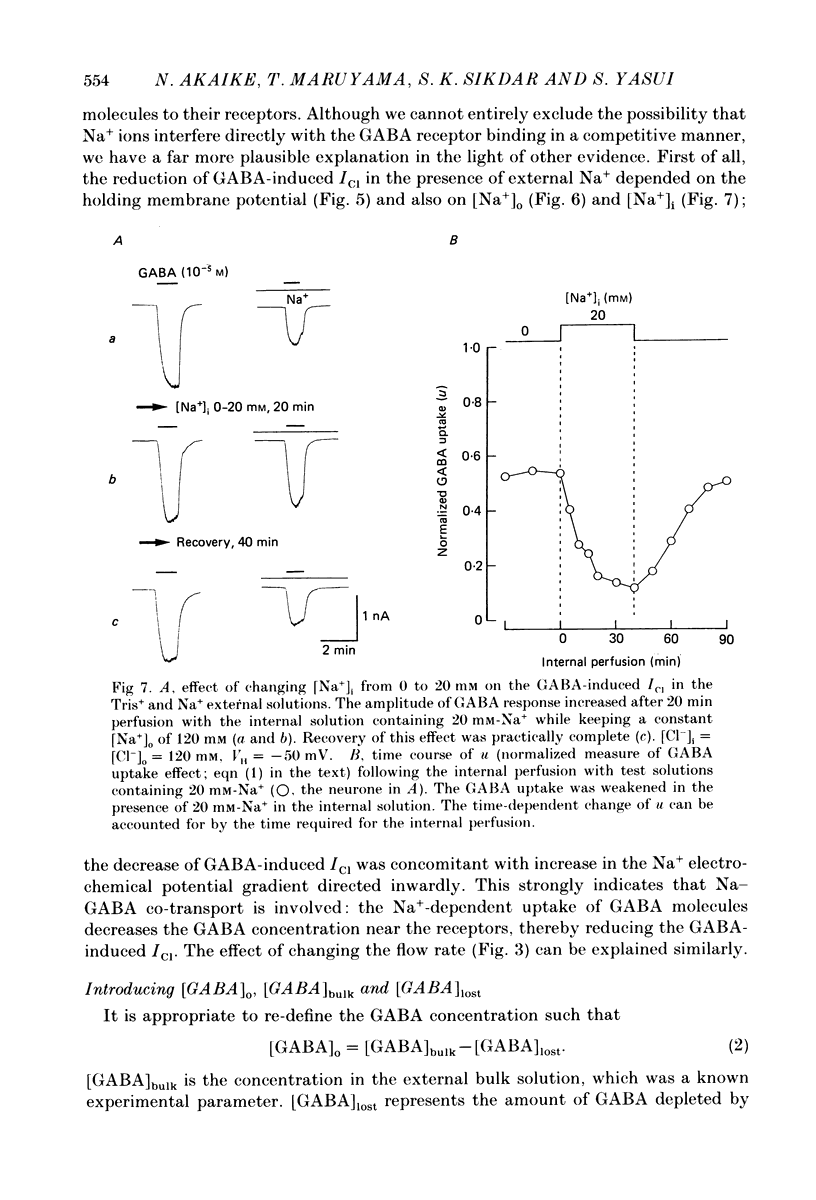
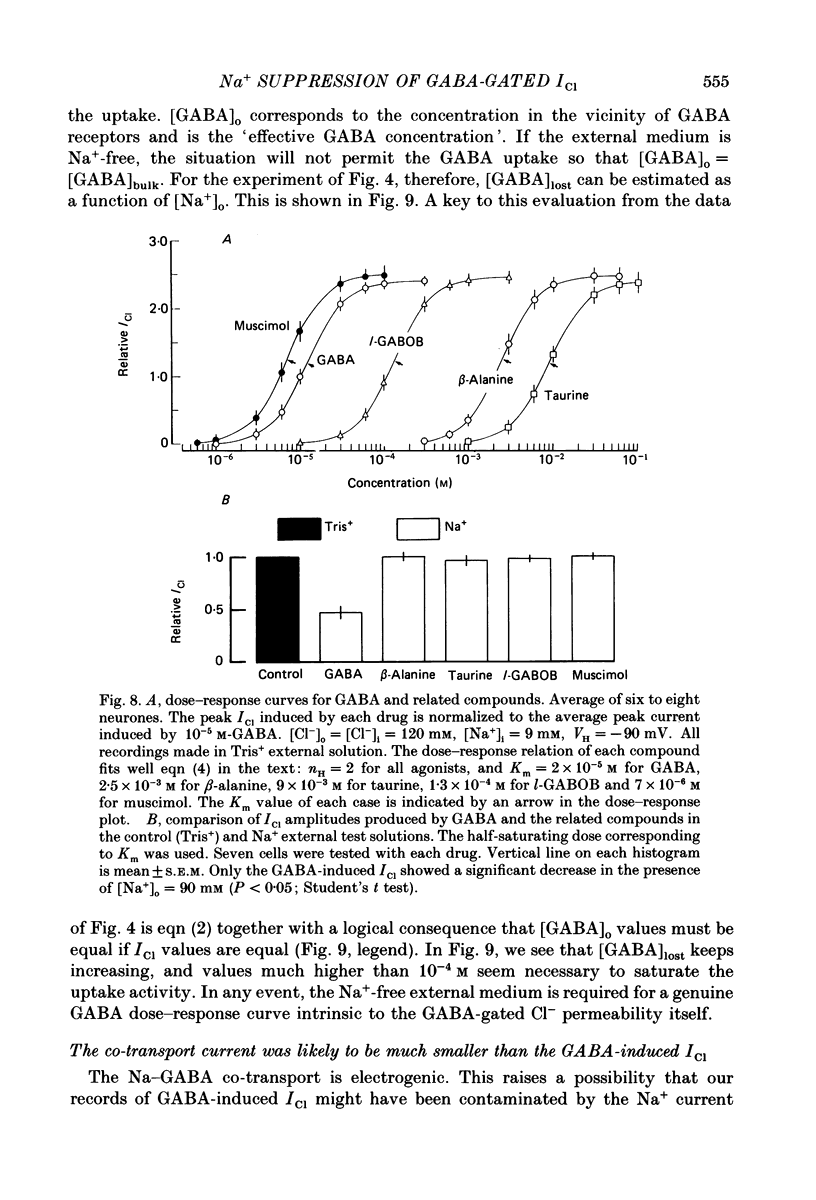
