Abstract
1. The aerobic accumulation of various monosaccharides in slices of rabbit kidney cortex at 25° was studied. 2. d-Fructose and α-methyl d-glucoside were readily accumulated against their concentration gradient by a phlorrhizin-sensitive Na+-dependent active transport. In the absence of external Na+ the maximal rate of α-methyl glucoside transport was decreased tenfold, the Km of entry into the cells (8·2mm) not being affected. Phlorrhizin and d-galactose inhibited the entry of α-methyl glucoside also in the absence of external Na+. 3. d-Xylose, 6-deoxy-d-glucose and 6-deoxy-d-galactose were poorly accumulated ([S]i/[S]o ratios slightly above 1·0); this transport was inhibited by phlorrhizin and by the absence of Na+. 4. 3-O-Methyl-d-glucose, d-arabinose and l-arabinose were not actively transported, [S]i/[S]o ratios never exceeding 1·0. 5. 2-Deoxy-d-glucose and 2-deoxy-d-galactose were readily accumulated against a high concentration gradient, this transport being Na+-independent and only slightly sensitive to phlorrhizin. External Na+ was not required for an inhibitory action of phlorrhizin and d-galactose on the entry of 2-deoxy-d-galactose into the cells. 6. Interference for entry into the cells between the following saccharides was found: d-galactose inhibited α-methyl d-glucoside transport; d-xylose