Abstract
The spatial distribution of depolarized patches of membrane during the excitation of single neurons in culture has been recorded with a high spatial resolution (1 micron2/pixel) imaging system based on a liquid-nitrogen-cooled astronomical camera mounted on an inverted microscope. Images were captured from rat nodose neurons stained with the voltage-sensitive dye RH237. Conventional intracellular microelectrode recordings were made in synchrony with the images. During an action potential the fluorescence changes occurred in localized, unevenly distributed membrane areas, which formed clusters of depolarized sites of different sizes and intensities. When fast conductances were blocked by the addition of tetrodotoxin, a reduction in the number and the intensities of the depolarized sites was observed. The blockade by tetrodotoxin of voltage-clamped neurons also reduced the number of depolarized sites, although the same depolarizing voltage step was applied. Similarly, when a voltage-clamped neuron was depolarized by a constant-amplitude voltage step, the number of depolarized sites varied according to the degree of activation of the voltage-sensitive channels, which was modified by changing the holding potential. These results suggest that the spatial patterns of depolarization observed during excitation are related to the operations of ionic channels in the membrane.
Full text
PDF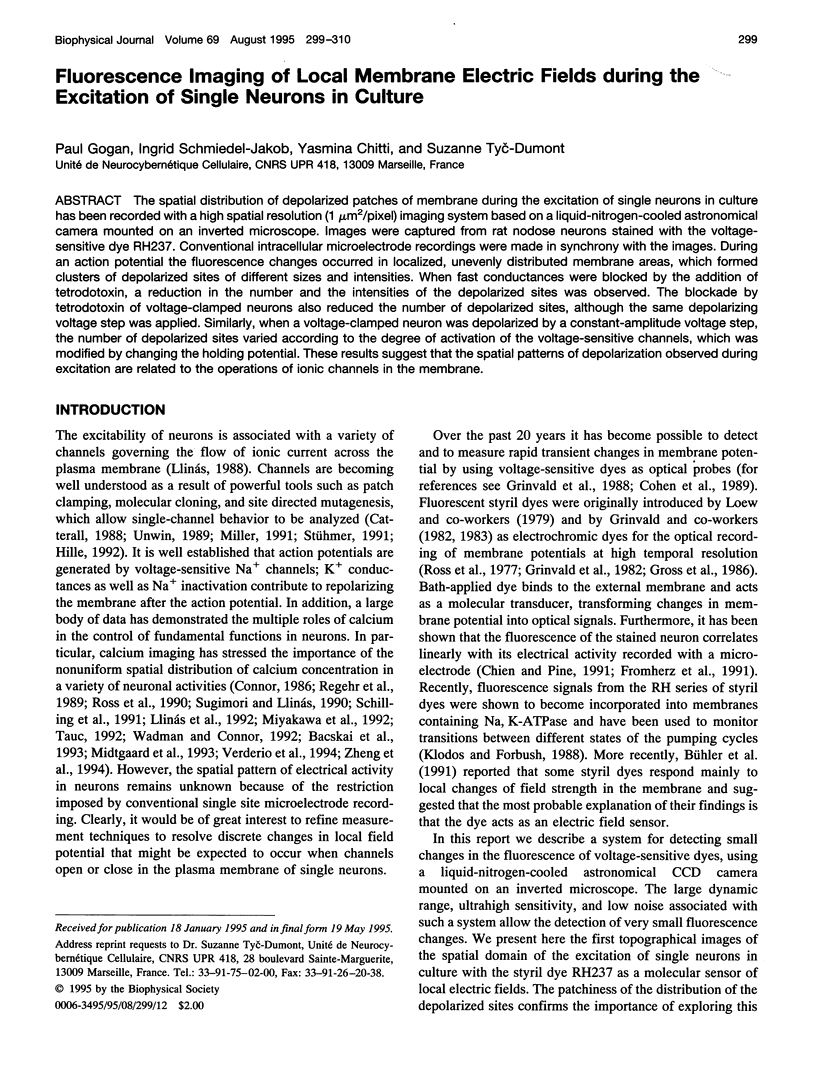
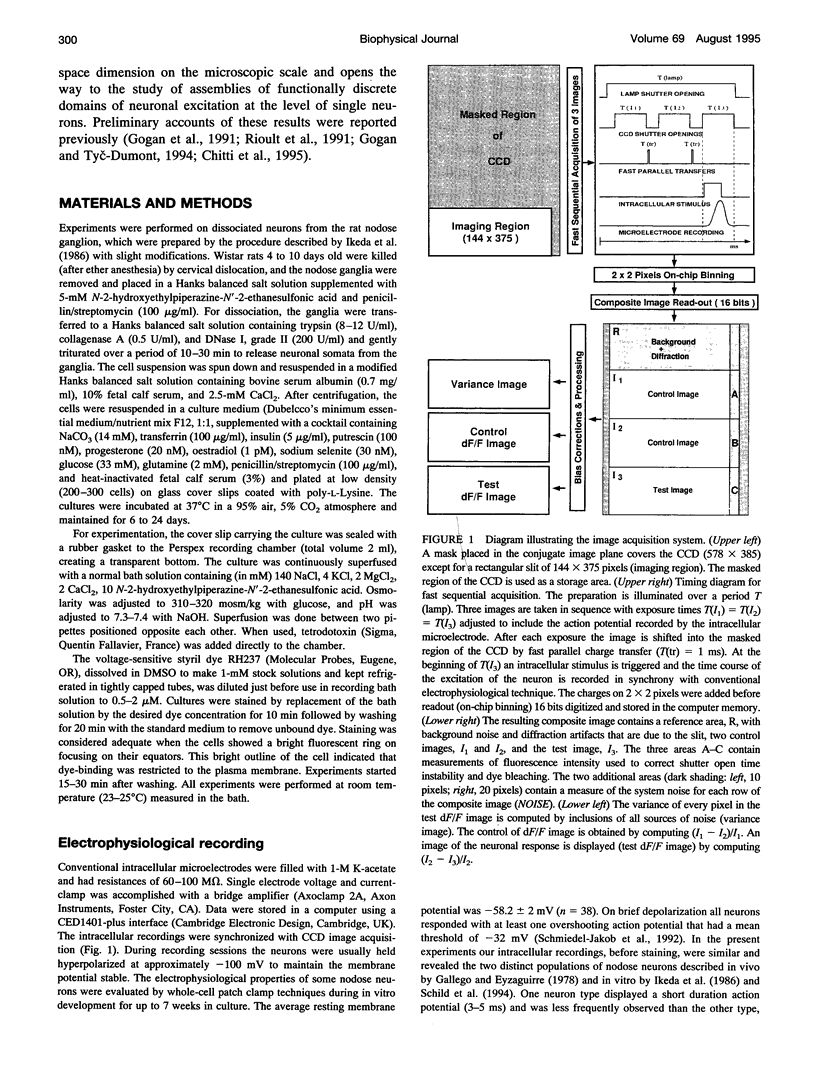
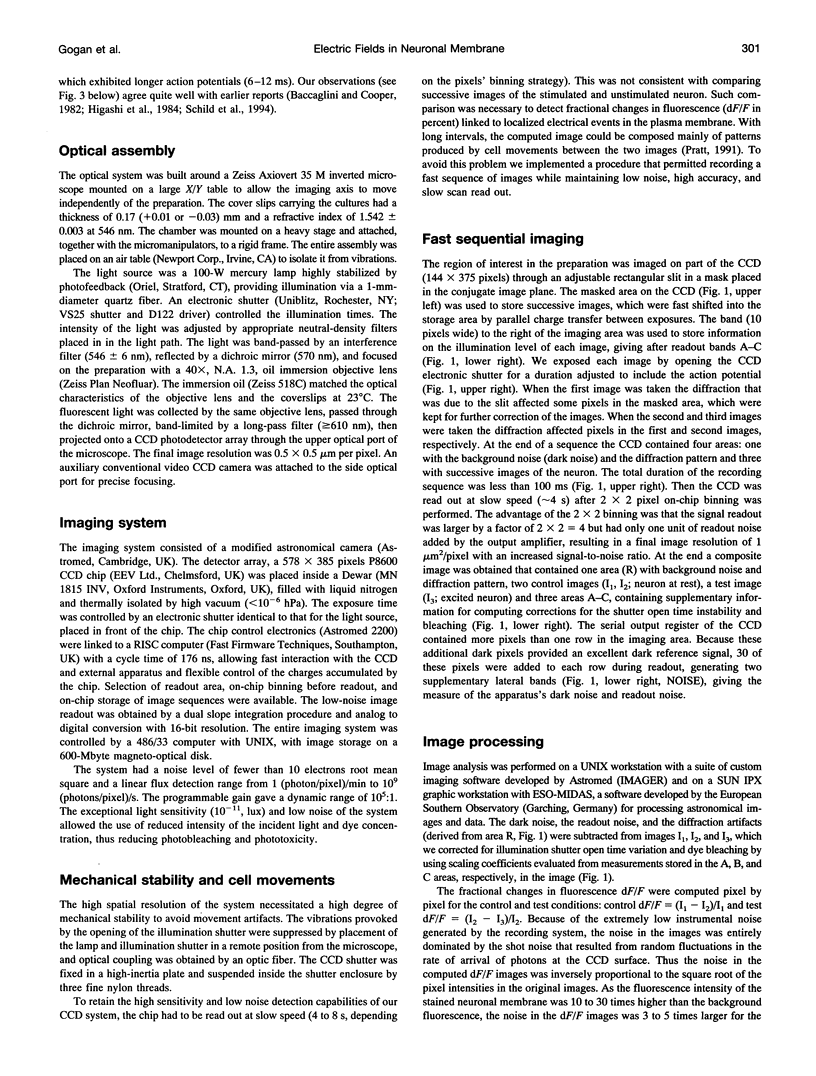
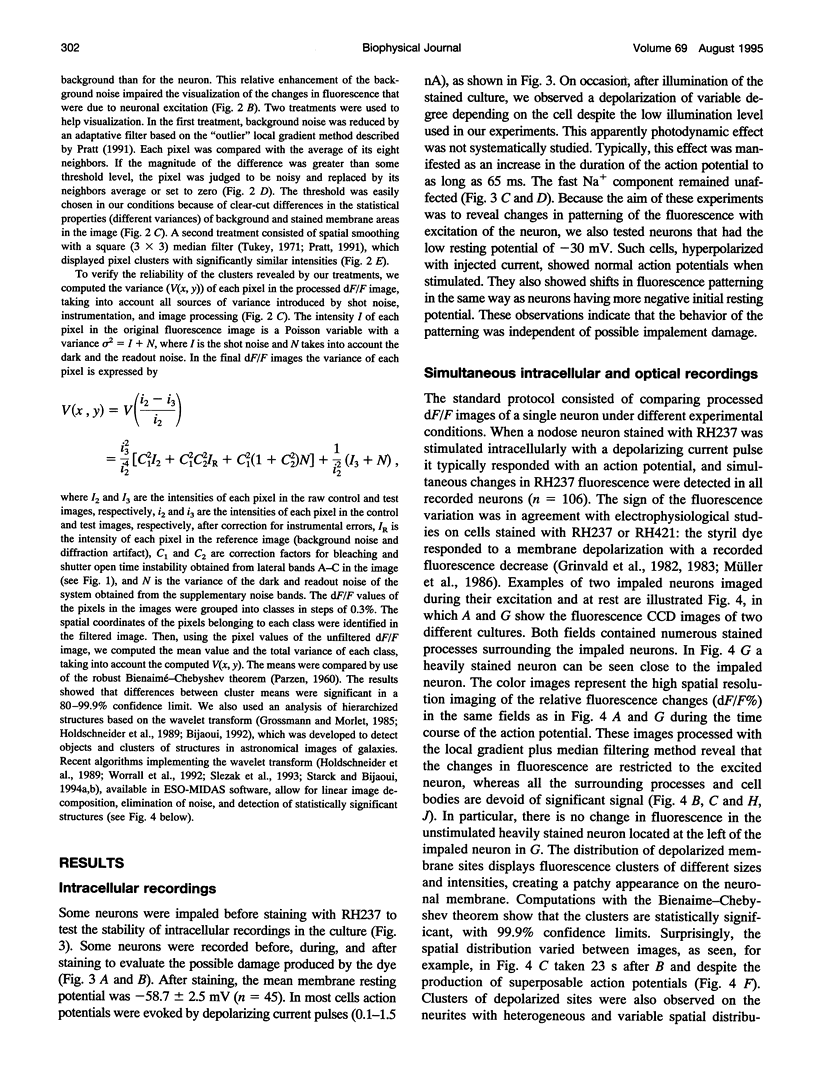
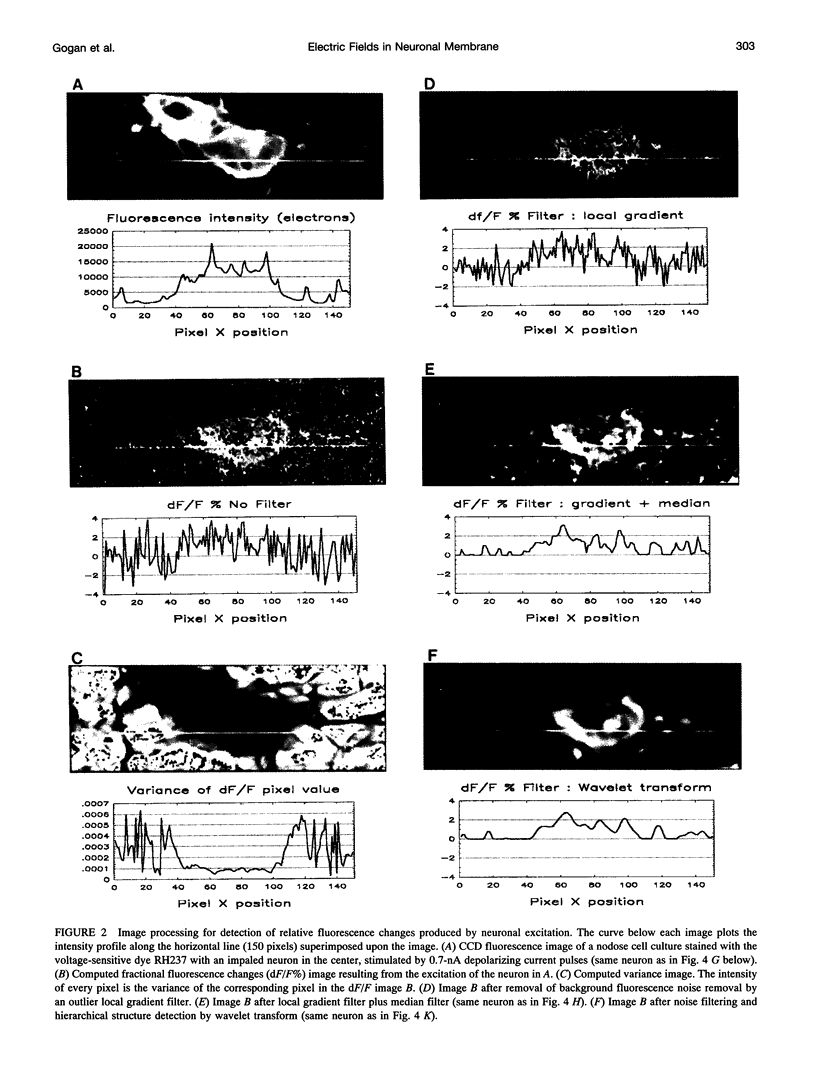
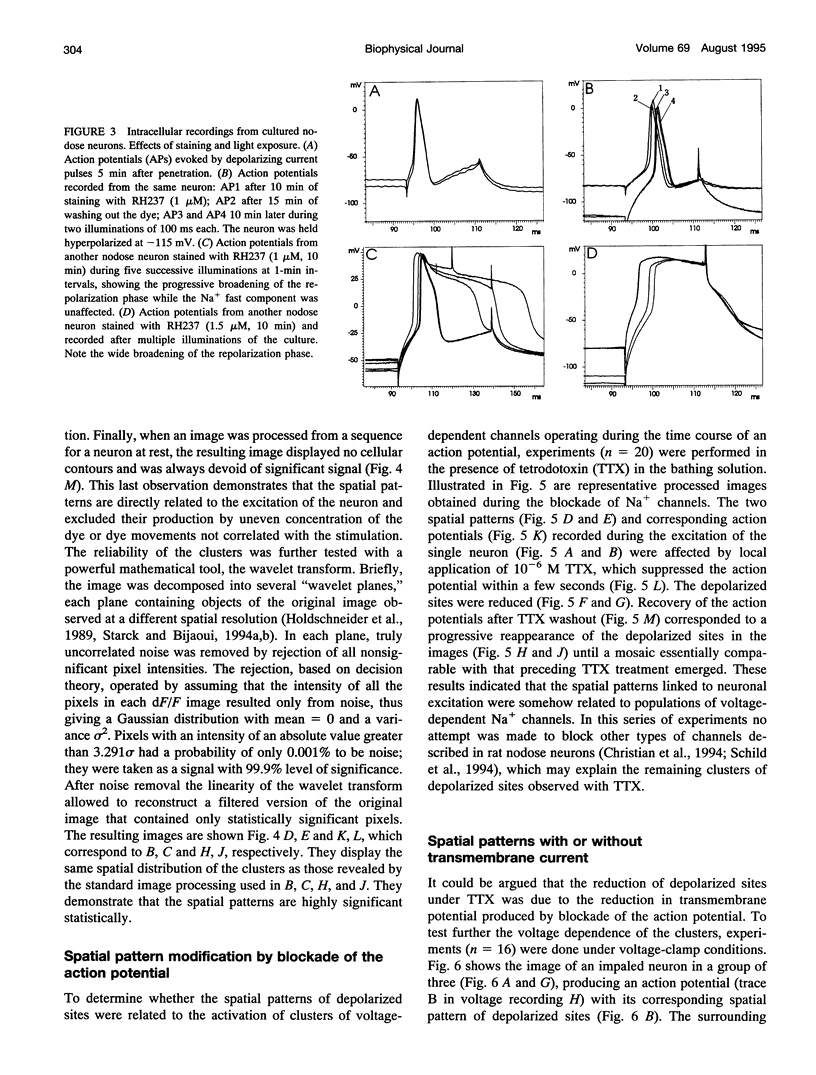
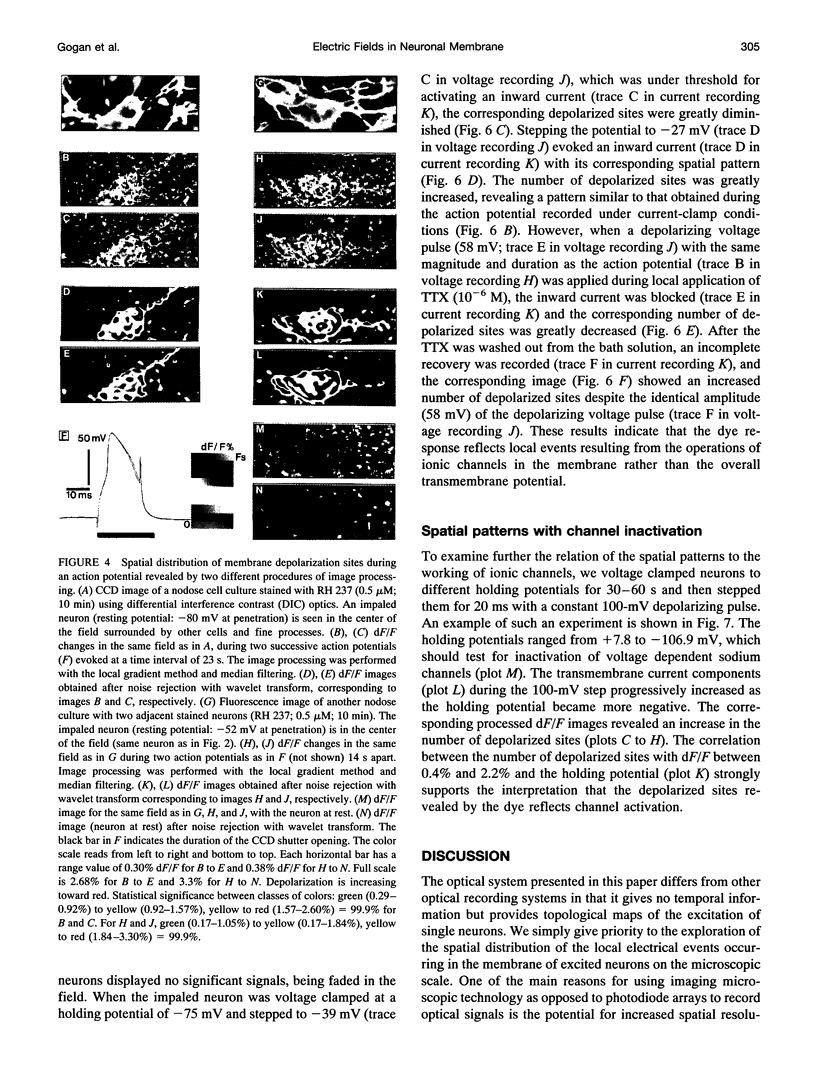
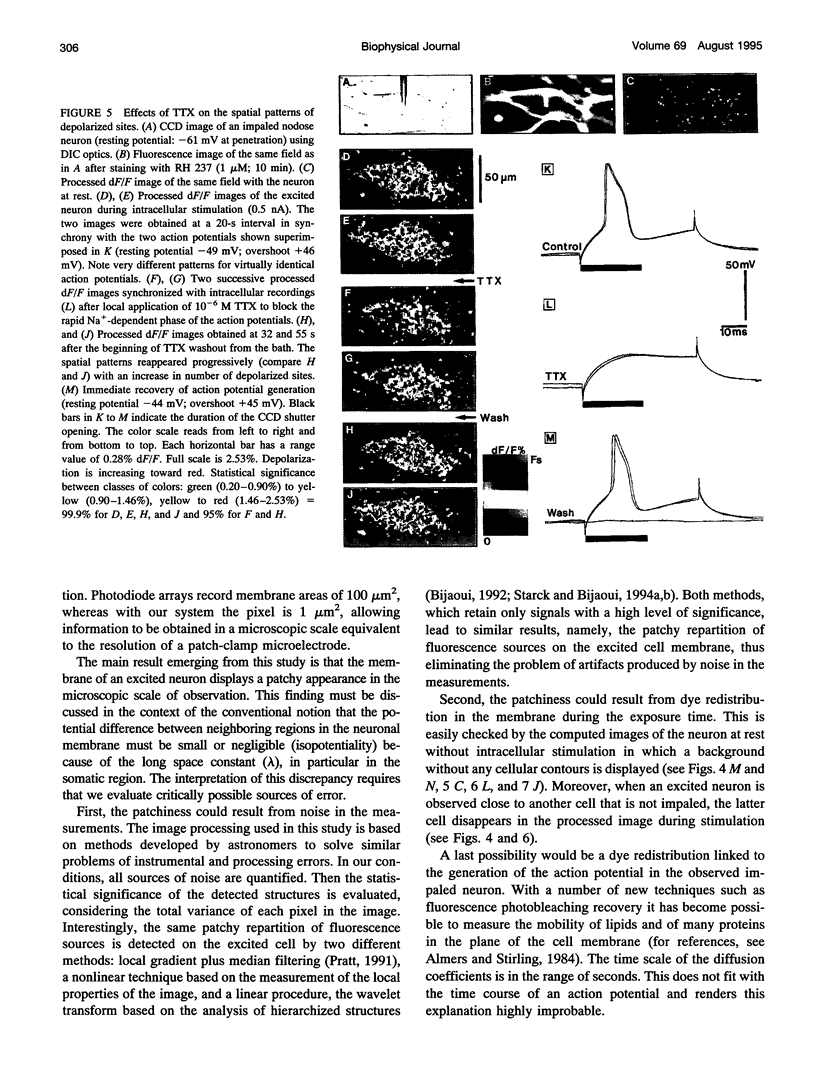
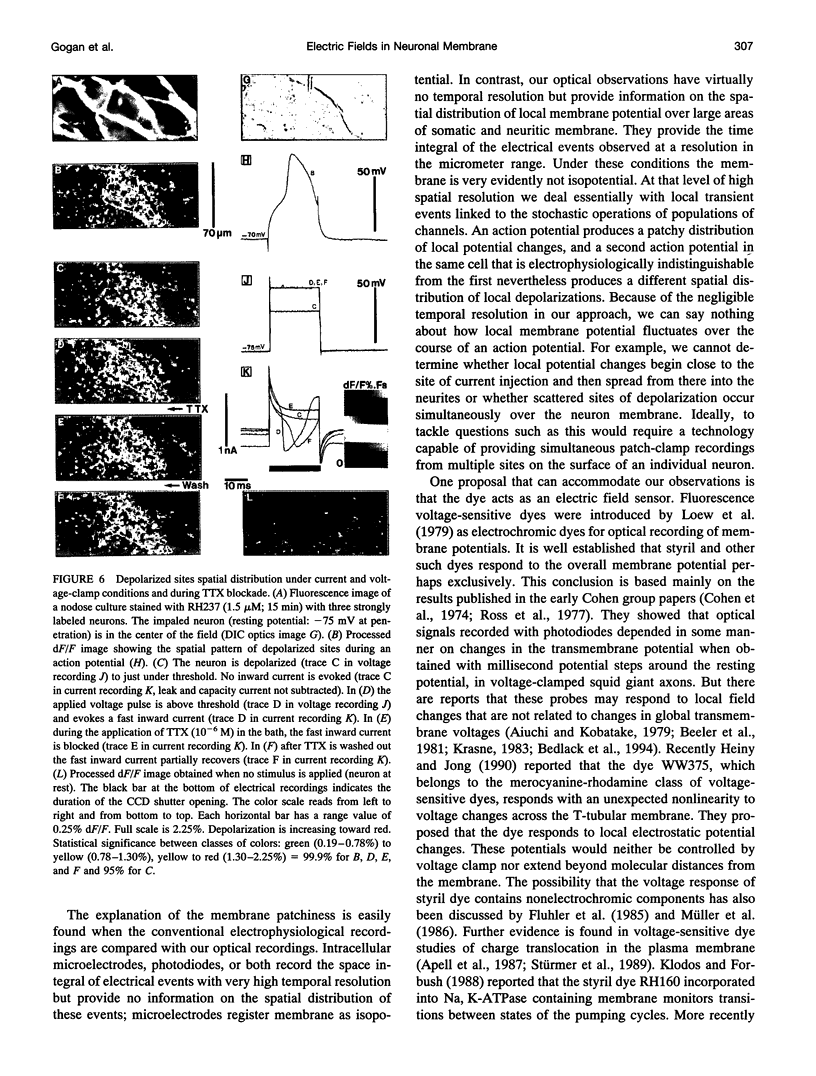
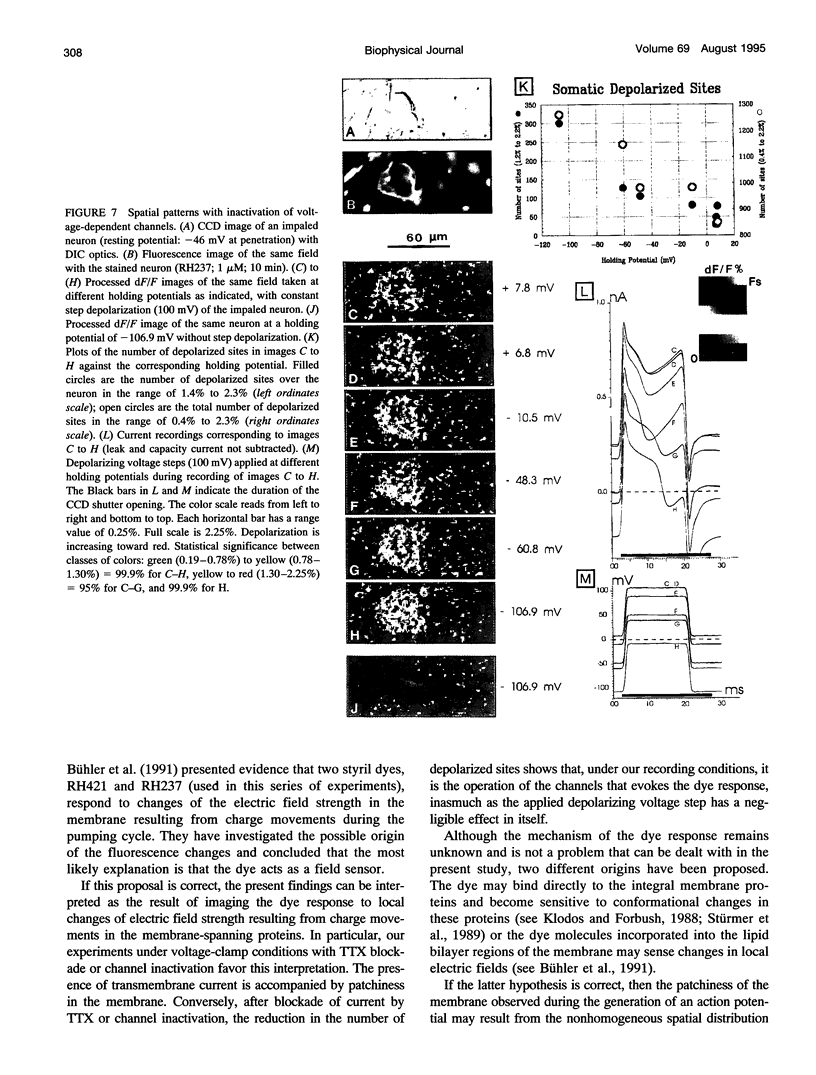
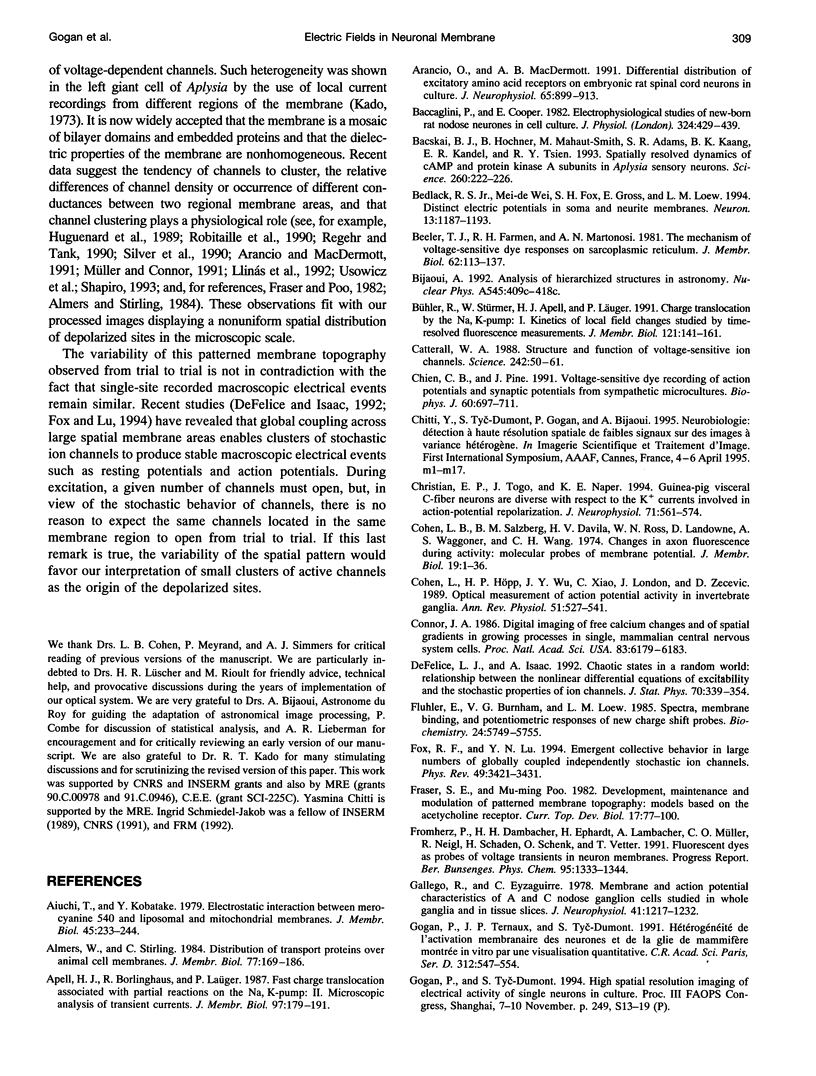
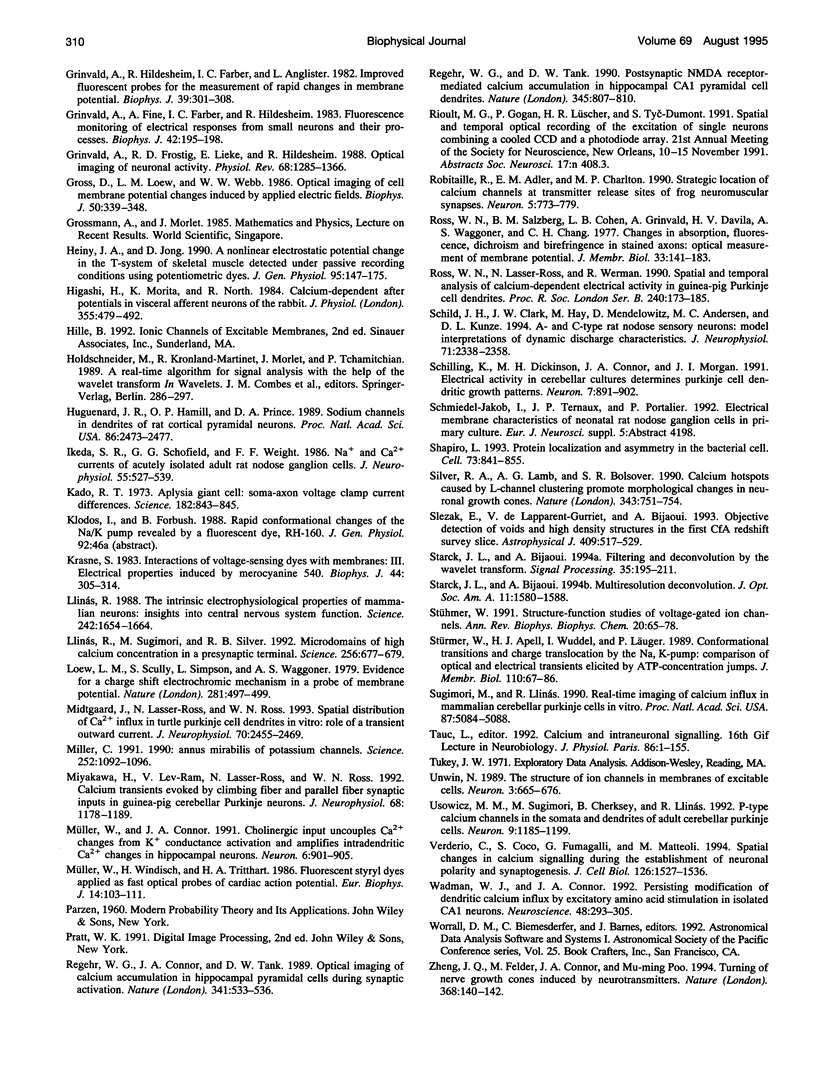