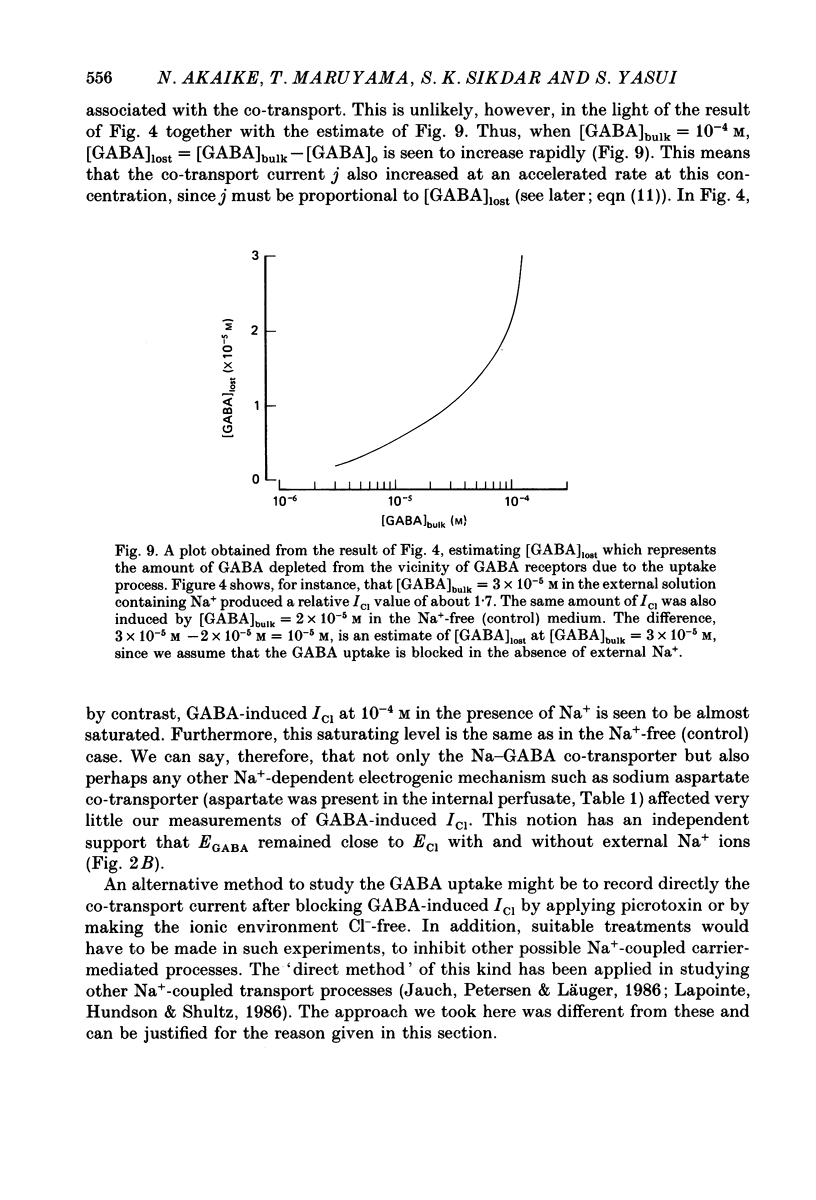
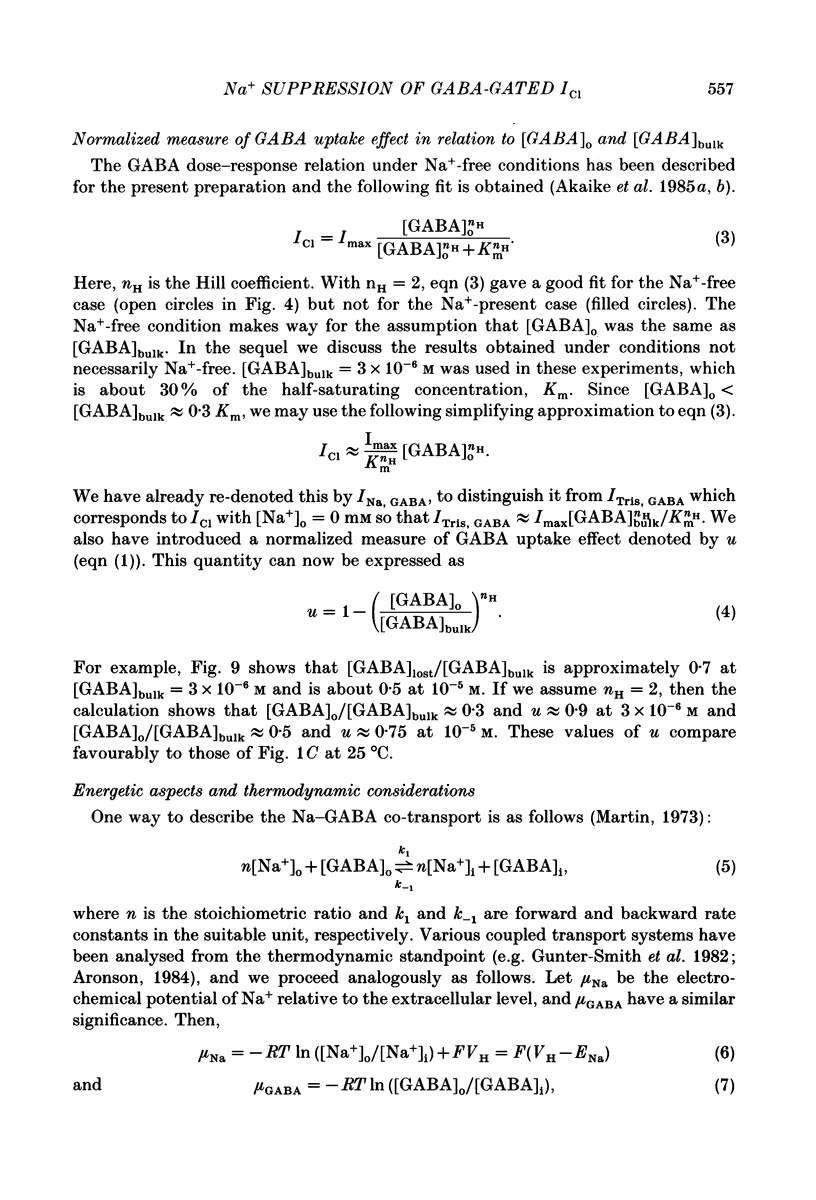
