Abstract
The geometry and mechanical properties of the human erythrocyte membrane cytoskeleton are investigated by a computer simulation in which the cytoskeleton is represented by a network of polymer chains. Four elastic moduli as well as the area and thickness are predicted for the chain network as a function of temperature and the number of segments in each chain. Comparisons are made with mean field arguments to examine the importance of steric interactions in determining network properties. Applied to the red blood cell, the simulation predicts that in the bilayer plane the membrane cytoskeleton has a shear modulus of 10 +/- 2 x 10(-6) J/m2 and an areal compression modulus of 17 +/- 2 x 10(-6) J/m2. The volume compression modulus and the transverse Young's modulus of the cytoskeleton are predicted to be 1.2 +/- 0.1 x 10(3) J/m3 and 2.0 +/- 0.1 x 10(3) J/m3, respectively. Elements of the cytoskeleton are predicted to have a mean displacement from the bilayer plane of 15 nm. The simulation agrees with some, but not all, of the shear modulus measurements. The other predicted moduli have not been measured.
Full text
PDF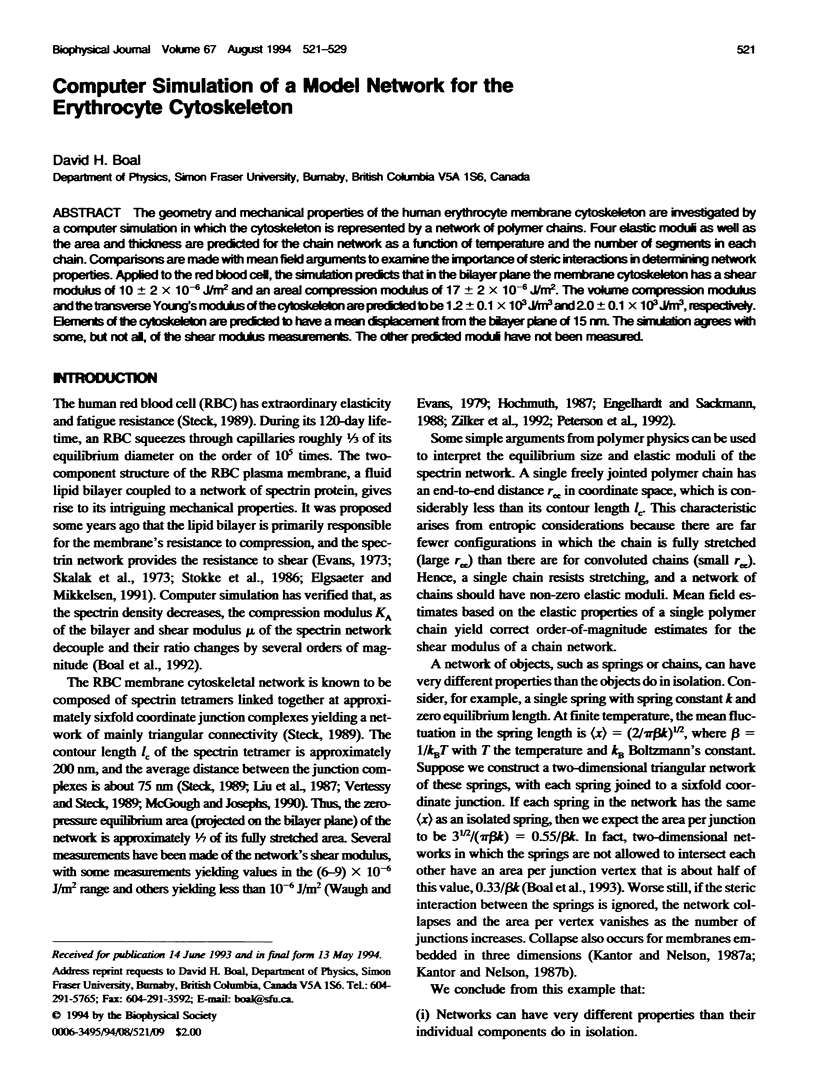
