Abstract
Membrane fluidity was measured in the isolated perfused proximal tubule from rabbit kidney. The apical and basolateral plasma membranes of tubule cells were stained separately with the fluidity-sensitive fluorophore trimethylammonium-diphenyl-hexatriene (TMA-DPH) by luminal or bath perfusion. Fluorescence anisotropy (r) of TMA-DPH was mapped with spatial resolution using an epifluorescence microscope (excitation 380 nm, emission greater than 410 nm) equipped with rotatable polarizers and a quantitative imaging system. To measure r without the confounding effects of fluorophore orientation, images were recorded with emission polarizer parallel and perpendicular to a continuum of orientations of the excitation polarizer. The theoretical basis of this approach was developed and its limitations were evaluated by mathematical modeling. The tubule inner surface (brush border) was brightly stained when the lumen was perfused with 1 microM TMA-DPH for 5 min; apical membrane r was 0.281 +/- 0.006 (23 degrees C). Staining of the tubule basolateral membrane by addition of TMA-DPH to the bath gave a significantly lower r of 0.242 +/- 0.010 (P less than 0.005); there was no staining of the brush border membrane. To interpret anisotropy images quantitatively, effects of tubule geometry, TMA-DPH lifetime, fluorescence anisotropy decay, and objective-depolarization were evaluated. Steady-state and time-resolved r and lifetimes in the intact tubule, measured by a nanosecond pulsed microscopy method, were compared