Images in this article
Selected References
These references are in PubMed. This may not be the complete list of references from this article.
- Aiuchi T., Kobatake Y. Electrostatic interaction between merocyanine 540 and liposomal and mitochondrial membranes. J Membr Biol. 1979 Apr 9;45(3-4):233–244. doi: 10.1007/BF01869287. [DOI] [PubMed] [Google Scholar]
- Almers W., Stirling C. Distribution of transport proteins over animal cell membranes. J Membr Biol. 1984;77(3):169–186. doi: 10.1007/BF01870567. [DOI] [PubMed] [Google Scholar]
- Apell H. J., Borlinghaus R., Läuger P. Fast charge translocations associated with partial reactions of the Na,K-pump: II. Microscopic analysis of transient currents. J Membr Biol. 1987;97(3):179–191. doi: 10.1007/BF01869221. [DOI] [PubMed] [Google Scholar]
- Arancio O., MacDermott A. B. Differential distribution of excitatory amino acid receptors on embryonic rat spinal cord neurons in culture. J Neurophysiol. 1991 Apr;65(4):899–913. doi: 10.1152/jn.1991.65.4.899. [DOI] [PubMed] [Google Scholar]
- Baccaglini P. I., Cooper E. Electrophysiological studies of new-born rat nodose neurones in cell culture. J Physiol. 1982 Mar;324:429–439. doi: 10.1113/jphysiol.1982.sp014122. [DOI] [PMC free article] [PubMed] [Google Scholar]
- Bacskai B. J., Hochner B., Mahaut-Smith M., Adams S. R., Kaang B. K., Kandel E. R., Tsien R. Y. Spatially resolved dynamics of cAMP and protein kinase A subunits in Aplysia sensory neurons. Science. 1993 Apr 9;260(5105):222–226. doi: 10.1126/science.7682336. [DOI] [PubMed] [Google Scholar]
- Bedlack R. S., Jr, Wei M. D., Fox S. H., Gross E., Loew L. M. Distinct electric potentials in soma and neurite membranes. Neuron. 1994 Nov;13(5):1187–1193. doi: 10.1016/0896-6273(94)90056-6. [DOI] [PubMed] [Google Scholar]