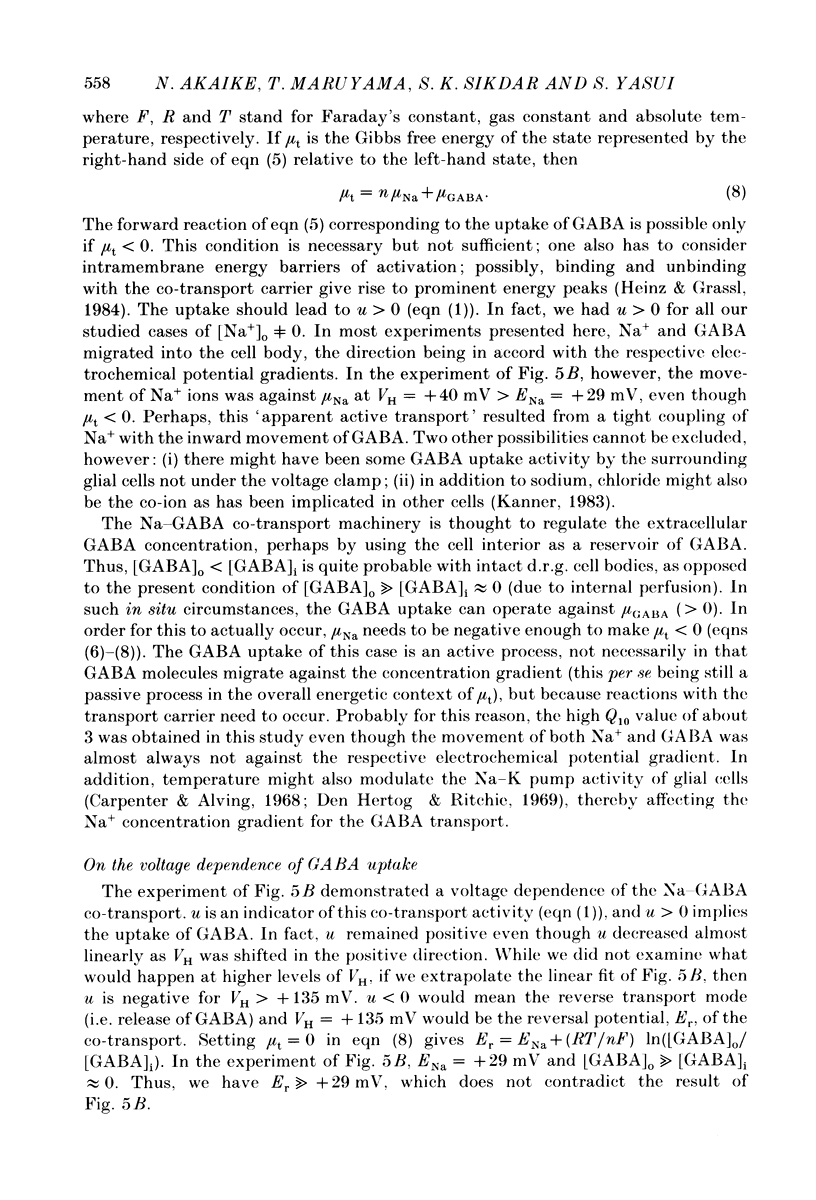
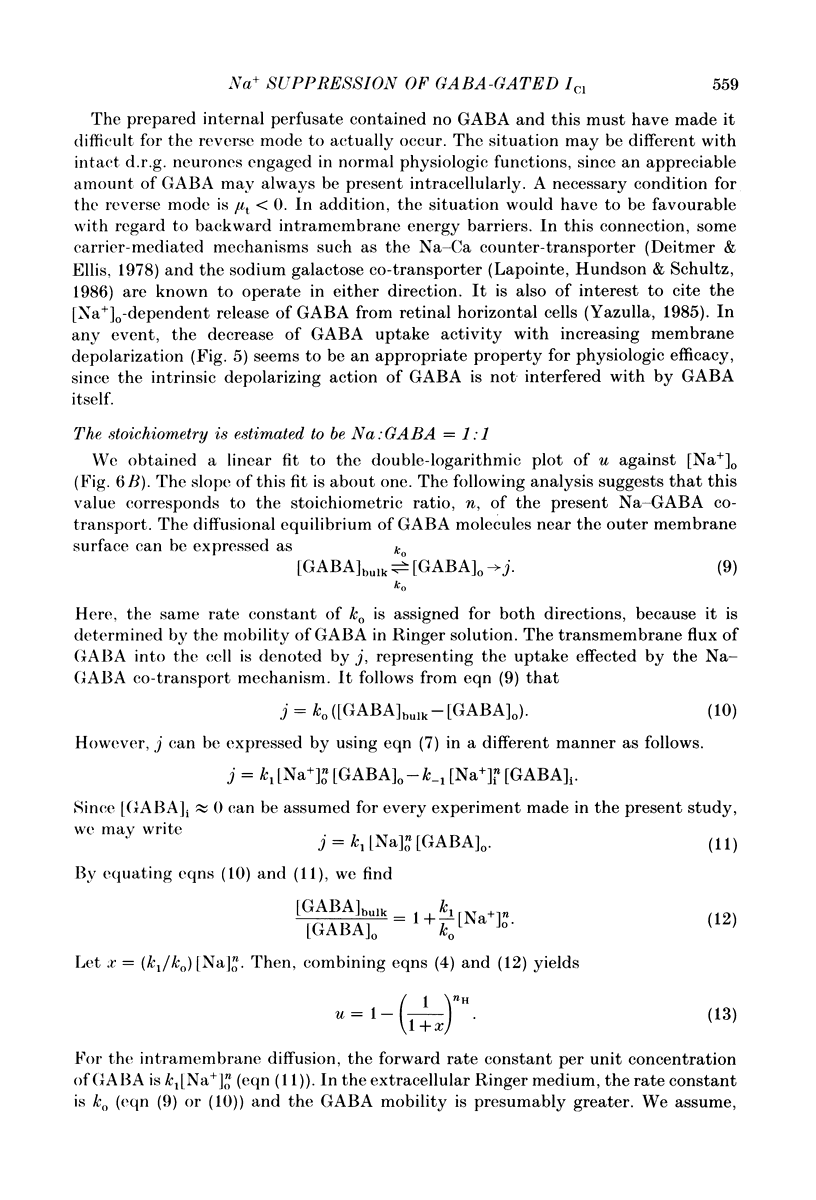