with results in isolated apical and basolateral membrane vesicles from rabbit proximal tubule measured by cuvette fluorometry; r was 0.281 (apical membrane) and 0.276 (basolateral membrane) (23 degrees C). These results establish a methodology to quantitate membrane fluidity in the intact proximal tubule, and demonstrate a significantly higher fluidity in the basolateral membrane than in the apical membrane.
Full text
PDF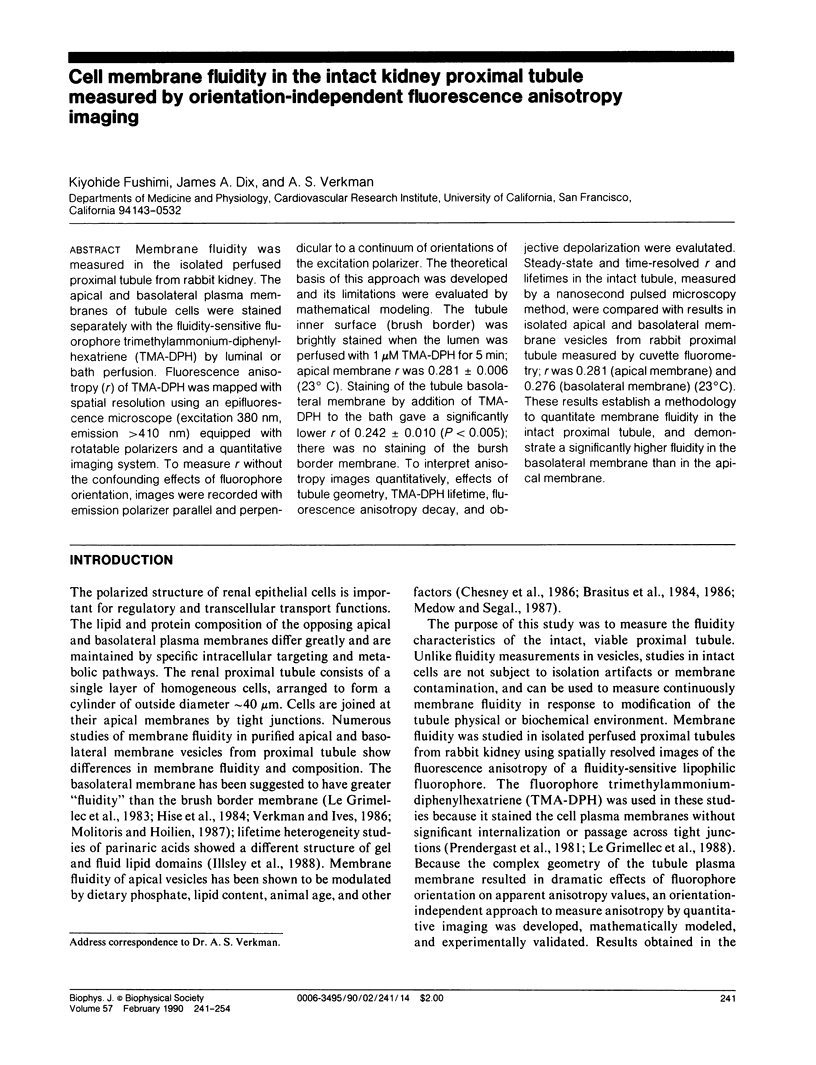
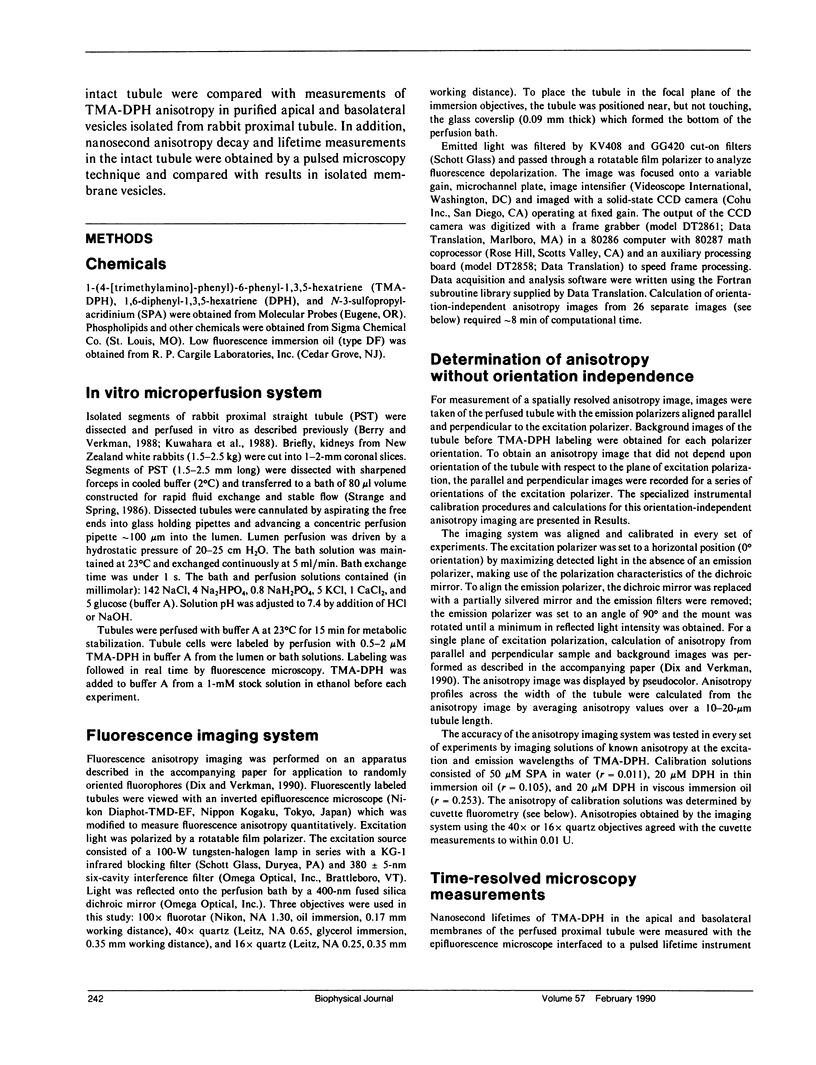
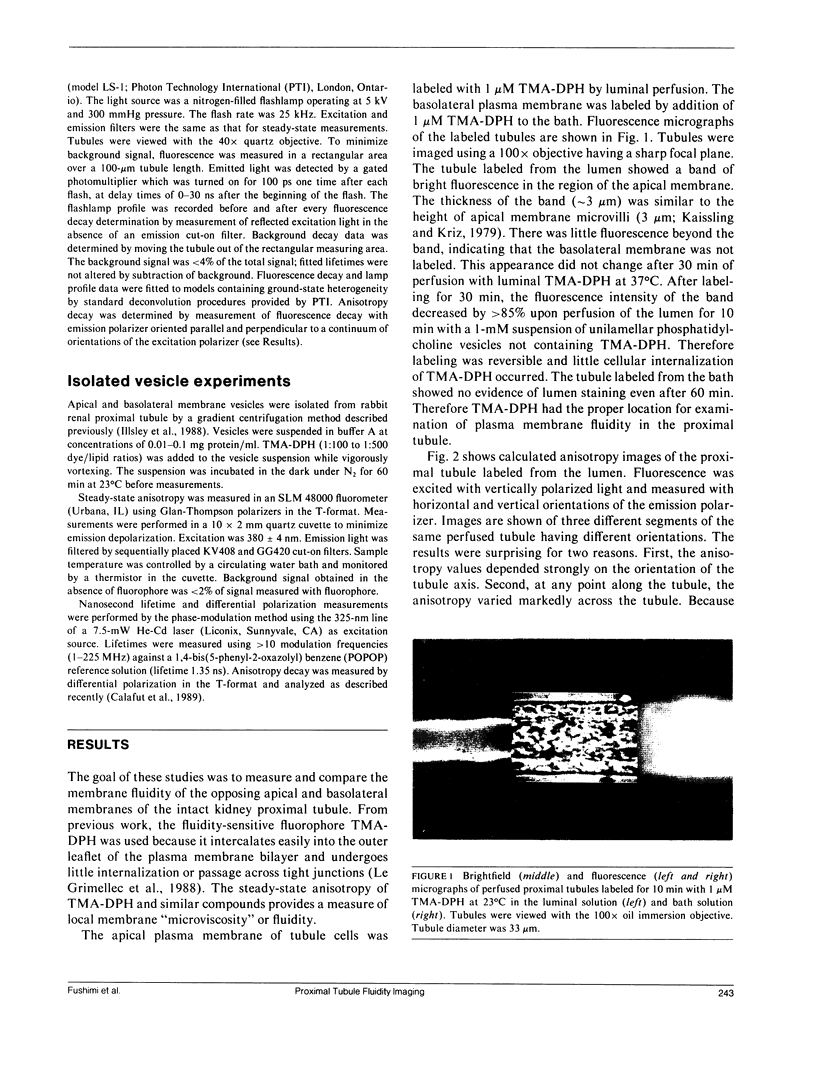
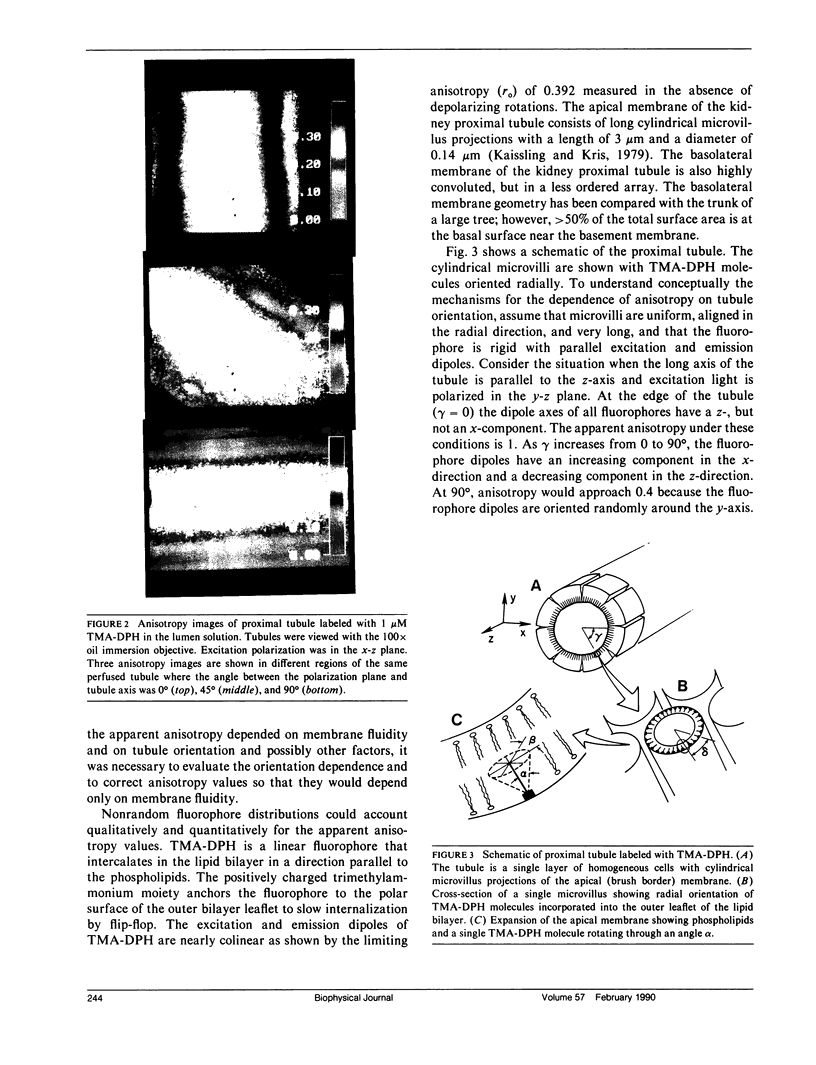
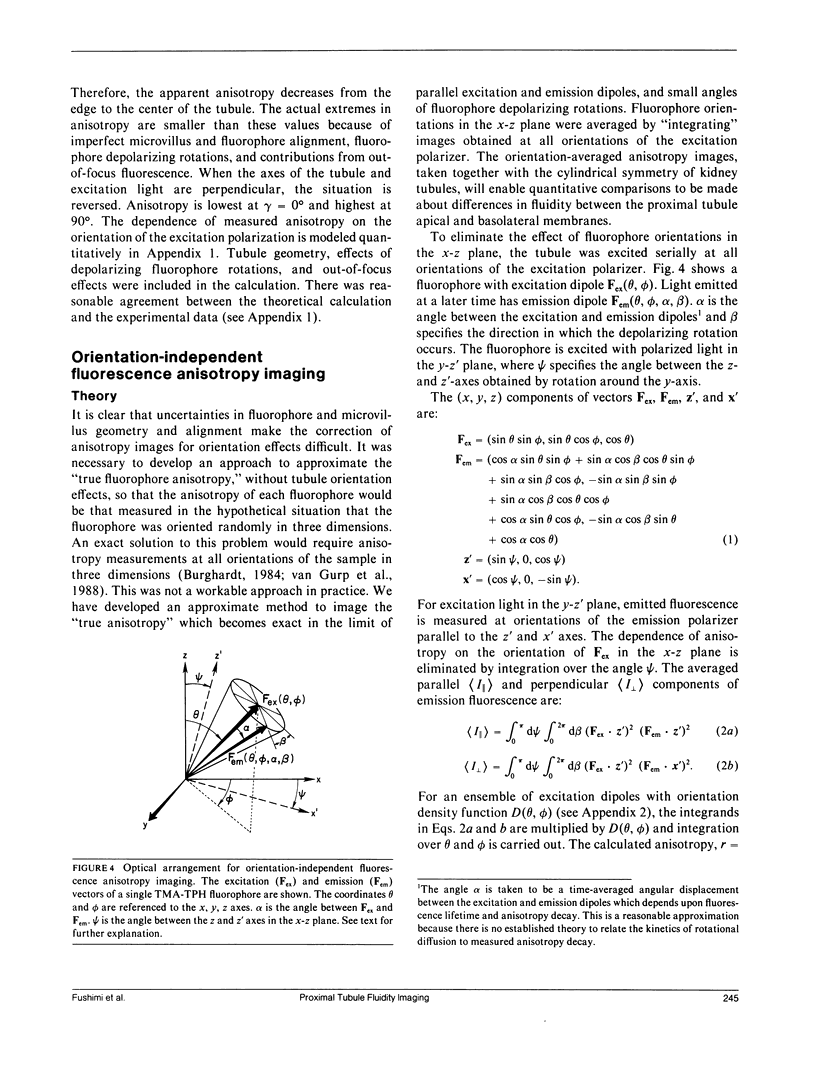
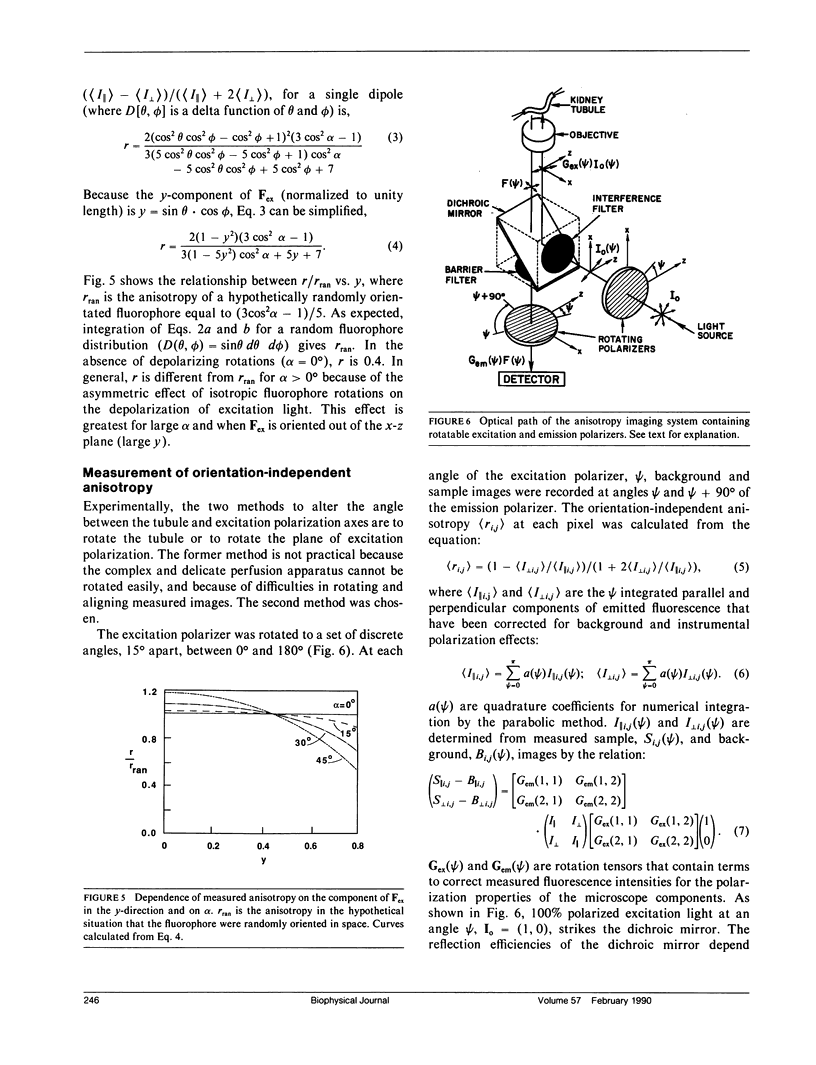
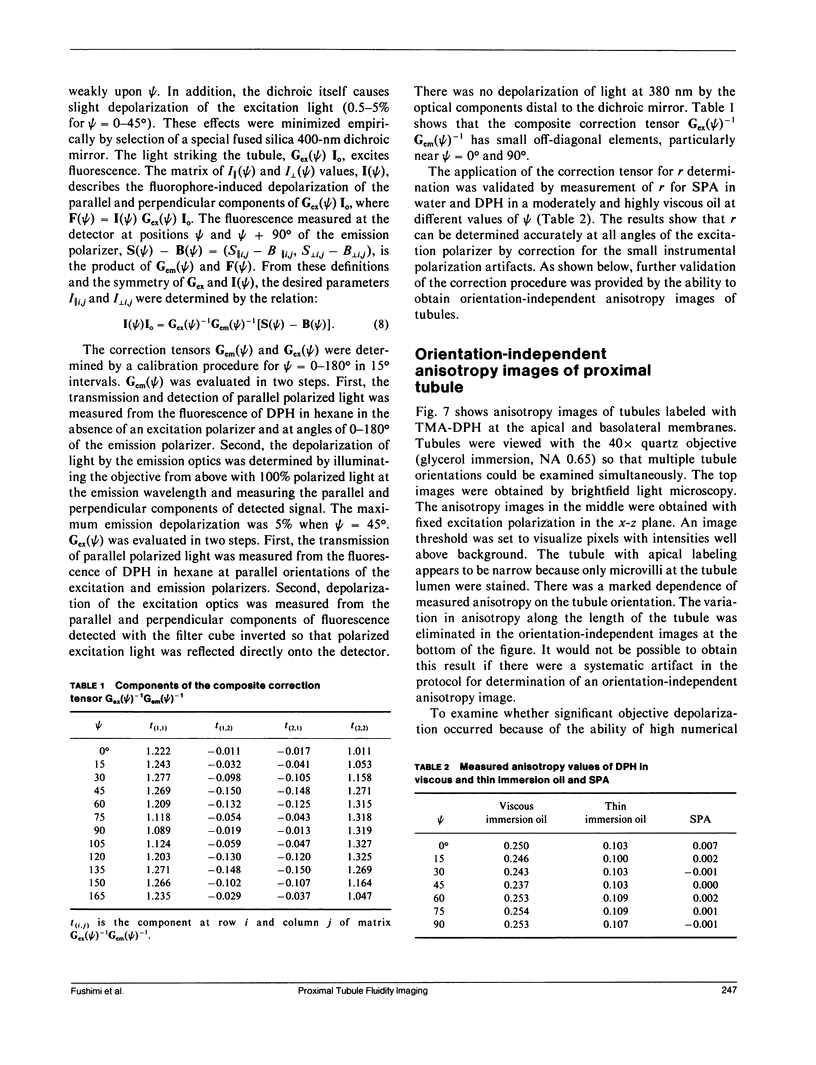
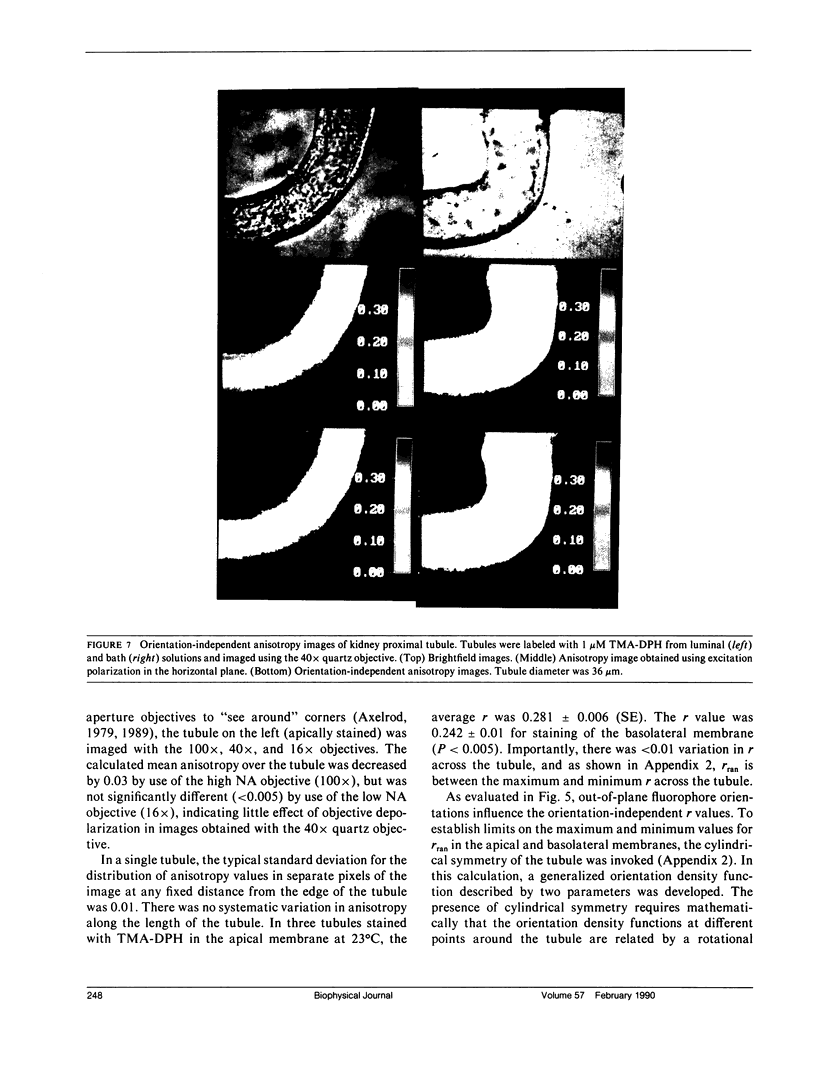