- Beeler T. J., Farmen R. H., Martonosi A. N. The mechanism of voltage-sensitive dye responses on sarcoplasmic reticulum. J Membr Biol. 1981;62(1-2):113–137. doi: 10.1007/BF01870205. [DOI] [PubMed] [Google Scholar]
- Bühler R., Stürmer W., Apell H. J., Läuger P. Charge translocation by the Na,K-pump: I. Kinetics of local field changes studied by time-resolved fluorescence measurements. J Membr Biol. 1991 Apr;121(2):141–161. doi: 10.1007/BF01870529. [DOI] [PubMed] [Google Scholar]
- Catterall W. A. Structure and function of voltage-sensitive ion channels. Science. 1988 Oct 7;242(4875):50–61. doi: 10.1126/science.2459775. [DOI] [PubMed] [Google Scholar]
- Chien C. B., Pine J. Voltage-sensitive dye recording of action potentials and synaptic potentials from sympathetic microcultures. Biophys J. 1991 Sep;60(3):697–711. doi: 10.1016/S0006-3495(91)82099-9. [DOI] [PMC free article] [PubMed] [Google Scholar]
- Christian E. P., Togo J., Naper K. E. Guinea pig visceral C-fiber neurons are diverse with respect to the K+ currents involved in action-potential repolarization. J Neurophysiol. 1994 Feb;71(2):561–574. doi: 10.1152/jn.1994.71.2.561. [DOI] [PubMed] [Google Scholar]
- Cohen L. B., Salzberg B. M., Davila H. V., Ross W. N., Landowne D., Waggoner A. S., Wang C. H. Changes in axon fluorescence during activity: molecular probes of membrane potential. J Membr Biol. 1974;19(1):1–36. doi: 10.1007/BF01869968. [DOI] [PubMed] [Google Scholar]
- Cohen L., Höpp H. P., Wu J. Y., Xiao C., London J. Optical measurement of action potential activity in invertebrate ganglia. Annu Rev Physiol. 1989;51:527–541. doi: 10.1146/annurev.ph.51.030189.002523. [DOI] [PubMed] [Google Scholar]
- Connor J. A. Digital imaging of free calcium changes and of spatial gradients in growing processes in single, mammalian central nervous system cells. Proc Natl Acad Sci U S A. 1986 Aug;83(16):6179–6183. doi: 10.1073/pnas.83.16.6179. [DOI] [PMC free article] [PubMed] [Google Scholar]