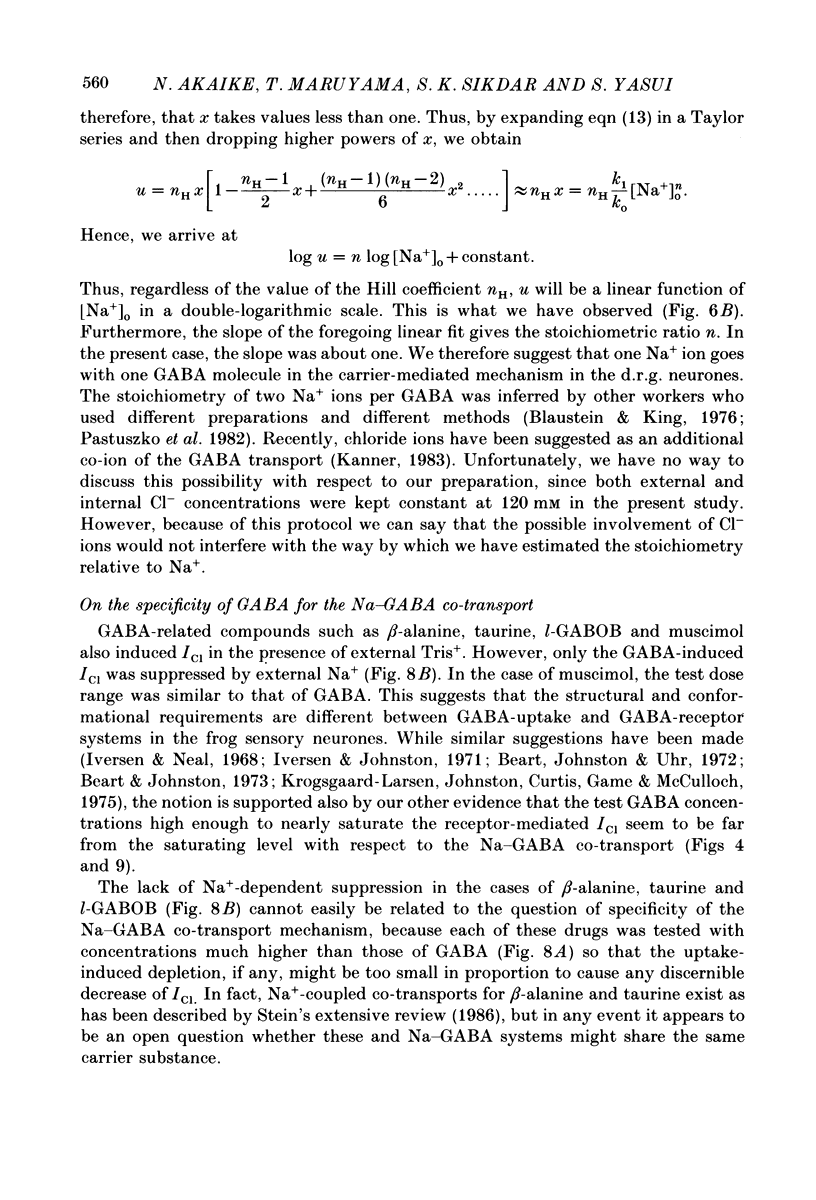
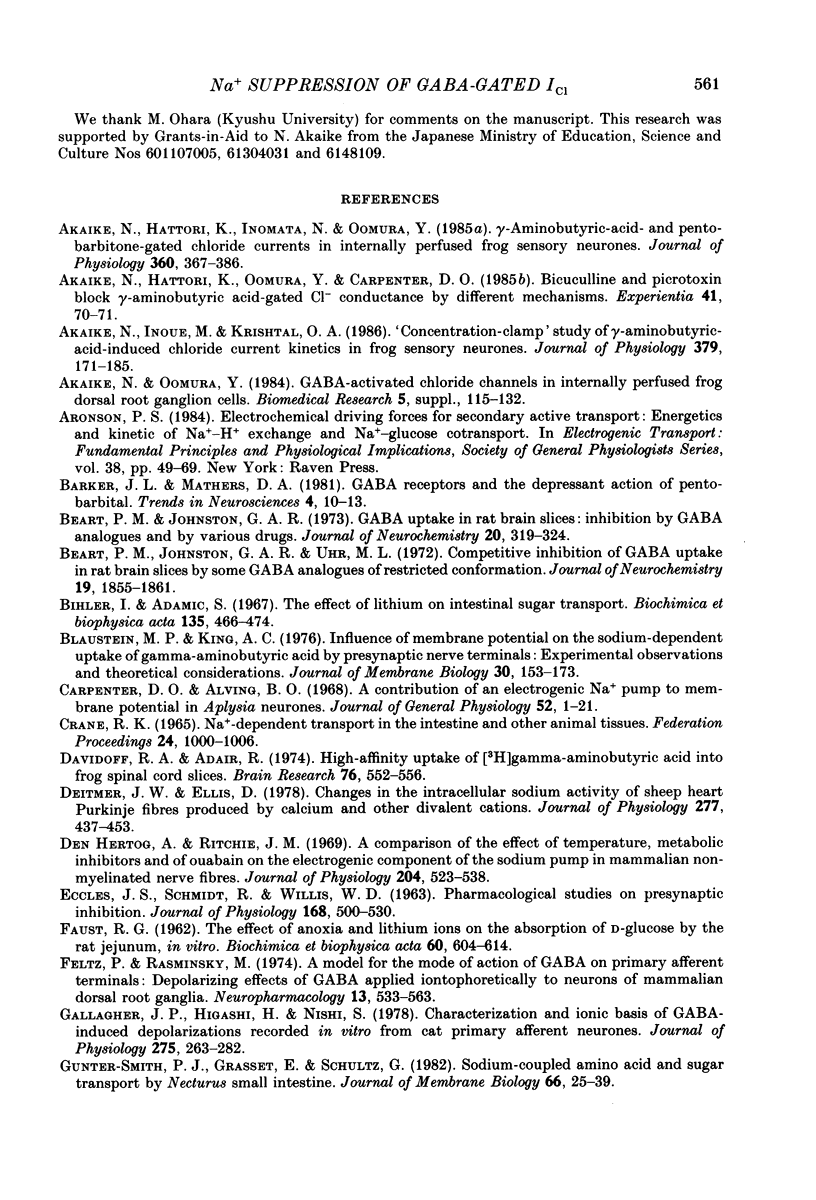