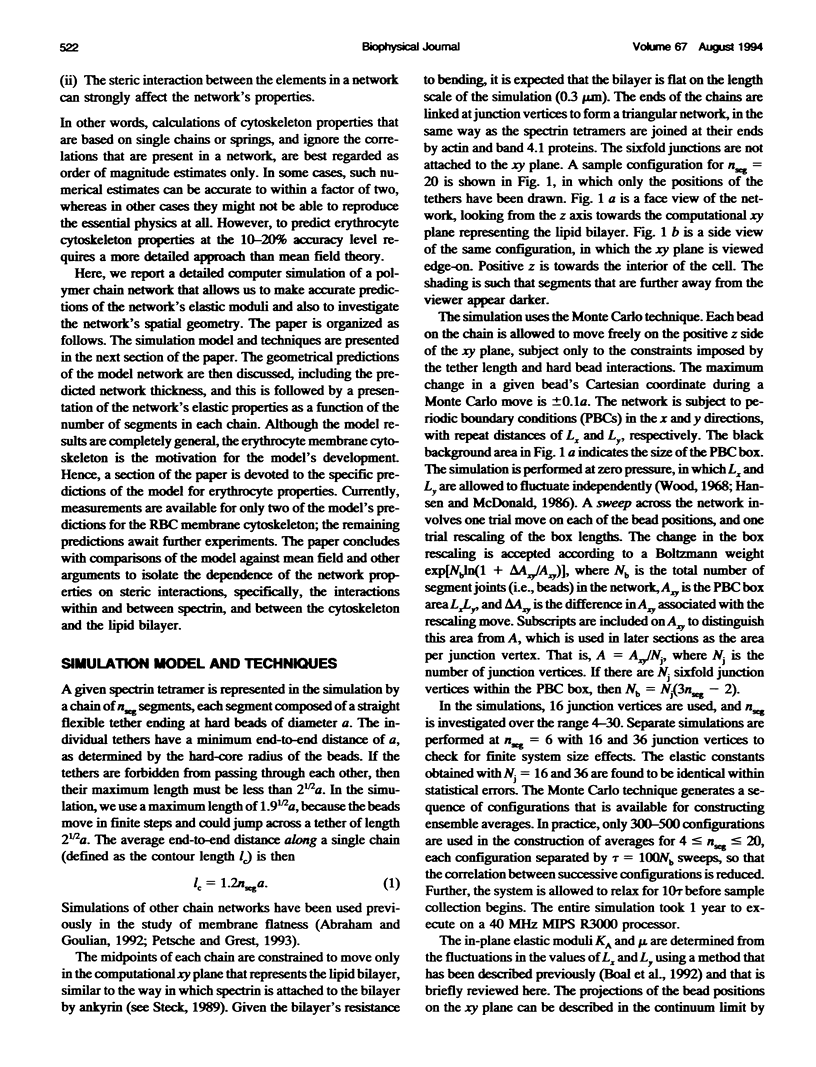
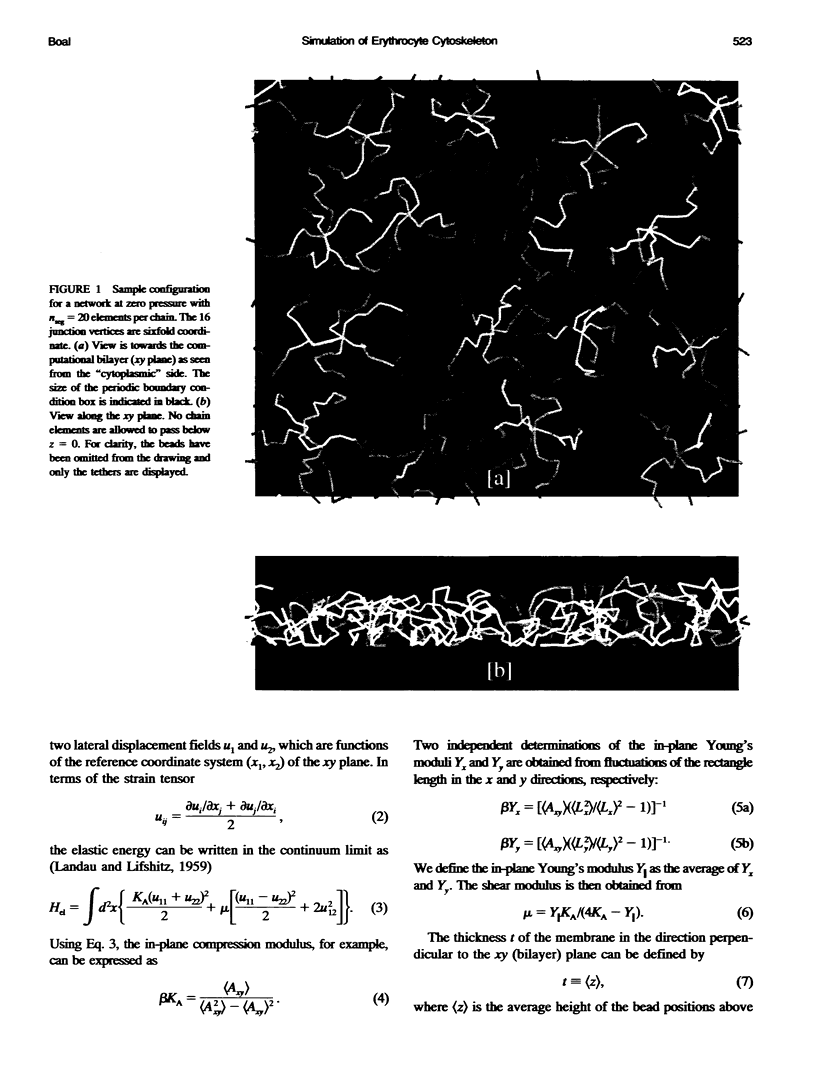
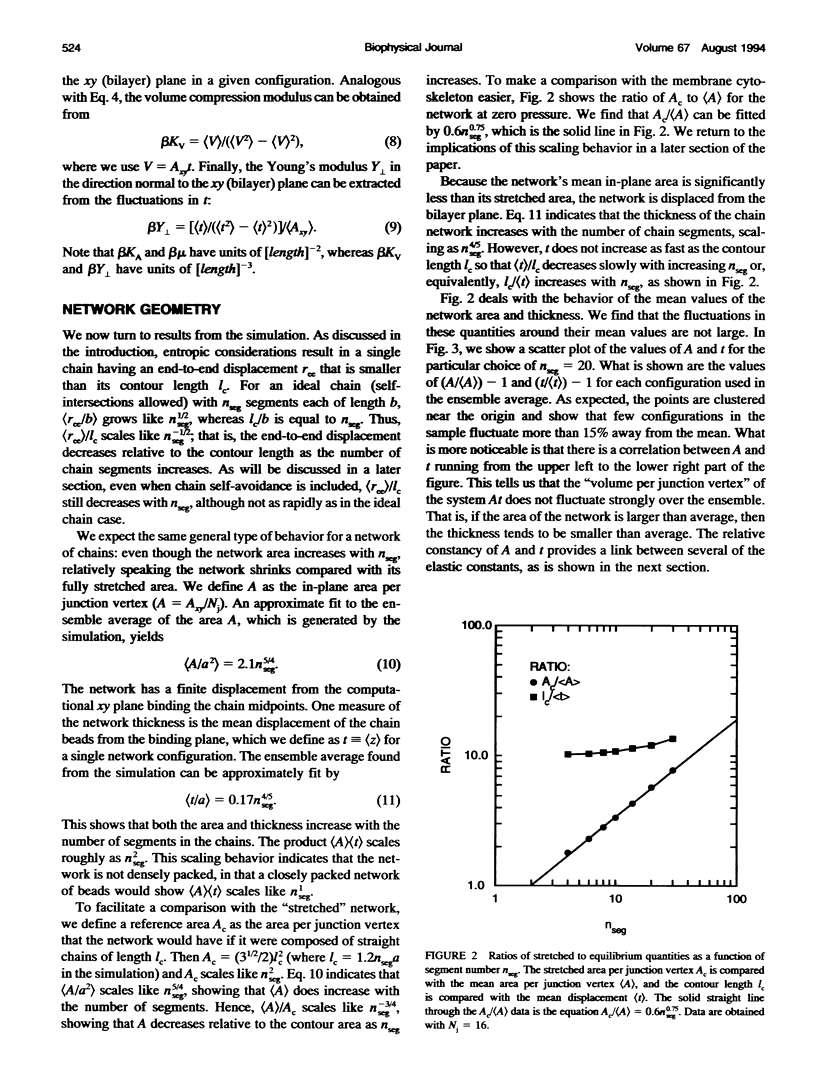
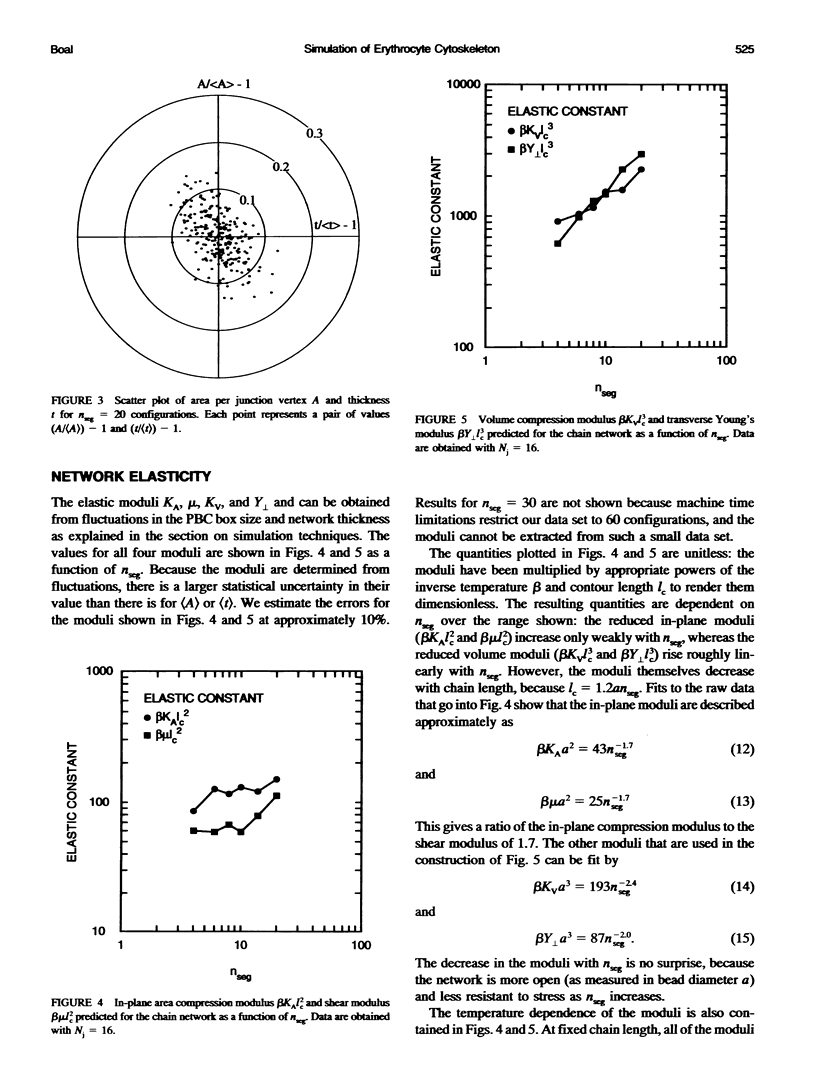
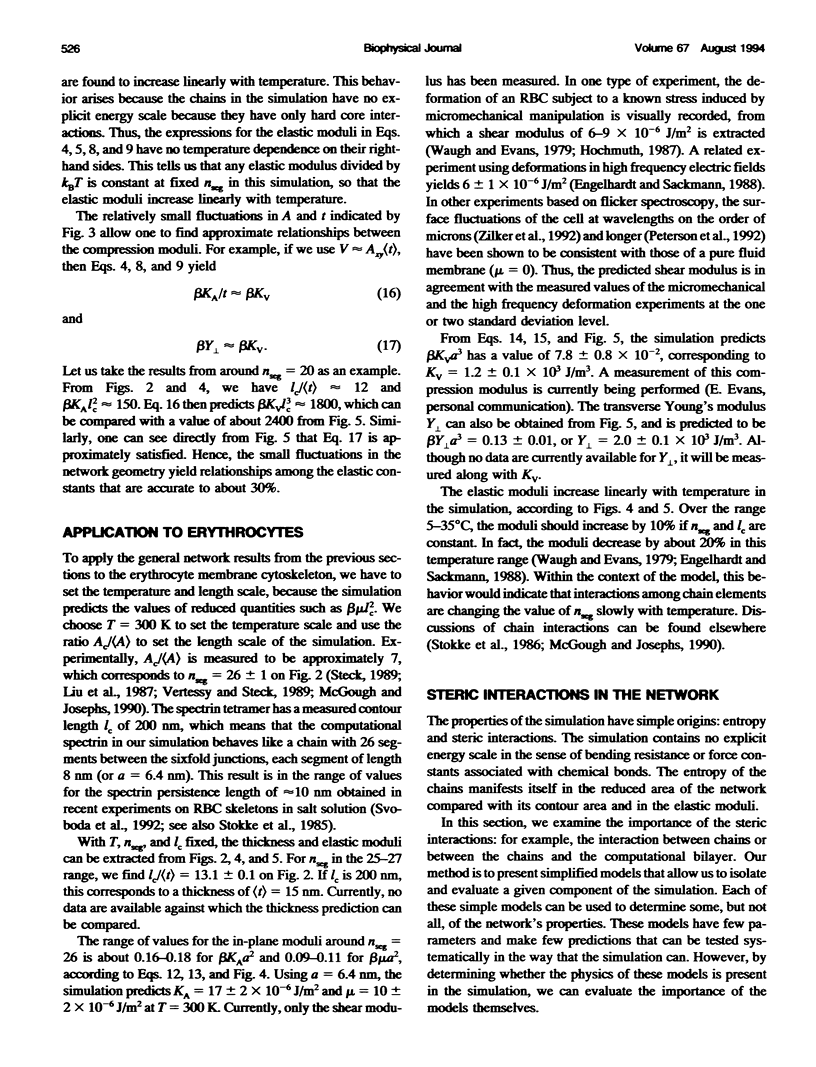
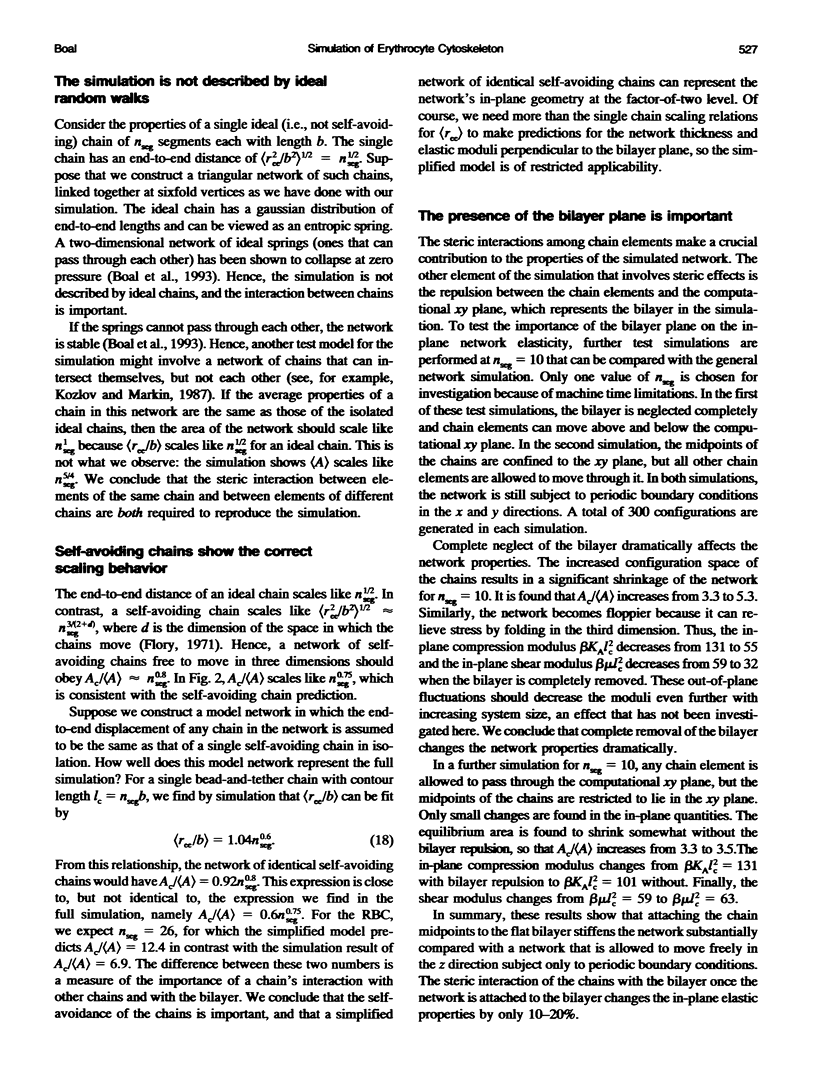