- Fluhler E., Burnham V. G., Loew L. M. Spectra, membrane binding, and potentiometric responses of new charge shift probes. Biochemistry. 1985 Oct 8;24(21):5749–5755. doi: 10.1021/bi00342a010. [DOI] [PubMed] [Google Scholar]
- Fox RF, Lu Yn. Emergent collective behavior in large numbers of globally coupled independently stochastic ion channels. Phys Rev E Stat Phys Plasmas Fluids Relat Interdiscip Topics. 1994 Apr;49(4):3421–3431. doi: 10.1103/physreve.49.3421. [DOI] [PubMed] [Google Scholar]
- Fraser S. E., Poo M. Development, maintenance, and modulation of patterned membrane topography: models based on the acetylcholine receptor. Curr Top Dev Biol. 1982;17(Pt 3):77–100. doi: 10.1016/s0070-2153(08)60519-0. [DOI] [PubMed] [Google Scholar]
- Gallego R., Eyzaguirre C. Membrane and action potential characteristics of A and C nodose ganglion cells studied in whole ganglia and in tissue slices. J Neurophysiol. 1978 Sep;41(5):1217–1232. doi: 10.1152/jn.1978.41.5.1217. [DOI] [PubMed] [Google Scholar]
- Gogan P., Ternaux J. P., Tyc-Dumont S. Visualisation quantitative de l'hétérogénéité de l'activation membranaire des neurones et de la glie de mammifère. C R Acad Sci III. 1991;312(11):547–554. [PubMed] [Google Scholar]
- Grinvald A., Fine A., Farber I. C., Hildesheim R. Fluorescence monitoring of electrical responses from small neurons and their processes. Biophys J. 1983 May;42(2):195–198. doi: 10.1016/S0006-3495(83)84386-0. [DOI] [PMC free article] [PubMed] [Google Scholar]
- Grinvald A., Frostig R. D., Lieke E., Hildesheim R. Optical imaging of neuronal activity. Physiol Rev. 1988 Oct;68(4):1285–1366. doi: 10.1152/physrev.1988.68.4.1285. [DOI] [PubMed] [Google Scholar]
- Grinvald A., Hildesheim R., Farber I. C., Anglister L. Improved fluorescent probes for the measurement of rapid changes in membrane potential. Biophys J. 1982 Sep;39(3):301–308. doi: 10.1016/S0006-3495(82)84520-7. [DOI] [PMC free article] [PubMed] [Google Scholar]