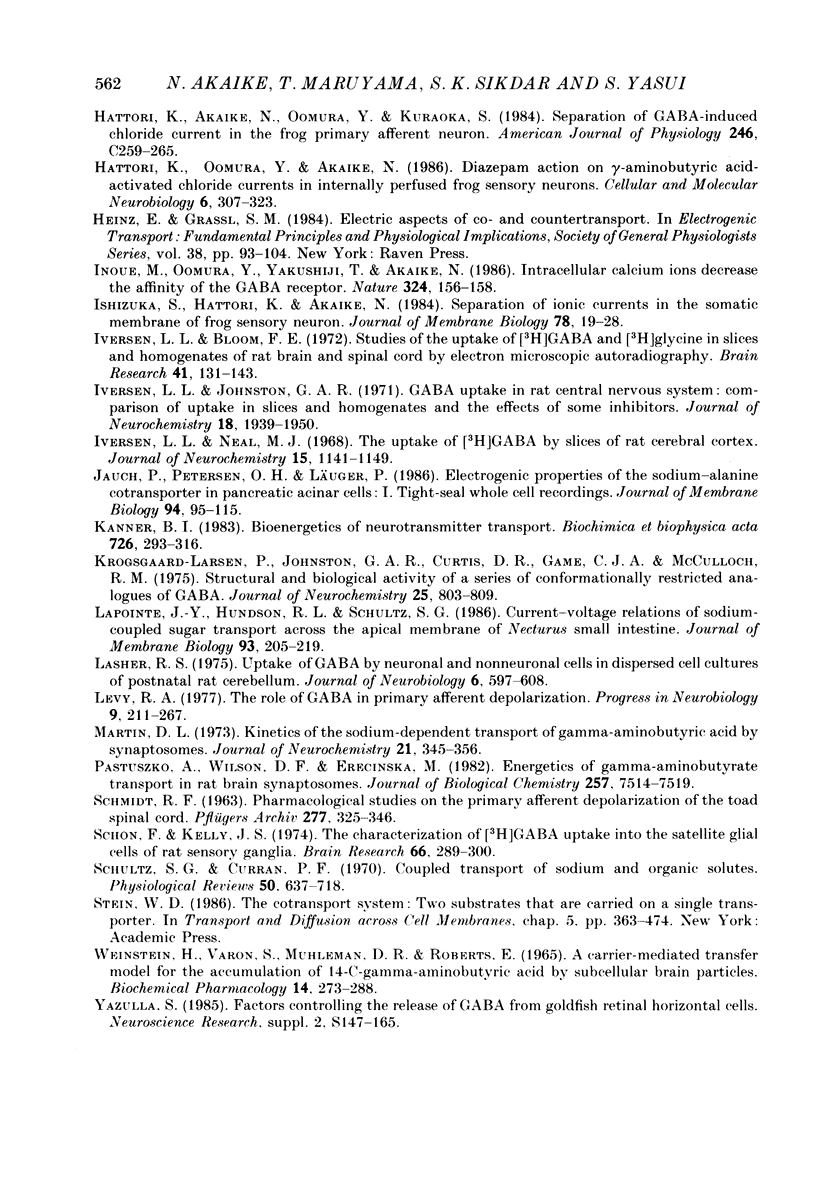
Selected References
These references are in PubMed. This may not be the complete list of references from this article.
