Abstract
We measured the dependence of electrophoretic mobility of dipalmitoylphosphatidylcholine (DPPC) vesicles on the aqueous concentration of negatively charged ions of pentachlorophenol (PCP), dipicrylamine (DPA), and tetraphenylborate (TPhB). The objective was to determine how the physical state of hydrocarbon chains of lipids affects adsorption of lipophilic ions. The studies were done at 25 and 42 degrees C to determine adsorption properties of DPPC membrane in the gel and fluid state, respectively. From the analysis of zeta-potential isotherms in terms of Langmuir-Stern-Grahame model we obtained the association constant, K, the area of the adsorption site, Ps, and the linear partition coefficient, beta. Results: K, (x 10(4)M-1): K(gel): PCP (0.49 +/- 0.28), DPA (25 +/- 10), TPhB (31 +/- 10); K(fluid): PCP (4.5 +/- 0.9), DPA (74 +/- 21), TPhB (59 +/- 14); Ps, (nm2): Ps(gel): PCP (5.4 +/- 2.3), DPA (5.9 +/- 2), TPhB (5.0 +/- 1.7); Ps(fluid): PCP (4.5 +/- 0.4), DPA (5.2 +/- 0.4), TPhB (4.1 +/- 0.2); beta, (x 10(-5) m): beta(gel): PCP (0.15 +/- 0.09), DPA (7.1 +/- 0.3), TPhB (10 +/- 7); beta(fluid): PCP (1.7 +/- 0.3), DPA (24 +/- 7), TPhB (24 +/- 6). It was interesting to find that the adsorption site area for PCP, DPA, and TPhB were very similar for both the gel and fluid membranes; also, the areas were independent of the size and molecular structure of the adsorbing species. Using