- Gross D., Loew L. M., Webb W. W. Optical imaging of cell membrane potential changes induced by applied electric fields. Biophys J. 1986 Aug;50(2):339–348. doi: 10.1016/S0006-3495(86)83467-1. [DOI] [PMC free article] [PubMed] [Google Scholar]
- Heiny J. A., Jong D. S. A nonlinear electrostatic potential change in the T-system of skeletal muscle detected under passive recording conditions using potentiometric dyes. J Gen Physiol. 1990 Jan;95(1):147–175. doi: 10.1085/jgp.95.1.147. [DOI] [PMC free article] [PubMed] [Google Scholar]
- Higashi H., Morita K., North R. A. Calcium-dependent after-potentials in visceral afferent neurones of the rabbit. J Physiol. 1984 Oct;355:479–492. doi: 10.1113/jphysiol.1984.sp015433. [DOI] [PMC free article] [PubMed] [Google Scholar]
- Huguenard J. R., Hamill O. P., Prince D. A. Sodium channels in dendrites of rat cortical pyramidal neurons. Proc Natl Acad Sci U S A. 1989 Apr;86(7):2473–2477. doi: 10.1073/pnas.86.7.2473. [DOI] [PMC free article] [PubMed] [Google Scholar]
- Ikeda S. R., Schofield G. G., Weight F. F. Na+ and Ca2+ currents of acutely isolated adult rat nodose ganglion cells. J Neurophysiol. 1986 Mar;55(3):527–539. doi: 10.1152/jn.1986.55.3.527. [DOI] [PubMed] [Google Scholar]
- Kado R. T. Aplysia giant cell: soma-axon voltage clamp current differences. Science. 1973 Nov 23;182(4114):843–845. doi: 10.1126/science.182.4114.843. [DOI] [PubMed] [Google Scholar]
- Krasne S. Interactions of voltage-sensing dyes with membranes. III. Electrical properties induced by merocyanine 540. Biophys J. 1983 Dec;44(3):305–314. doi: 10.1016/S0006-3495(83)84304-5. [DOI] [PMC free article] [PubMed] [Google Scholar]
- Llinás R. R. The intrinsic electrophysiological properties of mammalian neurons: insights into central nervous system function. Science. 1988 Dec 23;242(4886):1654–1664. doi: 10.1126/science.3059497. [DOI] [PubMed] [Google Scholar]