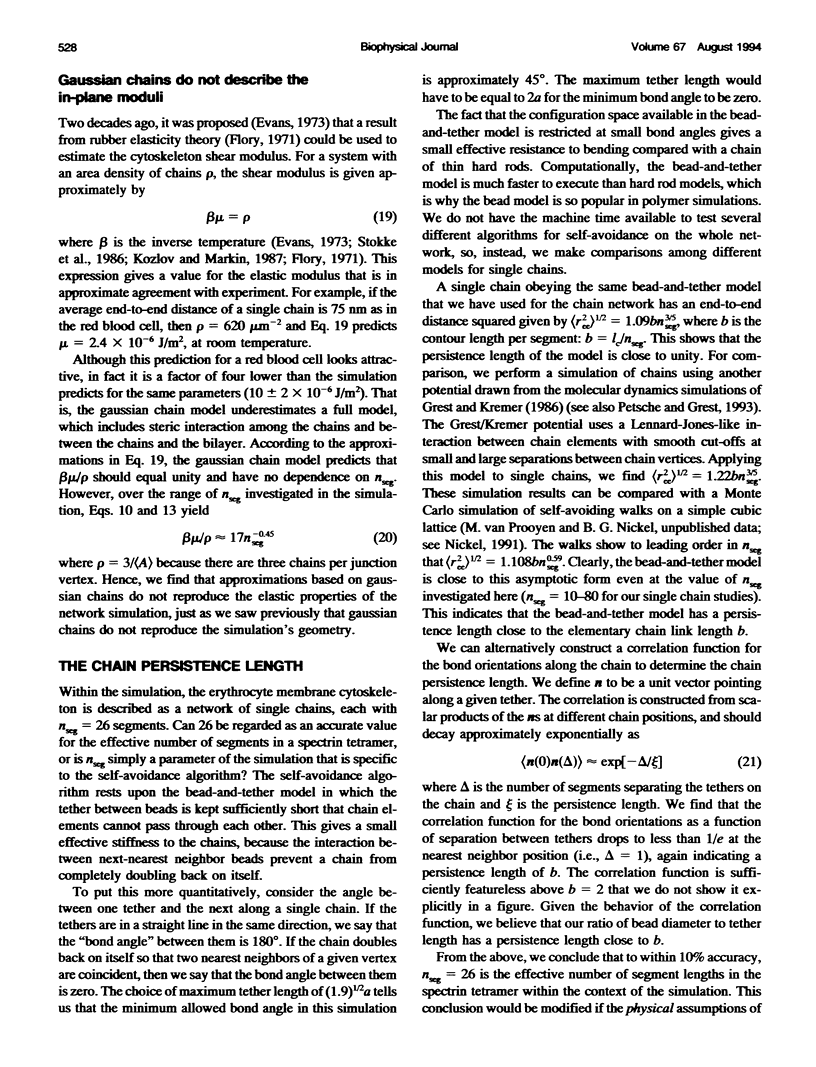
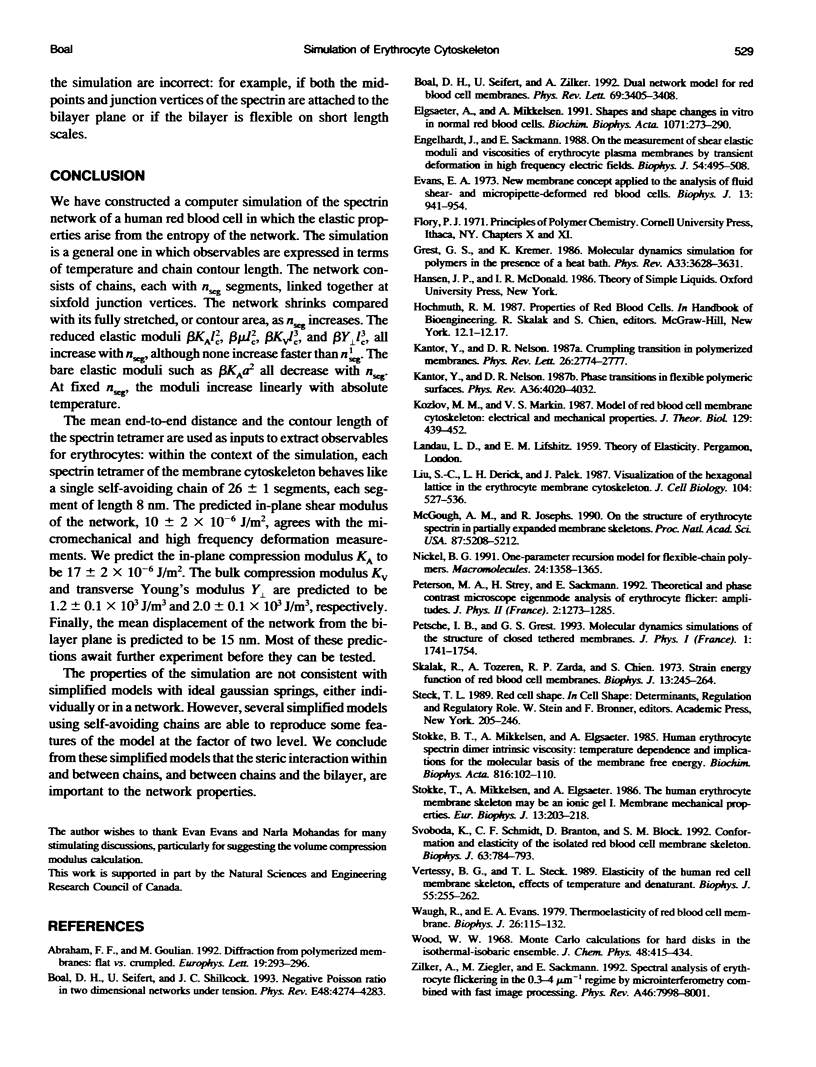
Images in this article
Selected References
These references are in PubMed. This may not be the complete list of references from this article.