- Akaike N., Hattori K., Inomata N., Oomura Y. gamma-Aminobutyric-acid- and pentobarbitone-gated chloride currents in internally perfused frog sensory neurones. J Physiol. 1985 Mar;360:367–386. doi: 10.1113/jphysiol.1985.sp015622. [DOI] [PMC free article] [PubMed] [Google Scholar]
- Akaike N., Hattori K., Oomura Y., Carpenter D. O. Bicuculline and picrotoxin block gamma-aminobutyric acid-gated Cl- conductance by different mechanisms. Experientia. 1985 Jan 15;41(1):70–71. doi: 10.1007/BF02005880. [DOI] [PubMed] [Google Scholar]
- Akaike N., Inoue M., Krishtal O. A. 'Concentration-clamp' study of gamma-aminobutyric-acid-induced chloride current kinetics in frog sensory neurones. J Physiol. 1986 Oct;379:171–185. doi: 10.1113/jphysiol.1986.sp016246. [DOI] [PMC free article] [PubMed] [Google Scholar]
- Beart P. M., Johnston G. A. GABA uptake in rat brain slices: inhibition by GABA analogues and by various drugs. J Neurochem. 1973 Feb;20(2):319–324. doi: 10.1111/j.1471-4159.1973.tb12131.x. [DOI] [PubMed] [Google Scholar]
- Beart P. M., Johnston G. A., Uhr M. L. Competitive inhibition of GABA uptake in rat brain slices by some GABA analogues of restricted conformation. J Neurochem. 1972 Aug;19(8):1855–1861. doi: 10.1111/j.1471-4159.1972.tb01474.x. [DOI] [PubMed] [Google Scholar]
- Bihler I., Adamic S. The effect of lithium on intestinal sugar transport. Biochim Biophys Acta. 1967 Jul 3;135(3):466–474. doi: 10.1016/0005-2736(67)90036-3. [DOI] [PubMed] [Google Scholar]
- Blaustein M. P., King A. C. Influence of membrane potential on the sodium-dependent uptake of gamma-aminobutyric acid by presynaptic nerve terminals: experimental observations and theoretical considerations. J Membr Biol. 1976 Dec 28;30(2):153–173. doi: 10.1007/BF01869665. [DOI] [PubMed] [Google Scholar]
- Carpenter D. O., Alving B. O. A contribution of an electrogenic Na+ pump to membrane potential in Aplysia neurons. J Gen Physiol. 1968 Jul;52(1):1–21. doi: 10.1085/jgp.52.1.1. [DOI] [PMC free article] [PubMed] [Google Scholar]
- Crane R. K. Na+ -dependent transport in the intestine and other animal tissues. Fed Proc. 1965 Sep-Oct;24(5):1000–1006. [PubMed] [Google Scholar]
- Davidoff R. A., Adair R. High-affinity uptake of (3H)gamma-aminobutyric acid into frog spinal cord slices. Brain Res. 1974 Aug 23;76(3):552–556. doi: 10.1016/0006-8993(74)90834-8. [DOI] [PubMed] [Google Scholar]
- Deitmer J. W., Ellis D. Changes in the intracellular sodium activity of sheep heart Purkinje fibres produced by calcium and other divalent cations. J Physiol. 1978 Apr;277:437–453. doi: 10.1113/jphysiol.1978.sp012283. [DOI] [PMC free article] [PubMed] [Google Scholar]
- ECCLES J. C., SCHMIDT R., WILLIS W. D. PHARMACOLOGICAL STUDIES ON PRESYNAPTIC INHIBITION. J Physiol. 1963 Oct;168:500–530. doi: 10.1113/jphysiol.1963.sp007205. [DOI] [PMC free article] [PubMed] [Google Scholar]
- FAUST R. G. The effect of anoxia and lithium ions on the absorption of D-glucose by the rat jejunum, in vitro. Biochim Biophys Acta. 1962 Jul 16;60:604–614. doi: 10.1016/0006-3002(62)90879-x. [DOI] [PubMed] [Google Scholar]
- Feltz P., Rasminsky M. A model for the mode of action of GABA on primary afferent terminals: depolarizing effects of GABA applied iontophoretically to neurones of mammalian dorsal root ganglia. Neuropharmacology. 1974 Jun;13(6):553–563. doi: 10.1016/0028-3908(74)90145-2. [DOI] [PubMed] [Google Scholar]
- Gallagher J. P., Higashi H., Nishi S. Characterization and ionic basis of GABA-induced depolarizations recorded in vitro from cat primary afferent neurones. J Physiol. 1978 Feb;275:263–282. doi: 10.1113/jphysiol.1978.sp012189. [DOI] [PMC free article] [PubMed] [Google Scholar]
- Gunter-Smith P. J., Grasset E., Schultz S. G. Sodium-coupled amino acid and sugar transport by Necturus small intestine. An equivalent electrical circuit analysis of a rheogenic co-transport system. J Membr Biol. 1982;66(1):25–39. doi: 10.1007/BF01868479. [DOI] [PubMed] [Google Scholar]
- Hattori K., Akaike N., Oomura Y., Kuraoka S. Internal perfusion studies demonstrating GABA-induced chloride responses in frog primary afferent neurons. Am J Physiol. 1984 Mar;246(3 Pt 1):C259–C265. doi: 10.1152/ajpcell.1984.246.3.C259. [DOI] [PubMed] [Google Scholar]