- Llinás R., Sugimori M., Silver R. B. Microdomains of high calcium concentration in a presynaptic terminal. Science. 1992 May 1;256(5057):677–679. doi: 10.1126/science.1350109. [DOI] [PubMed] [Google Scholar]
- Loew L. M., Scully S., Simpson L., Waggoner A. S. Evidence for a charge-shift electrochromic mechanism in a probe of membrane potential. Nature. 1979 Oct 11;281(5731):497–499. doi: 10.1038/281497a0. [DOI] [PubMed] [Google Scholar]
- Midtgaard J., Lasser-Ross N., Ross W. N. Spatial distribution of Ca2+ influx in turtle Purkinje cell dendrites in vitro: role of a transient outward current. J Neurophysiol. 1993 Dec;70(6):2455–2469. doi: 10.1152/jn.1993.70.6.2455. [DOI] [PubMed] [Google Scholar]
- Miyakawa H., Lev-Ram V., Lasser-Ross N., Ross W. N. Calcium transients evoked by climbing fiber and parallel fiber synaptic inputs in guinea pig cerebellar Purkinje neurons. J Neurophysiol. 1992 Oct;68(4):1178–1189. doi: 10.1152/jn.1992.68.4.1178. [DOI] [PubMed] [Google Scholar]
- Müller W., Connor J. A. Cholinergic input uncouples Ca2+ changes from K+ conductance activation and amplifies intradendritic Ca2+ changes in hippocampal neurons. Neuron. 1991 Jun;6(6):901–905. doi: 10.1016/0896-6273(91)90230-w. [DOI] [PubMed] [Google Scholar]
- Müller W., Windisch H., Tritthart H. A. Fluorescent styryl dyes applied as fast optical probes of cardiac action potential. Eur Biophys J. 1986;14(2):103–111. doi: 10.1007/BF00263067. [DOI] [PubMed] [Google Scholar]
- Regehr W. G., Connor J. A., Tank D. W. Optical imaging of calcium accumulation in hippocampal pyramidal cells during synaptic activation. Nature. 1989 Oct 12;341(6242):533–536. doi: 10.1038/341533a0. [DOI] [PubMed] [Google Scholar]