entry was inhibited by d-glucose; d-galactose transport was inhibited by d-xylose; a mutual interference between d-galactose and its 2-deoxy analogue was found. 7. It is concluded that d-glucose, d-galactose, α-methyl d-glucoside, d-xylose and possibly also some other monosaccharides share a common active transport system. 8. The specificity of the Na+-dependent phlorrhizin-sensitive active transport system for monosaccharides in kidney-cortex cells differs from that in intestinal epithelial cells.
Full text
PDF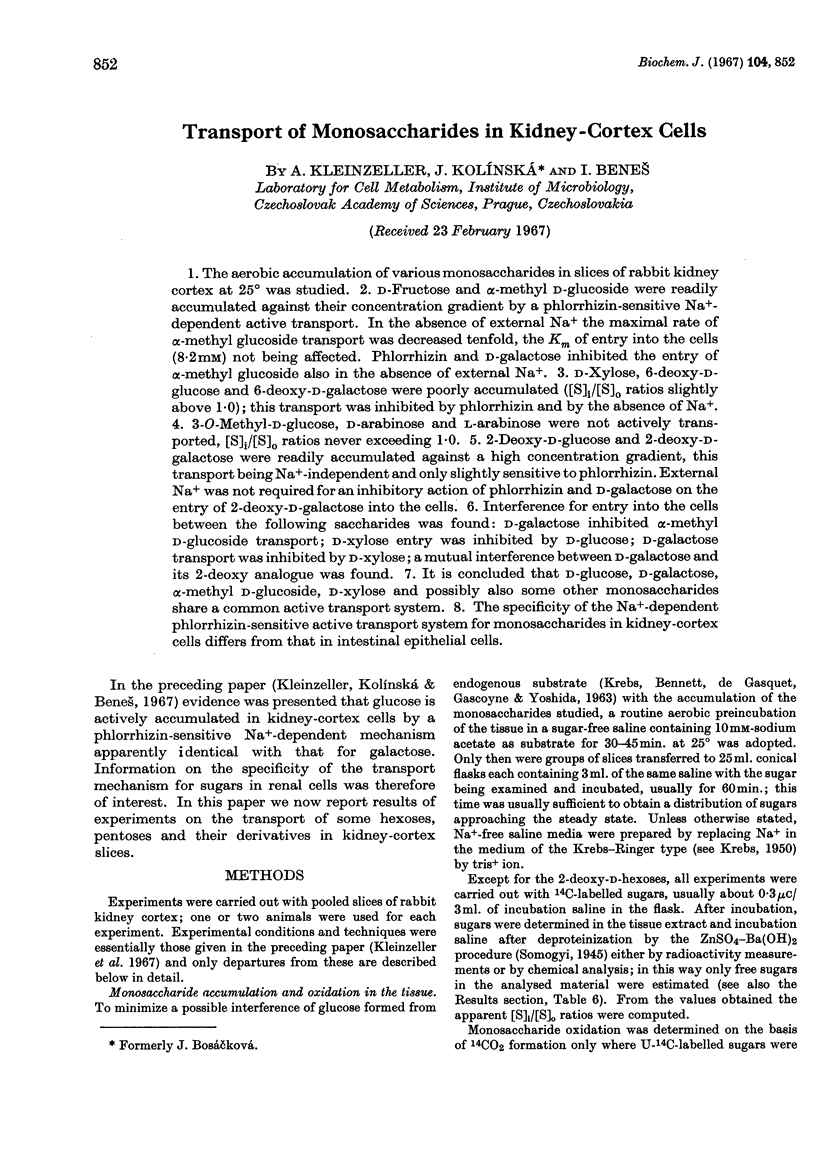
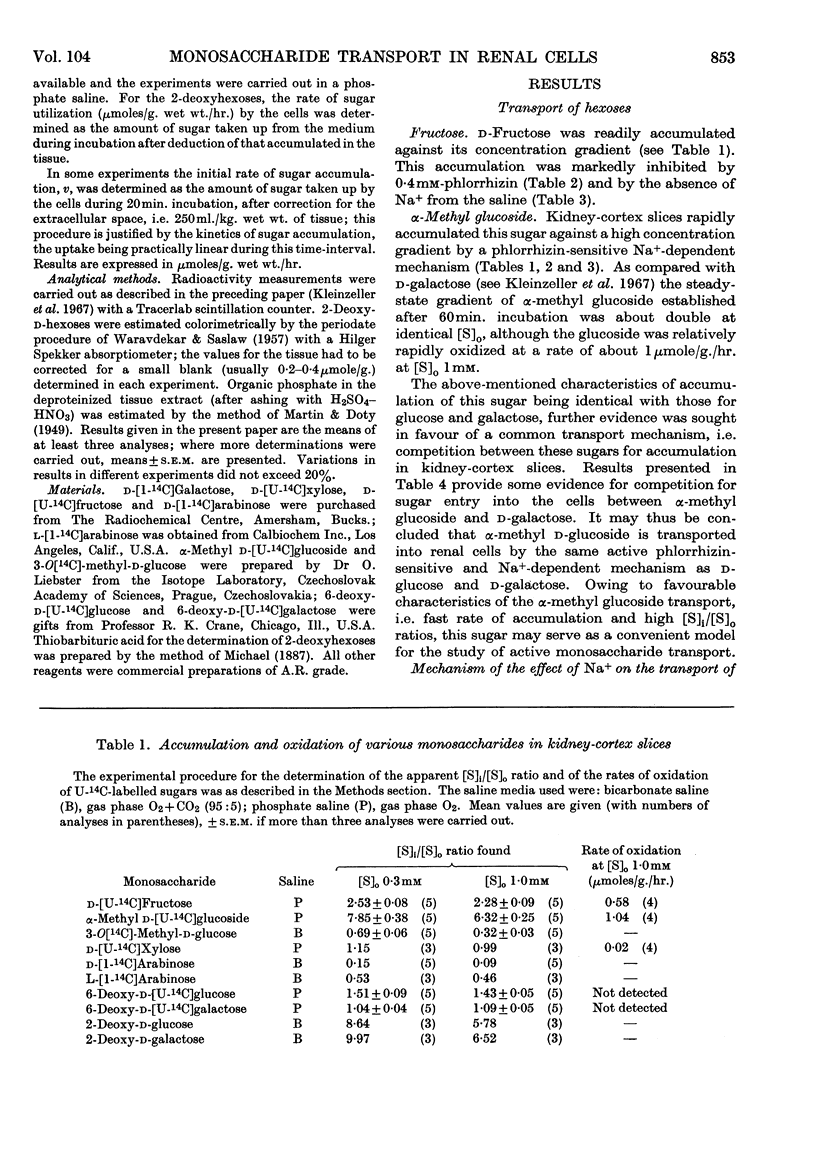
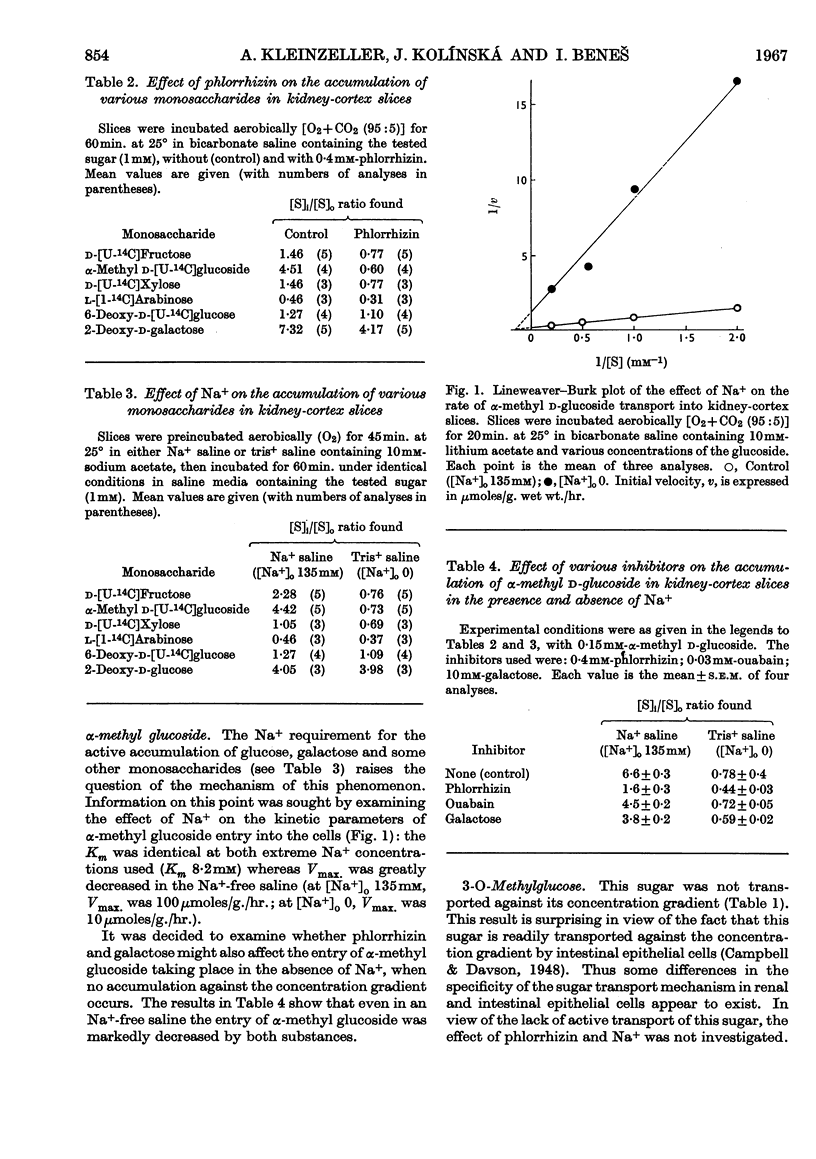
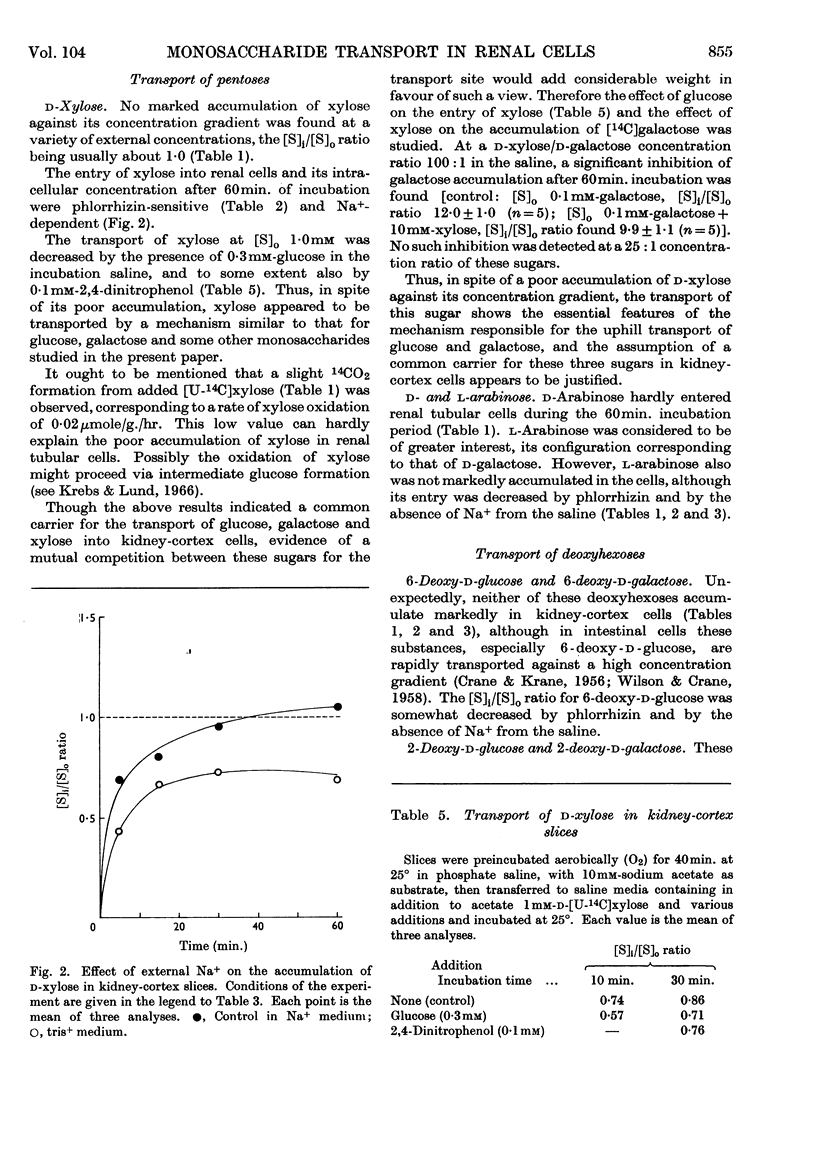