- Boal DH, Seifert U, Shillcock JC. Negative Poisson ratio in two-dimensional networks under tension. Phys Rev E Stat Phys Plasmas Fluids Relat Interdiscip Topics. 1993 Dec;48(6):4274–4283. doi: 10.1103/physreve.48.4274. [DOI] [PubMed] [Google Scholar]
- Boal DH, Seifert U, Zilker A. Dual network model for red blood cell membranes. Phys Rev Lett. 1992 Dec 7;69(23):3405–3408. doi: 10.1103/PhysRevLett.69.3405. [DOI] [PubMed] [Google Scholar]
- Elgsaeter A., Mikkelsen A. Shapes and shape changes in vitro in normal red blood cells. Biochim Biophys Acta. 1991 Nov 13;1071(3):273–290. doi: 10.1016/0304-4157(91)90017-q. [DOI] [PubMed] [Google Scholar]
- Engelhardt H., Sackmann E. On the measurement of shear elastic moduli and viscosities of erythrocyte plasma membranes by transient deformation in high frequency electric fields. Biophys J. 1988 Sep;54(3):495–508. doi: 10.1016/S0006-3495(88)82982-5. [DOI] [PMC free article] [PubMed] [Google Scholar]
- Evans E. A. New membrane concept applied to the analysis of fluid shear- and micropipette-deformed red blood cells. Biophys J. 1973 Sep;13(9):941–954. doi: 10.1016/S0006-3495(73)86036-9. [DOI] [PMC free article] [PubMed] [Google Scholar]
- Grest GS, Kremer K. Molecular dynamics simulation for polymers in the presence of a heat bath. Phys Rev A Gen Phys. 1986 May;33(5):3628–3631. doi: 10.1103/physreva.33.3628. [DOI] [PubMed] [Google Scholar]
- Kozlov M. M., Markin V. S. Model of red blood cell membrane skeleton: electrical and mechanical properties. J Theor Biol. 1987 Dec 21;129(4):439–452. doi: 10.1016/s0022-5193(87)80023-1. [DOI] [PubMed] [Google Scholar]
- Liu S. C., Derick L. H., Palek J. Visualization of the hexagonal lattice in the erythrocyte membrane skeleton. J Cell Biol. 1987 Mar;104(3):527–536. doi: 10.1083/jcb.104.3.527. [DOI] [PMC free article] [PubMed] [Google Scholar]
- McGough A. M., Josephs R. On the structure of erythrocyte spectrin in partially expanded membrane skeletons. Proc Natl Acad Sci U S A. 1990 Jul;87(13):5208–5212. doi: 10.1073/pnas.87.13.5208. [DOI] [PMC free article] [PubMed] [Google Scholar]
- Skalak R., Tozeren A., Zarda R. P., Chien S. Strain energy function of red blood cell membranes. Biophys J. 1973 Mar;13(3):245–264. doi: 10.1016/S0006-3495(73)85983-1. [DOI] [PMC free article] [PubMed] [Google Scholar]
- Stokke B. T., Mikkelsen A., Elgsaeter A. Human erythrocyte spectrin dimer intrinsic viscosity: temperature dependence and implications for the molecular basis of the erythrocyte membrane free energy. Biochim Biophys Acta. 1985 Jun 11;816(1):102–110. doi: 10.1016/0005-2736(85)90398-0. [DOI] [PubMed] [Google Scholar]
- Svoboda K., Schmidt C. F., Branton D., Block S. M. Conformation and elasticity of the isolated red blood cell membrane skeleton. Biophys J. 1992 Sep;63(3):784–793. doi: 10.1016/S0006-3495(92)81644-2. [DOI] [PMC free article] [PubMed] [Google Scholar]
- Vertessy B. G., Steck T. L. Elasticity of the human red cell membrane skeleton. Effects of temperature and denaturants. Biophys J. 1989 Feb;55(2):255–262. doi: 10.1016/S0006-3495(89)82800-0. [DOI] [PMC free article] [PubMed] [Google Scholar]
- Waugh R., Evans E. A. Thermoelasticity of red blood cell membrane. Biophys J. 1979 Apr;26(1):115–131. doi: 10.1016/S0006-3495(79)85239-X. [DOI] [PMC free article] [PubMed] [Google Scholar]