- Regehr W. G., Tank D. W. Postsynaptic NMDA receptor-mediated calcium accumulation in hippocampal CA1 pyramidal cell dendrites. Nature. 1990 Jun 28;345(6278):807–810. doi: 10.1038/345807a0. [DOI] [PubMed] [Google Scholar]
- Robitaille R., Adler E. M., Charlton M. P. Strategic location of calcium channels at transmitter release sites of frog neuromuscular synapses. Neuron. 1990 Dec;5(6):773–779. doi: 10.1016/0896-6273(90)90336-e. [DOI] [PubMed] [Google Scholar]
- Ross W. N., Lasser-Ross N., Werman R. Spatial and temporal analysis of calcium-dependent electrical activity in guinea pig Purkinje cell dendrites. Proc R Soc Lond B Biol Sci. 1990 May 22;240(1297):173–185. doi: 10.1098/rspb.1990.0032. [DOI] [PubMed] [Google Scholar]
- Ross W. N., Salzberg B. M., Cohen L. B., Grinvald A., Davila H. V., Waggoner A. S., Wang C. H. Changes in absorption, fluorescence, dichroism, and Birefringence in stained giant axons: : optical measurement of membrane potential. J Membr Biol. 1977 May 6;33(1-2):141–183. doi: 10.1007/BF01869514. [DOI] [PubMed] [Google Scholar]
- Schild J. H., Clark J. W., Hay M., Mendelowitz D., Andresen M. C., Kunze D. L. A- and C-type rat nodose sensory neurons: model interpretations of dynamic discharge characteristics. J Neurophysiol. 1994 Jun;71(6):2338–2358. doi: 10.1152/jn.1994.71.6.2338. [DOI] [PubMed] [Google Scholar]
- Schilling K., Dickinson M. H., Connor J. A., Morgan J. I. Electrical activity in cerebellar cultures determines Purkinje cell dendritic growth patterns. Neuron. 1991 Dec;7(6):891–902. doi: 10.1016/0896-6273(91)90335-w. [DOI] [PubMed] [Google Scholar]
- Shapiro L. Protein localization and asymmetry in the bacterial cell. Cell. 1993 Jun 4;73(5):841–855. doi: 10.1016/0092-8674(93)90266-s. [DOI] [PubMed] [Google Scholar]
- Silver R. A., Lamb A. G., Bolsover S. R. Calcium hotspots caused by L-channel clustering promote morphological changes in neuronal growth cones. Nature. 1990 Feb 22;343(6260):751–754. doi: 10.1038/343751a0. [DOI] [PubMed] [Google Scholar]