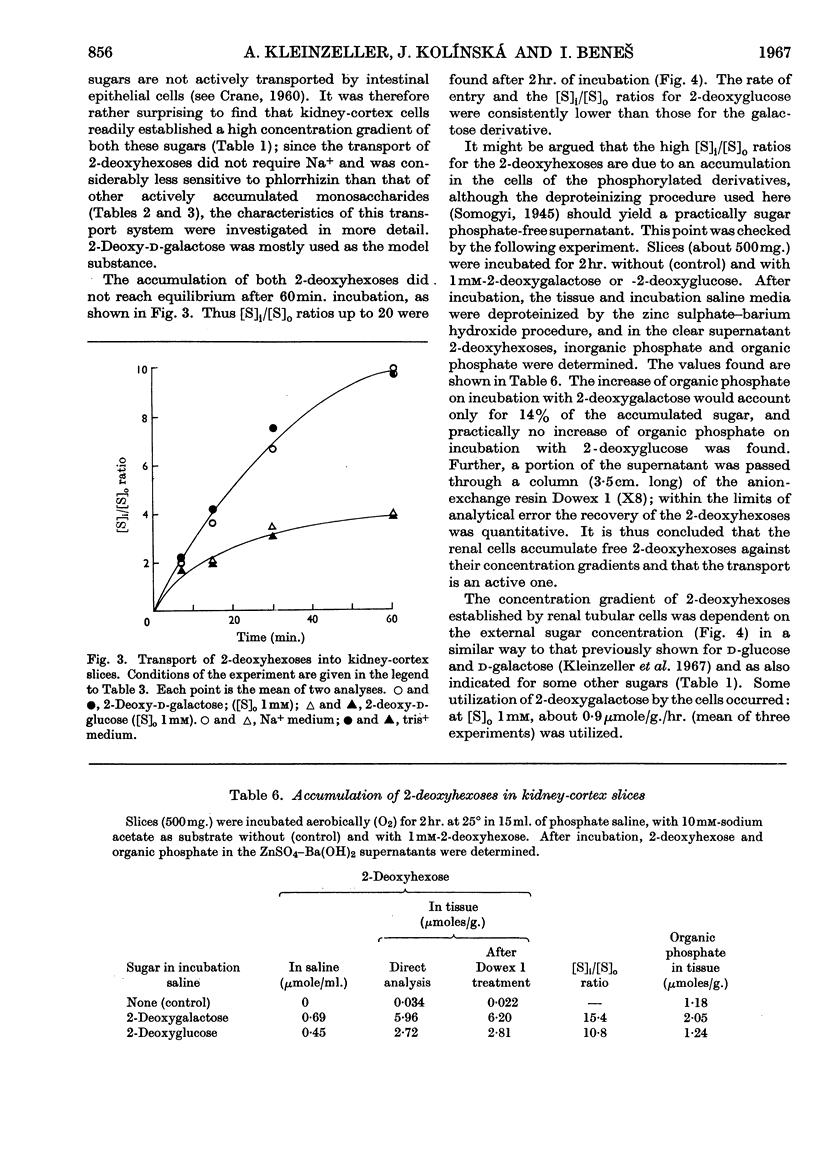
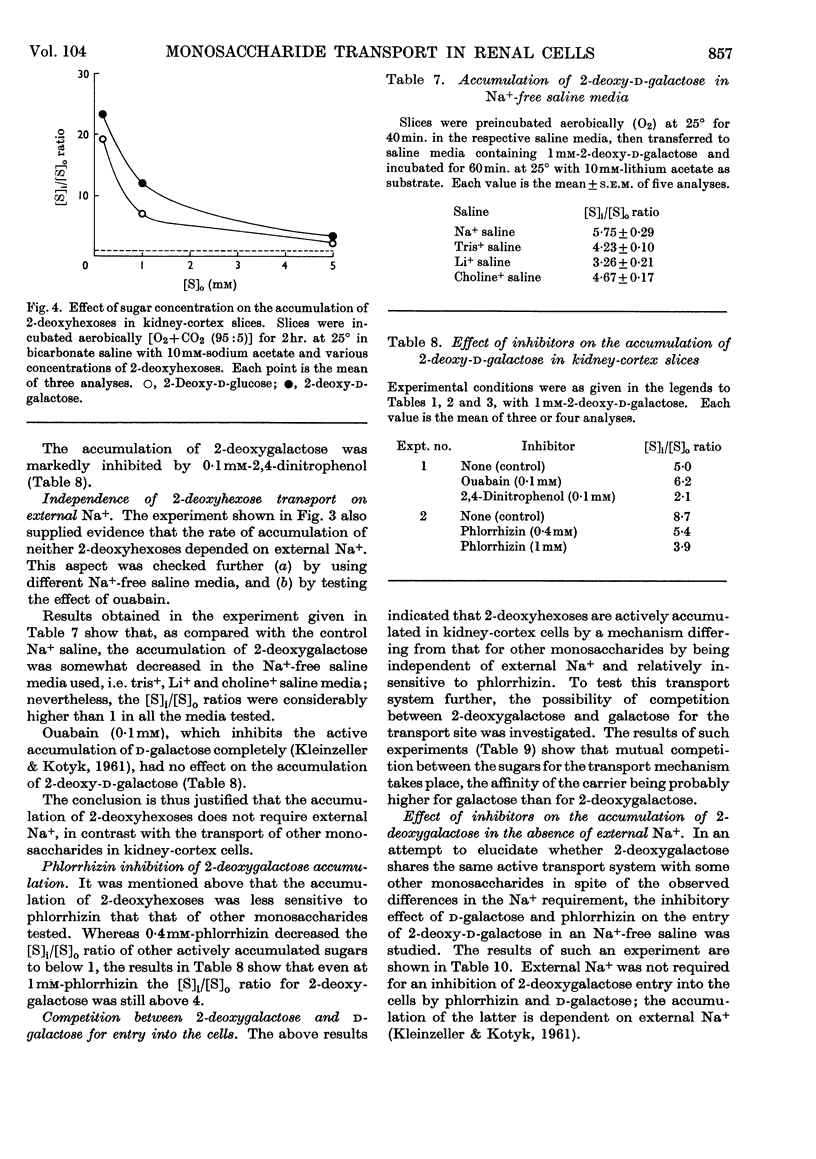
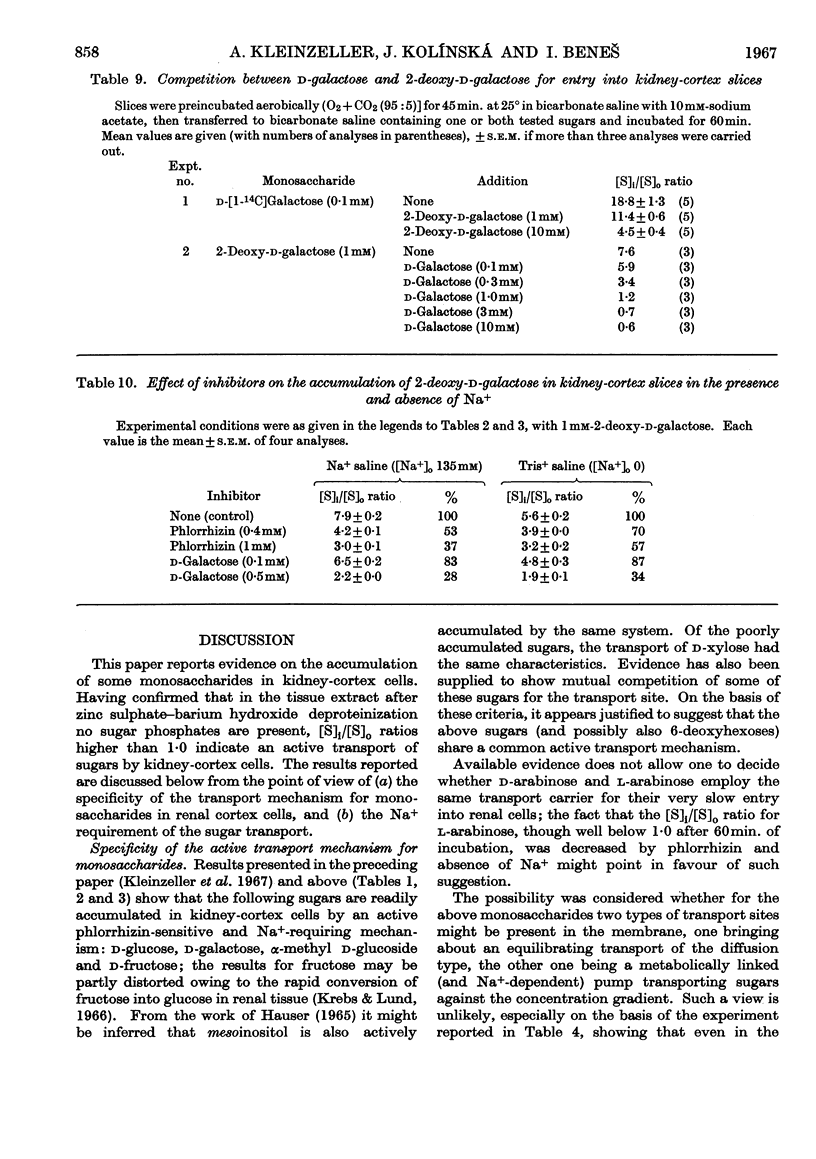
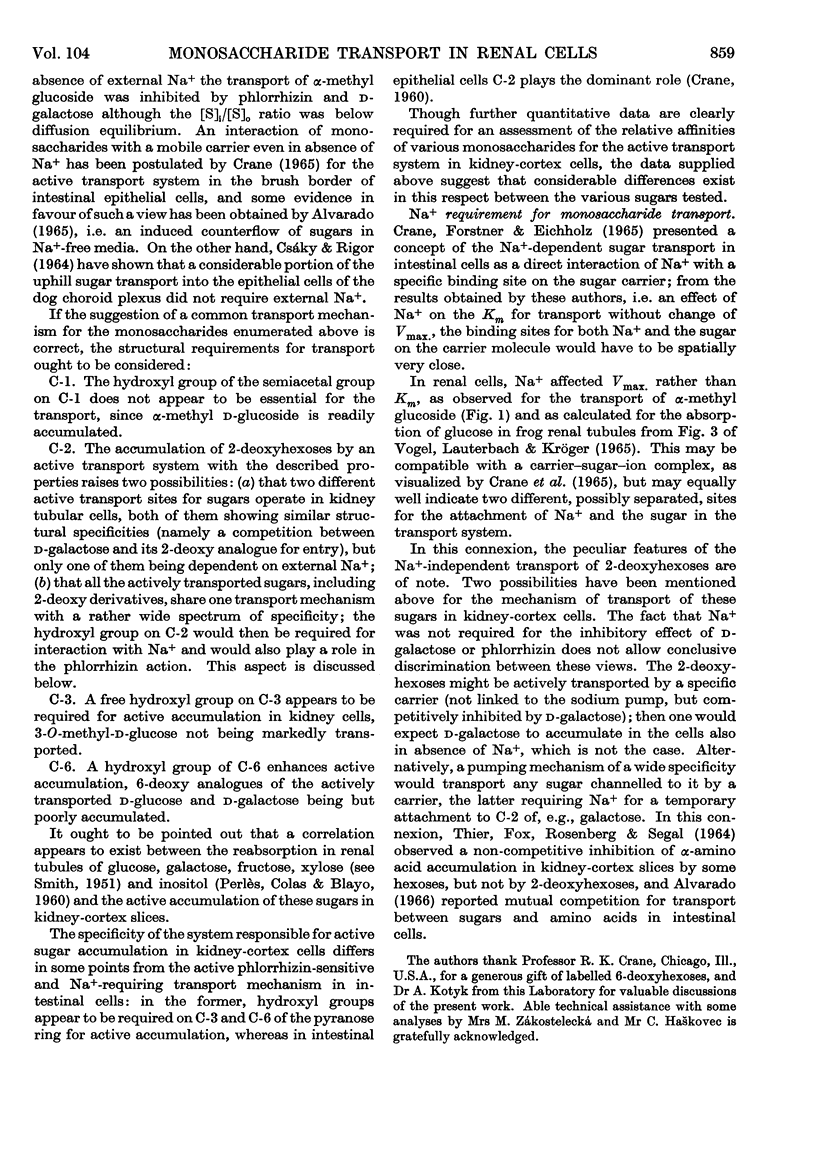
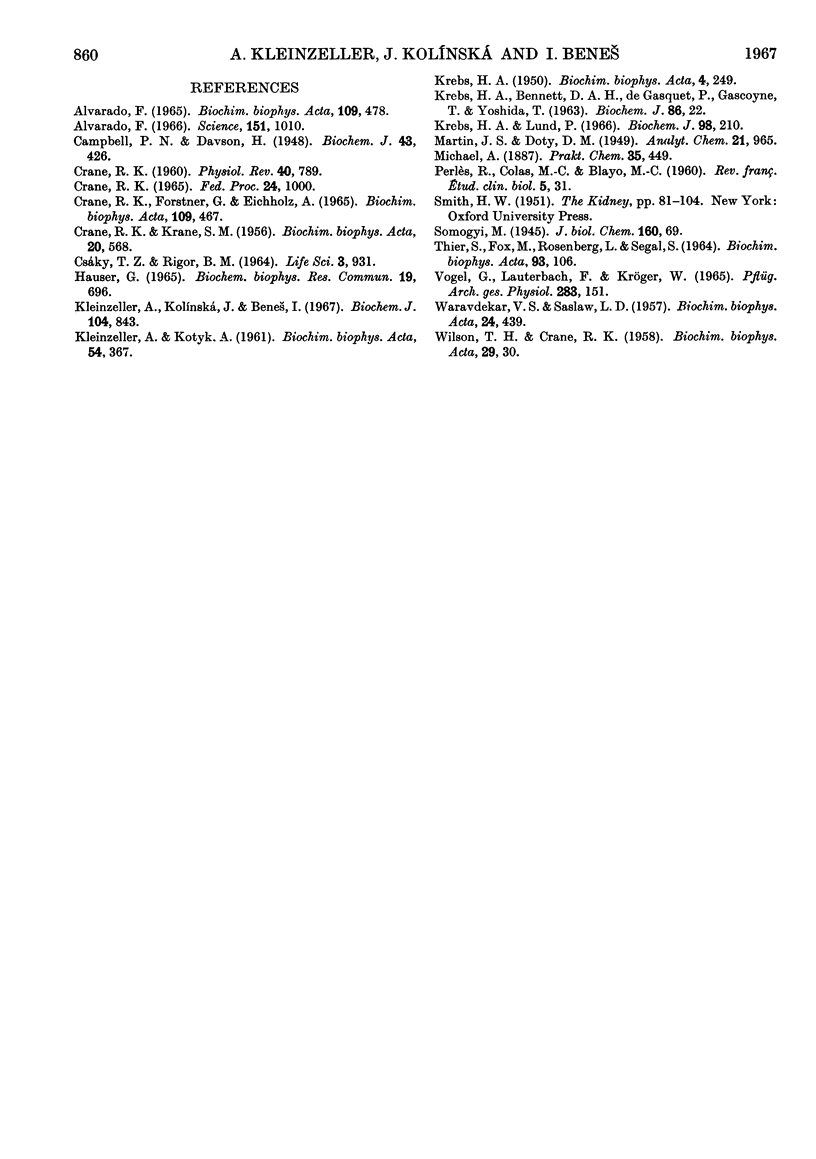
Selected References
These references are in PubMed. This may not be the complete list of references from this article.