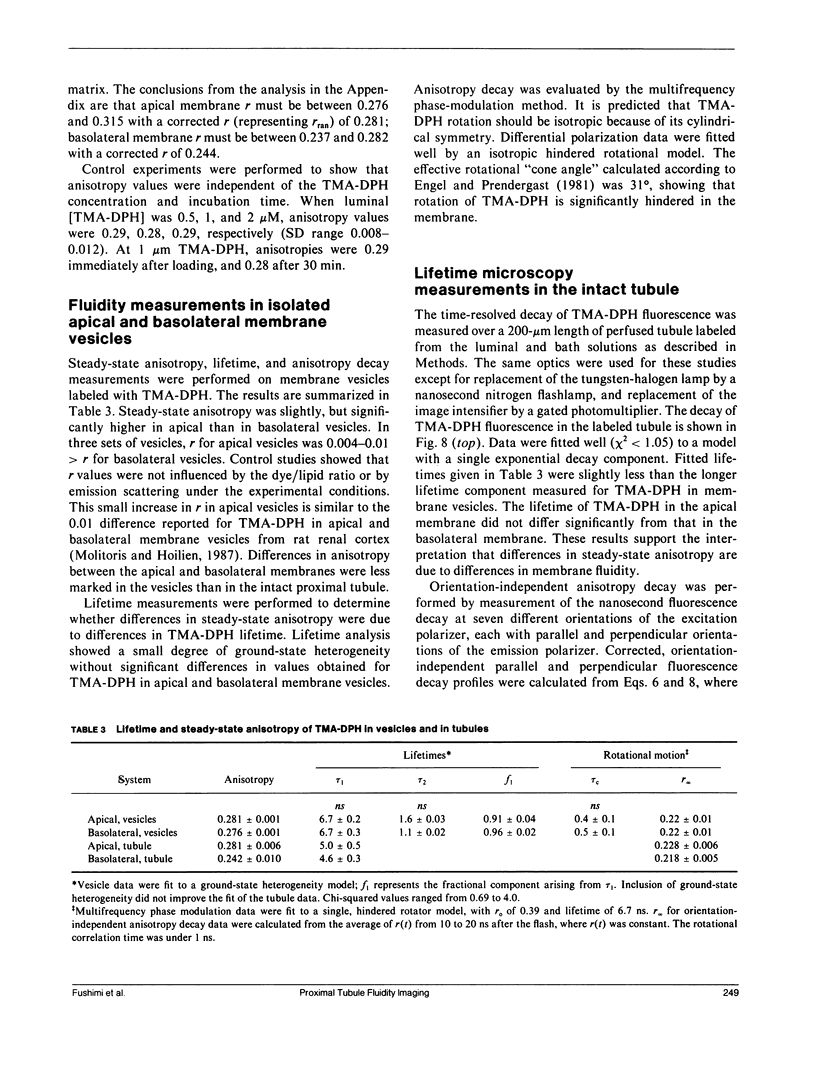
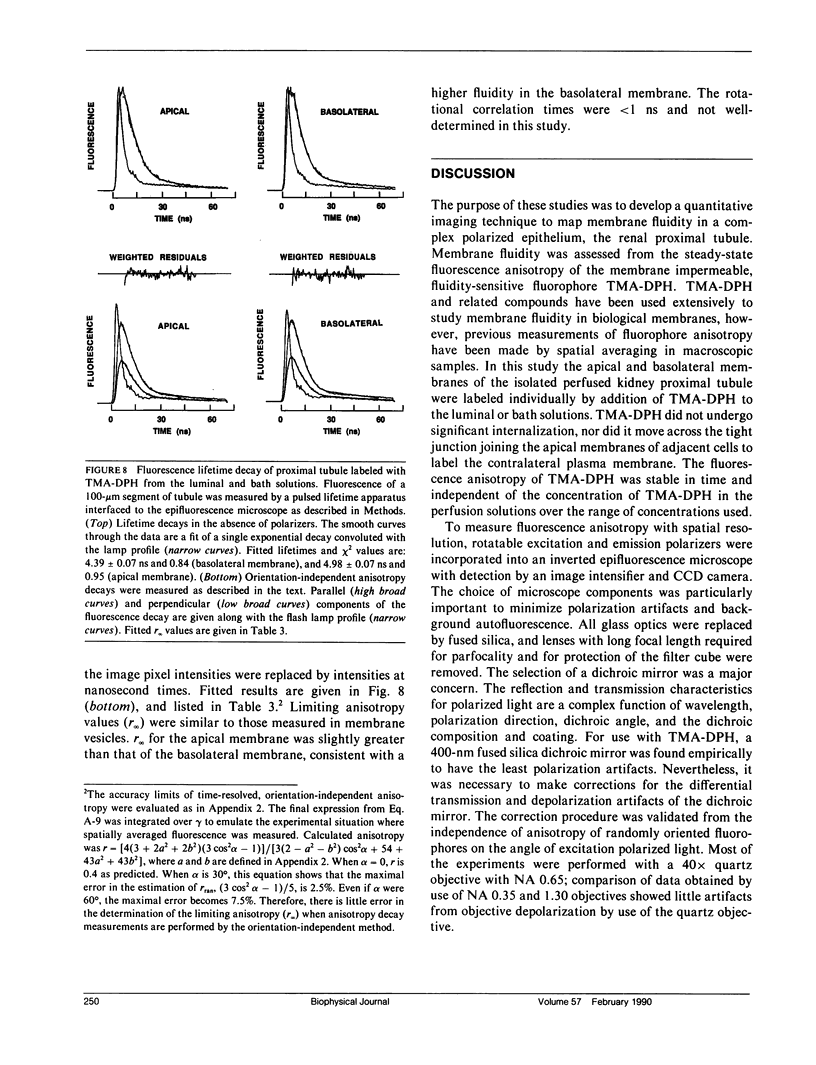
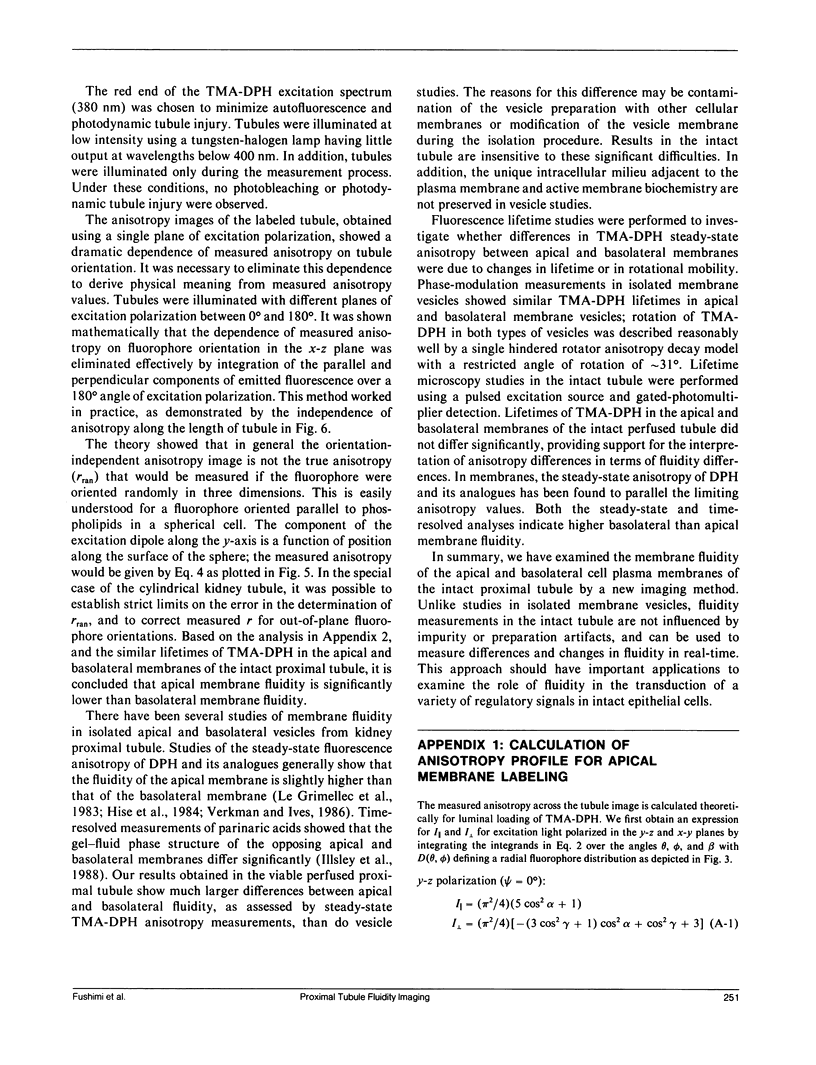
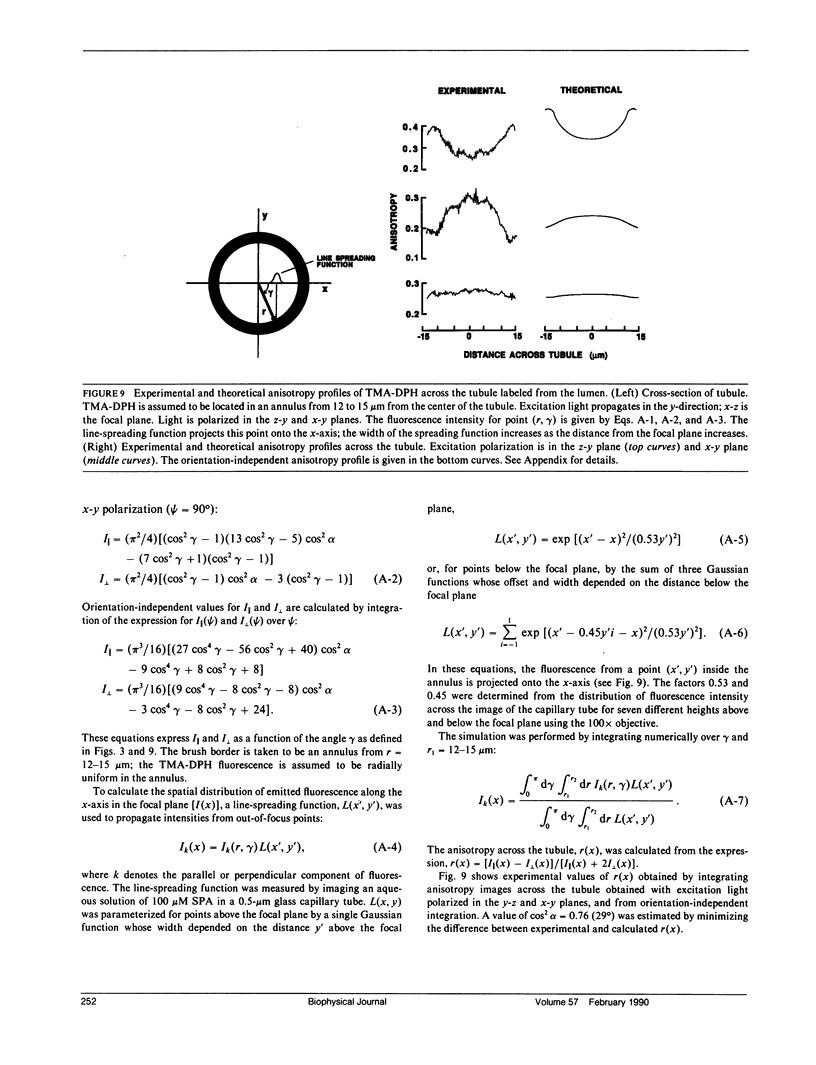
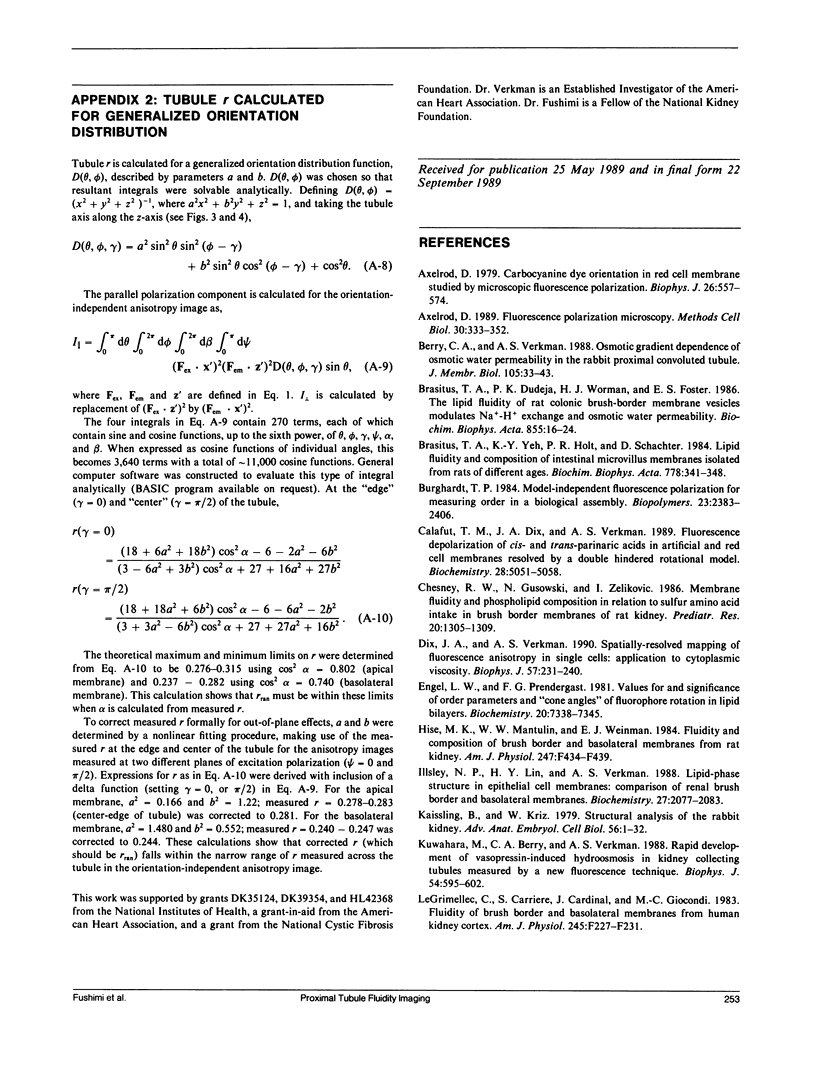
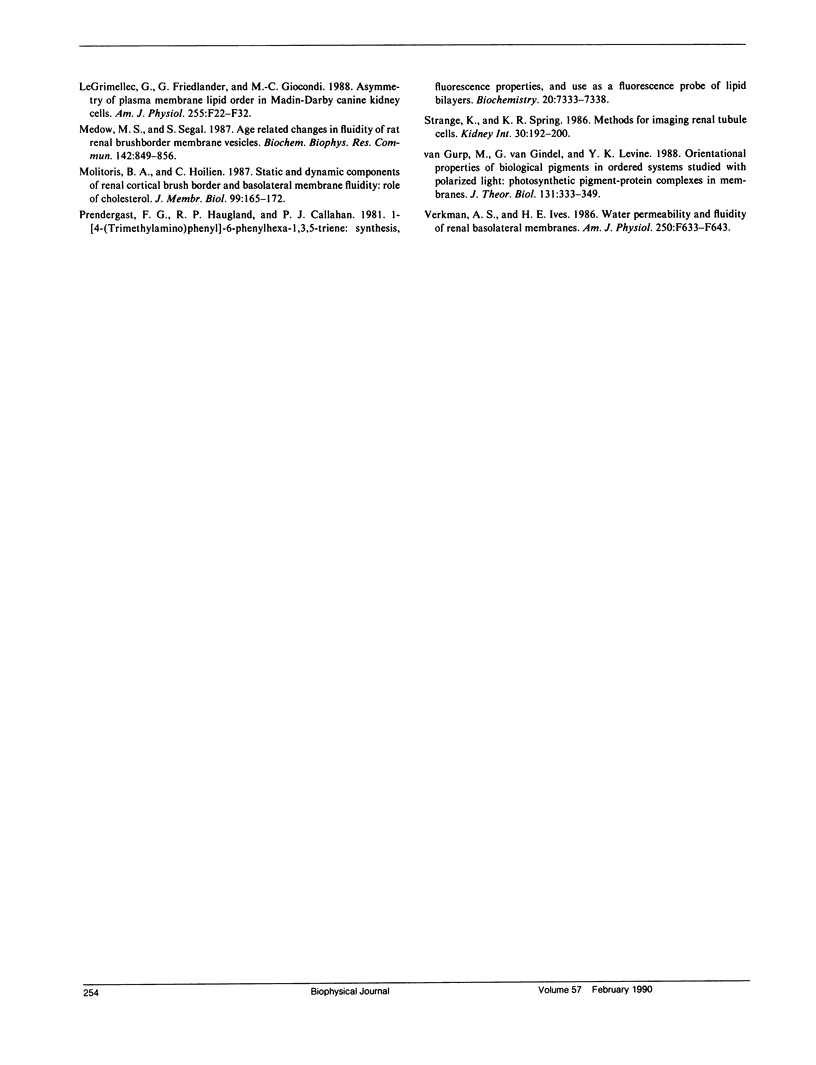
Images in this article
Selected References
These references are in PubMed. This may not be the complete list of references from this article.