- Stühmer W. Structure-function studies of voltage-gated ion channels. Annu Rev Biophys Biophys Chem. 1991;20:65–78. doi: 10.1146/annurev.bb.20.060191.000433. [DOI] [PubMed] [Google Scholar]
- Stürmer W., Apell H. J., Wuddel I., Läuger P. Conformational transitions and change translocation by the Na,K pump: comparison of optical and electrical transients elicited by ATP-concentration jumps. J Membr Biol. 1989 Aug;110(1):67–86. doi: 10.1007/BF01870994. [DOI] [PubMed] [Google Scholar]
- Sugimori M., Llinás R. R. Real-time imaging of calcium influx in mammalian cerebellar Purkinje cells in vitro. Proc Natl Acad Sci U S A. 1990 Jul;87(13):5084–5088. doi: 10.1073/pnas.87.13.5084. [DOI] [PMC free article] [PubMed] [Google Scholar]
- Unwin N. The structure of ion channels in membranes of excitable cells. Neuron. 1989 Dec;3(6):665–676. doi: 10.1016/0896-6273(89)90235-3. [DOI] [PubMed] [Google Scholar]
- Usowicz M. M., Sugimori M., Cherksey B., Llinás R. P-type calcium channels in the somata and dendrites of adult cerebellar Purkinje cells. Neuron. 1992 Dec;9(6):1185–1199. doi: 10.1016/0896-6273(92)90076-p. [DOI] [PubMed] [Google Scholar]
- Verderio C., Coco S., Fumagalli G., Matteoli M. Spatial changes in calcium signaling during the establishment of neuronal polarity and synaptogenesis. J Cell Biol. 1994 Sep;126(6):1527–1536. doi: 10.1083/jcb.126.6.1527. [DOI] [PMC free article] [PubMed] [Google Scholar]
- Wadman W. J., Connor J. A. Persisting modification of dendritic calcium influx by excitatory amino acid stimulation in isolated Ca1 neurons. Neuroscience. 1992;48(2):293–305. doi: 10.1016/0306-4522(92)90491-j. [DOI] [PubMed] [Google Scholar]
- Zheng J. Q., Felder M., Connor J. A., Poo M. M. Turning of nerve growth cones induced by neurotransmitters. Nature. 1994 Mar 10;368(6467):140–144. doi: 10.1038/368140a0. [DOI] [PubMed] [Google Scholar]