- Alvarado F. The relationship between Na+ and the active transport of arbutin in the small intestine. Biochim Biophys Acta. 1965 Nov 29;109(2):478–494. doi: 10.1016/0926-6585(65)90173-1. [DOI] [PubMed] [Google Scholar]
- Alvarado F. Transport of sugars and amino acids in the intestine: evidence for a common carrier. Science. 1966 Feb 25;151(3713):1010–1013. doi: 10.1126/science.151.3713.1010. [DOI] [PubMed] [Google Scholar]
- CRANE R. K. Intestinal absorption of sugars. Physiol Rev. 1960 Oct;40:789–825. doi: 10.1152/physrev.1960.40.4.789. [DOI] [PubMed] [Google Scholar]
- CRANE R. K., KRANE S. M. On the mechanism of the intestinal absorption of sugars. Biochim Biophys Acta. 1956 Jun;20(3):568–569. doi: 10.1016/0006-3002(56)90361-4. [DOI] [PubMed] [Google Scholar]
- CSAKY T. Z., RIGOR B. M., Sr A CONCENTRATIVE MECHANISM FOR SUGARS IN THE CHOROID PLEXUS. Life Sci. 1964 Sep;3:931–936. doi: 10.1016/0024-3205(64)90101-8. [DOI] [PubMed] [Google Scholar]
- Campbell P. N., Davson H. Absorption of 3-methylglucose from the small intestine of the rat and the cat. Biochem J. 1948;43(3):426–429. doi: 10.1042/bj0430426. [DOI] [PMC free article] [PubMed] [Google Scholar]
- Crane R. K., Forstner G., Eichholz A. Studies on the mechanism of the intestinal absorption of sugars. X. An effect of Na+ concentration on the apparent Michaelis constants for intestinal sugar transport, in vitro. Biochim Biophys Acta. 1965 Nov 29;109(2):467–477. doi: 10.1016/0926-6585(65)90172-x. [DOI] [PubMed] [Google Scholar]
- Crane R. K. Na+ -dependent transport in the intestine and other animal tissues. Fed Proc. 1965 Sep-Oct;24(5):1000–1006. [PubMed] [Google Scholar]
- KLEINZELLER A., KOTYK A. Cations and transport of galactose in kidney-cortex slices. Biochim Biophys Acta. 1961 Dec 9;54:367–369. doi: 10.1016/0006-3002(61)90383-3. [DOI] [PubMed] [Google Scholar]
- KREBS H. A., BENNETT D. A., DE GASQUET P., GASQUET P., GASCOYNE T., YOSHIDA T. Renal gluconeogenesis. The effect of diet on the gluconeogenic capacity of rat-kidney-cortex slices. Biochem J. 1963 Jan;86:22–27. doi: 10.1042/bj0860022. [DOI] [PMC free article] [PubMed] [Google Scholar]
- KREBS H. A. Body size and tissue respiration. Biochim Biophys Acta. 1950 Jan;4(1-3):249–269. doi: 10.1016/0006-3002(50)90032-1. [DOI] [PubMed] [Google Scholar]
- Kleinzeller A., Kolínská J., Benes I. Transport of glucose and galactose in kidney-cortex cells. Biochem J. 1967 Sep;104(3):843–851. doi: 10.1042/bj1040843. [DOI] [PMC free article] [PubMed] [Google Scholar]
- Krebs H. A., Lund P. Formation of glucose from hexoses, pentoses, polyols and related substances in kidney cortex. Biochem J. 1966 Jan;98(1):210–214. doi: 10.1042/bj0980210. [DOI] [PMC free article] [PubMed] [Google Scholar]
- THIER S., FOX M., ROSENBERG L., SEGAL S. HEXOSE INHIBITION OF AMINO ACID UPTAKE IN THE RAT-KIDNEY-CORTEX SLICE. Biochim Biophys Acta. 1964 Oct 9;93:106–115. doi: 10.1016/0304-4165(64)90265-x. [DOI] [PubMed] [Google Scholar]
- VOGEL G., LAUTERBACH F., KROEGER W. DIE BEDEUTUNG DES NATRIUMS FUER DIE RENALEN TRANSPORTE VON GLUCOSE UND PARA-AMINOHIPPURSAEURE. Pflugers Arch Gesamte Physiol Menschen Tiere. 1965 Mar 18;283:151–159. [PubMed] [Google Scholar]
- WARAVDEKAR V. S., SASLAW L. D. A method of estimation of 2-deoxyribose. Biochim Biophys Acta. 1957 May;24(2):439–439. doi: 10.1016/0006-3002(57)90224-x. [DOI] [PubMed] [Google Scholar]
- WILSON T. H., CRANE R. K. The specificity of sugar transport by hamster intestine. Biochim Biophys Acta. 1958 Jul;29(1):30–32. doi: 10.1016/0006-3002(58)90142-2. [DOI] [PubMed] [Google Scholar]