- Hattori K., Oomura Y., Akaike N. Diazepam action on gamma-aminobutyric acid-activated chloride currents in internally perfused frog sensory neurons. Cell Mol Neurobiol. 1986 Sep;6(3):307–323. doi: 10.1007/BF00711116. [DOI] [PubMed] [Google Scholar]
- Inoue M., Oomura Y., Yakushiji T., Akaike N. Intracellular calcium ions decrease the affinity of the GABA receptor. Nature. 1986 Nov 13;324(6093):156–158. doi: 10.1038/324156a0. [DOI] [PubMed] [Google Scholar]
- Ishizuka S., Hattori K., Akaike N. Separation of ionic currents in the somatic membrane of frog sensory neurons. J Membr Biol. 1984;78(1):19–28. doi: 10.1007/BF01872528. [DOI] [PubMed] [Google Scholar]
- Iversen L. L., Bloom F. E. Studies of the uptake of 3 H-gaba and ( 3 H)glycine in slices and homogenates of rat brain and spinal cord by electron microscopic autoradiography. Brain Res. 1972 Jun 8;41(1):131–143. doi: 10.1016/0006-8993(72)90621-x. [DOI] [PubMed] [Google Scholar]
- Iversen L. L., Johnston G. A. GABA uptake in rat central nervous system: comparison of uptake in slices and homogenates and the effects of some inhibitors. J Neurochem. 1971 Oct;18(10):1939–1950. doi: 10.1111/j.1471-4159.1971.tb09600.x. [DOI] [PubMed] [Google Scholar]
- Iversen L. L., Neal M. J. The uptake of [3H]GABA by slices of rat cerebral cortex. J Neurochem. 1968 Oct;15(10):1141–1149. doi: 10.1111/j.1471-4159.1968.tb06831.x. [DOI] [PubMed] [Google Scholar]
- Jauch P., Petersen O. H., Läuger P. Electrogenic properties of the sodium-alanine cotransporter in pancreatic acinar cells: I. Tight-seal whole-cell recordings. J Membr Biol. 1986;94(2):99–115. doi: 10.1007/BF01871191. [DOI] [PubMed] [Google Scholar]
- Kanner B. I. Bioenergetics of neurotransmitter transport. Biochim Biophys Acta. 1983 Dec 30;726(4):293–316. doi: 10.1016/0304-4173(83)90013-7. [DOI] [PubMed] [Google Scholar]
- Krogsgaard-Larsen P., Johnston G. A., Curtis D. R., Game C. J., McCulloch R. M. Structure and biological activity of a series of conformationally restricted analogues of GABA. J Neurochem. 1975 Dec;25(6):803–809. doi: 10.1111/j.1471-4159.1975.tb04411.x. [DOI] [PubMed] [Google Scholar]
- Lapointe J. Y., Hudson R. L., Schultz S. G. Current-voltage relations of sodium-coupled sugar transport across the apical membrane of Necturus small intestine. J Membr Biol. 1986;93(3):205–219. doi: 10.1007/BF01871175. [DOI] [PubMed] [Google Scholar]
- Lasher R. S. Uptake of GABA by neuronal and nonneuronal cells in dispersed cell cultures of postnatal rat cerebellum. J Neurobiol. 1975 Nov;6(6):597–608. doi: 10.1002/neu.480060606. [DOI] [PubMed] [Google Scholar]
- Levy R. A. The role of GABA in primary afferent depolarization. Prog Neurobiol. 1977;9(4):211–267. doi: 10.1016/0301-0082(77)90002-8. [DOI] [PubMed] [Google Scholar]
- Martin D. L. Kinetics of the sodium-dependent transport of gamma-aminobutyric acid by synaptosomes. J Neurochem. 1973 Aug;21(2):345–356. doi: 10.1111/j.1471-4159.1973.tb04255.x. [DOI] [PubMed] [Google Scholar]
- Pastuszko A., Wilson D. F., Erecinska M. Energetics of gamma-aminobutyrate transport in rat brain synaptosomes. J Biol Chem. 1982 Jul 10;257(13):7514–7519. [PubMed] [Google Scholar]
- SCHMIDT R. F. PHARMACOLOGICAL STUDIES ON THE PRIMARY AFFERENT DEPOLARIZATION OF THE TOAD SPINAL CORD. Pflugers Arch Gesamte Physiol Menschen Tiere. 1963 Jul 2;277:325–346. doi: 10.1007/BF00362515. [DOI] [PubMed] [Google Scholar]
- WEINSTEIN H., VARON S., MUHLEMAN D. R., ROBERTS E. A CARRIER-MEDIATED TRANSFER MODEL FOR THE ACCUMULATION OF 14-C-GAMMA-AMINOBUTYRIC ACID BY SUBCELLULAR BRAIN PARTICLES. Biochem Pharmacol. 1965 Mar;14:273–288. doi: 10.1016/0006-2952(65)90192-9. [DOI] [PubMed] [Google Scholar]
- Yazulla S. Factors controlling the release of GABA from goldfish retinal horizontal cells. Neurosci Res Suppl. 1985;2:S147–S165. doi: 10.1016/0921-8696(85)90014-3. [DOI] [PubMed] [Google Scholar]
- den Hertog A., Ritchie J. M. A comparison of the effect of temperature, metabolic inhibitors and of ouabain on the electrogenic componen of the sodium pump in mammalian non-myelinated nerve fibres. J Physiol. 1969 Oct;204(3):523–538. doi: 10.1113/jphysiol.1969.sp008929. [DOI] [PMC free article] [PubMed] [Google Scholar]