- Axelrod D. Carbocyanine dye orientation in red cell membrane studied by microscopic fluorescence polarization. Biophys J. 1979 Jun;26(3):557–573. doi: 10.1016/S0006-3495(79)85271-6. [DOI] [PMC free article] [PubMed] [Google Scholar]
- Axelrod D. Fluorescence polarization microscopy. Methods Cell Biol. 1989;30:333–352. [PubMed] [Google Scholar]
- Berry C. A., Verkman A. S. Osmotic gradient dependence of osmotic water permeability in rabbit proximal convoluted tubule. J Membr Biol. 1988 Oct;105(1):33–43. doi: 10.1007/BF01871104. [DOI] [PubMed] [Google Scholar]
- Brasitus T. A., Dudeja P. K., Worman H. J., Foster E. S. The lipid fluidity of rat colonic brush-border membrane vesicles modulates Na+-H+ exchange and osmotic water permeability. Biochim Biophys Acta. 1986 Feb 13;855(1):16–24. doi: 10.1016/0005-2736(86)90183-5. [DOI] [PubMed] [Google Scholar]
- Brasitus T. A., Yeh K. Y., Holt P. R., Schachter D. Lipid fluidity and composition of intestinal microvillus membranes isolated from rats of different ages. Biochim Biophys Acta. 1984 Dec 5;778(2):341–348. doi: 10.1016/0005-2736(84)90378-x. [DOI] [PubMed] [Google Scholar]
- Burghardt T. P. Model-independent fluorescence polarization for measuring order in a biological assembly. Biopolymers. 1984 Nov;23(11 Pt 2):2383–2406. doi: 10.1002/bip.360231118. [DOI] [PubMed] [Google Scholar]
- Calafut T. M., Dix J. A., Verkman A. S. Fluorescence depolarization of cis- and trans-parinaric acids in artificial and red cell membranes resolved by a double hindered rotational model. Biochemistry. 1989 Jun 13;28(12):5051–5058. doi: 10.1021/bi00438a023. [DOI] [PubMed] [Google Scholar]
- Chesney R. W., Gusowski N., Zelikovic I. Membrane fluidity and phospholipid composition in relation to sulfur amino acid intake in brush border membranes of rat kidney. Pediatr Res. 1986 Dec;20(12):1305–1309. doi: 10.1203/00006450-198612000-00024. [DOI] [PubMed] [Google Scholar]
- Dix J. A., Verkman A. S. Mapping of fluorescence anisotropy in living cells by ratio imaging. Application to cytoplasmic viscosity. Biophys J. 1990 Feb;57(2):231–240. doi: 10.1016/S0006-3495(90)82526-1. [DOI] [PMC free article] [PubMed] [Google Scholar]
- Engel L. W., Prendergast F. G. Values for and significance of order parameters and "cone angles" of fluorophore rotation in lipid bilayers. Biochemistry. 1981 Dec 22;20(26):7338–7345. doi: 10.1021/bi00529a003. [DOI] [PubMed] [Google Scholar]
- Hise M. K., Mantulin W. W., Weinman E. J. Fluidity and composition of brush border and basolateral membranes from rat kidney. Am J Physiol. 1984 Sep;247(3 Pt 2):F434–F439. doi: 10.1152/ajprenal.1984.247.3.F434. [DOI] [PubMed] [Google Scholar]
- Illsley N. P., Lin H. Y., Verkman A. S. Lipid-phase structure in epithelial cell membranes: comparison of renal brush border and basolateral membranes. Biochemistry. 1988 Mar 22;27(6):2077–2083. doi: 10.1021/bi00406a039. [DOI] [PubMed] [Google Scholar]
- Kaissling B., Kriz W. Structural analysis of the rabbit kidney. Adv Anat Embryol Cell Biol. 1979;56:1–123. doi: 10.1007/978-3-642-67147-0. [DOI] [PubMed] [Google Scholar]
- Kuwahara M., Berry C. A., Verkman A. S. Rapid development of vasopressin-induced hydroosmosis in kidney collecting tubules measured by a new fluorescence technique. Biophys J. 1988 Oct;54(4):595–602. doi: 10.1016/S0006-3495(88)82994-1. [DOI] [PMC free article] [PubMed] [Google Scholar]
- Le Grimellec C., Carrière S., Cardinal J., Giocondi M. C. Fluidity of brush border and basolateral membranes from human kidney cortex. Am J Physiol. 1983 Aug;245(2):F227–F231. doi: 10.1152/ajprenal.1983.245.2.F227. [DOI] [PubMed] [Google Scholar]
- Le Grimellec C., Friedlander G., Giocondi M. C. Asymmetry of plasma membrane lipid order in Madin-Darby Canine Kidney cells. Am J Physiol. 1988 Jul;255(1 Pt 2):F22–F32. doi: 10.1152/ajprenal.1988.255.1.F22. [DOI] [PubMed] [Google Scholar]
- Medow M. S., Segal S. Age related changes in fluidity of rat renal brushborder membrane vesicles. Biochem Biophys Res Commun. 1987 Feb 13;142(3):849–856. doi: 10.1016/0006-291x(87)91491-4. [DOI] [PubMed] [Google Scholar]
- Molitoris B. A., Hoilien C. Static and dynamic components of renal cortical brush border and basolateral membrane fluidity: role of cholesterol. J Membr Biol. 1987;99(3):165–172. doi: 10.1007/BF01995697. [DOI] [PubMed] [Google Scholar]
- Prendergast F. G., Haugland R. P., Callahan P. J. 1-[4-(Trimethylamino)phenyl]-6-phenylhexa-1,3,5-triene: synthesis, fluorescence properties, and use as a fluorescence probe of lipid bilayers. Biochemistry. 1981 Dec 22;20(26):7333–7338. doi: 10.1021/bi00529a002. [DOI] [PubMed] [Google Scholar]
- Strange K., Spring K. R. Methods for imaging renal tubule cells. Kidney Int. 1986 Aug;30(2):192–200. doi: 10.1038/ki.1986.171. [DOI] [PubMed] [Google Scholar]
- Van Gurp M., Van Ginkel G., Levine Y. K. Orientational properties of biological pigments in ordered systems studied with polarized light: photosynthetic pigment-protein complexes in membranes. J Theor Biol. 1988 Apr 7;131(3):333–349. doi: 10.1016/s0022-5193(88)80229-7. [DOI] [PubMed] [Google Scholar]
- Verkman A. S., Ives H. E. Water permeability and fluidity of renal basolateral membranes. Am J Physiol. 1986 Apr;250(4 Pt 2):F633–F643. doi: 10.1152/ajprenal.1986.250.4.F633. [DOI] [PubMed] [Google Scholar]