a simple discrete charge model the adsorption site areas for all species were consistent with a dielectric constant of 8-10 and with an ion adsorption depth of 0.4-0.6 nm below the water/dielectric interface. The delta delta G0 = delta G0(gel) - delta G0(fluid) was found to be about twice as large for PCP than for DPA and TPhB. This indicates that PCP will be significantly more adsorbed in the fluid and disordered regions of biomembranes, whereas the distribution of DPA and TPhB is expected to be relatively more even.
Full text
PDF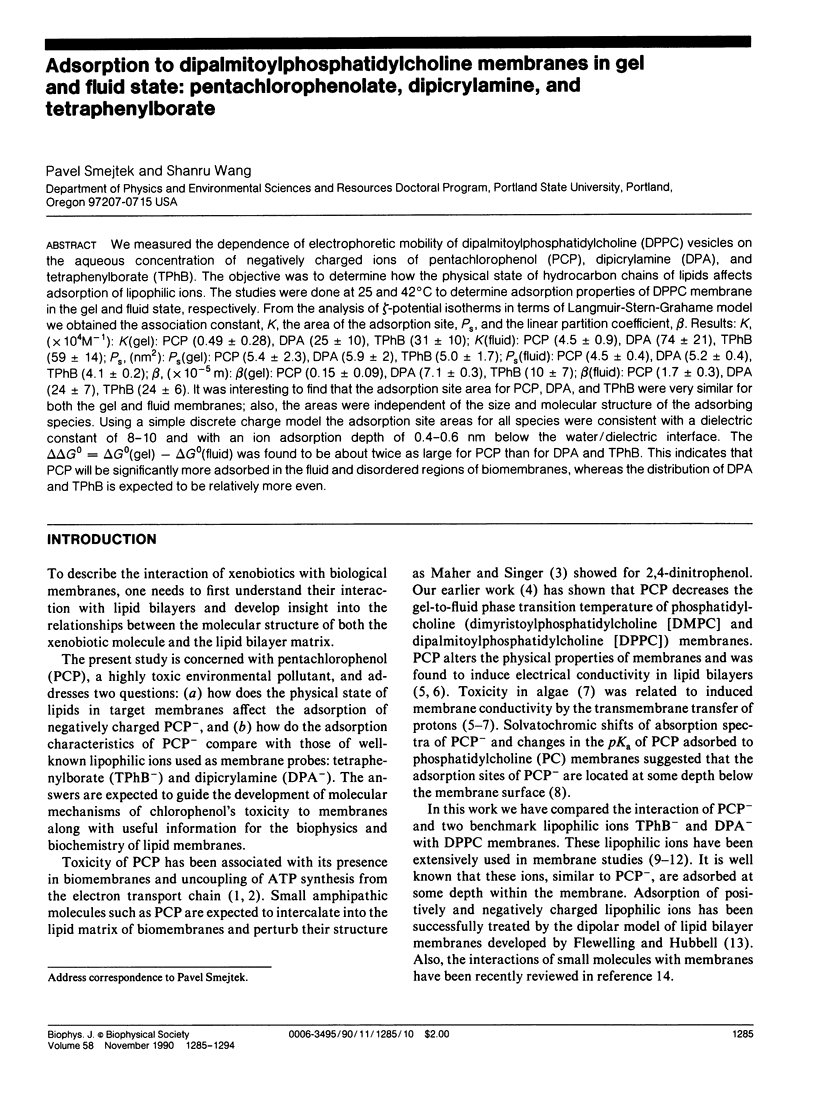
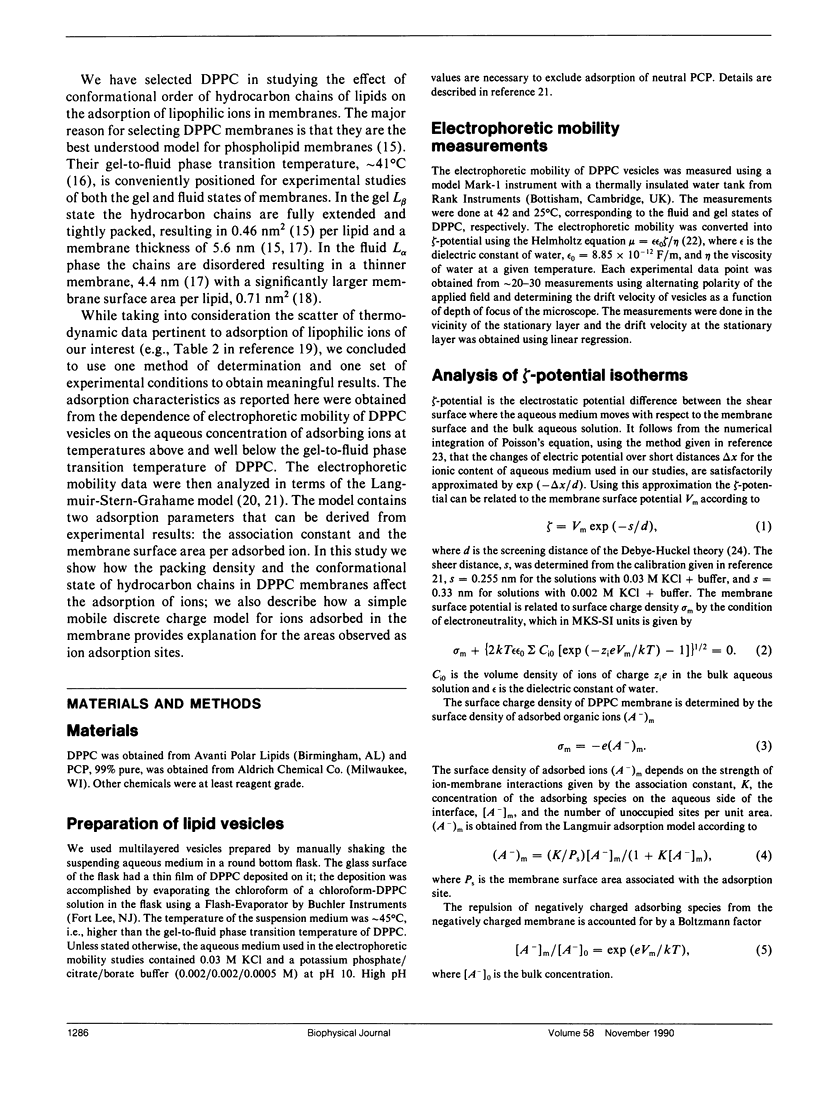
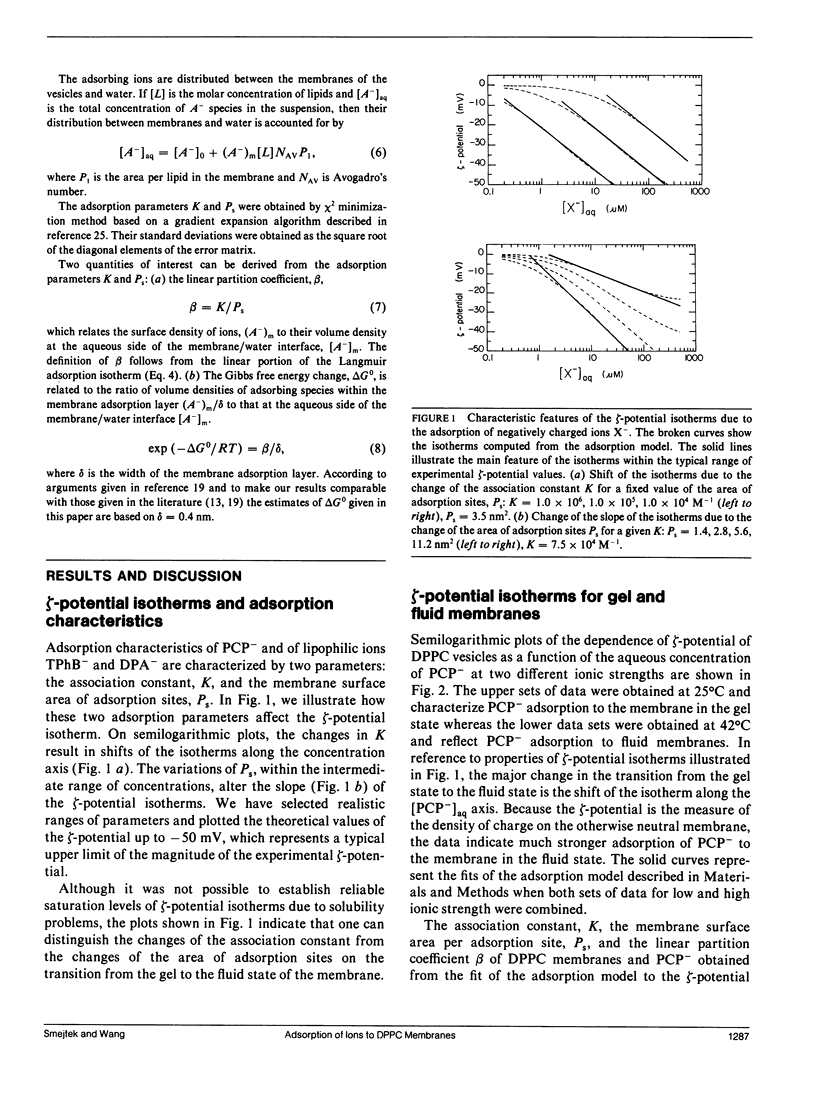
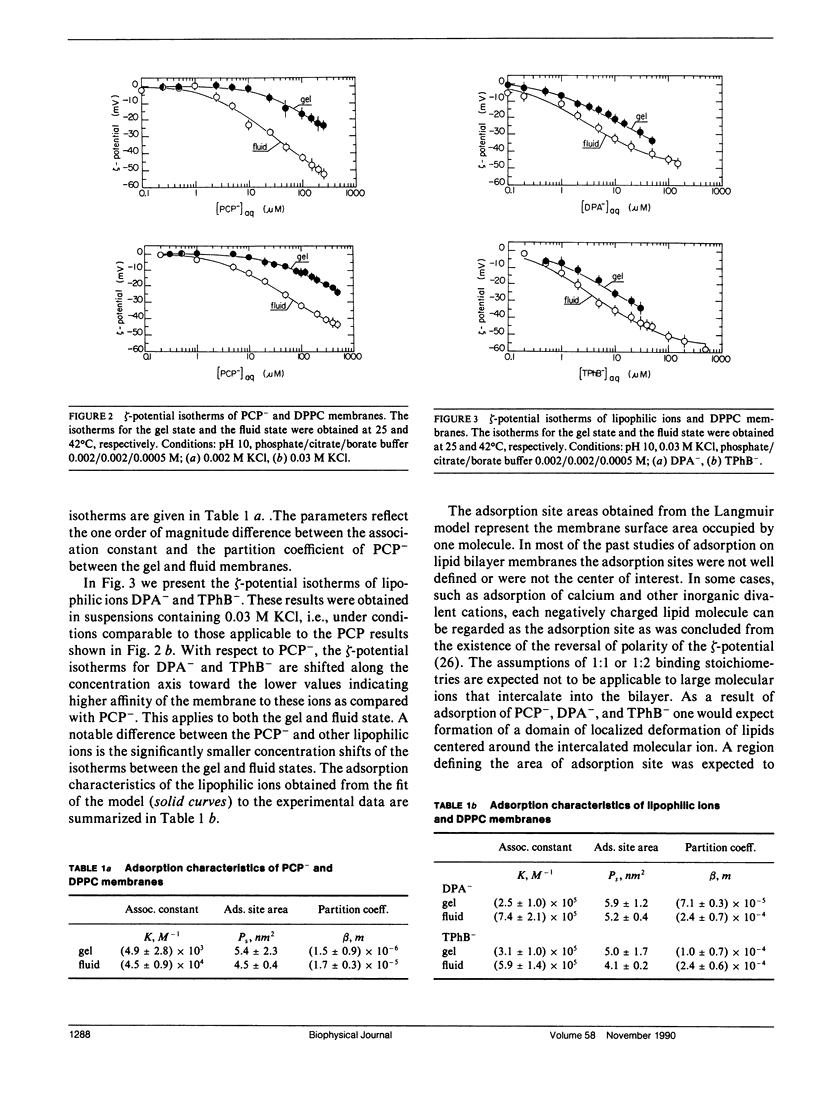
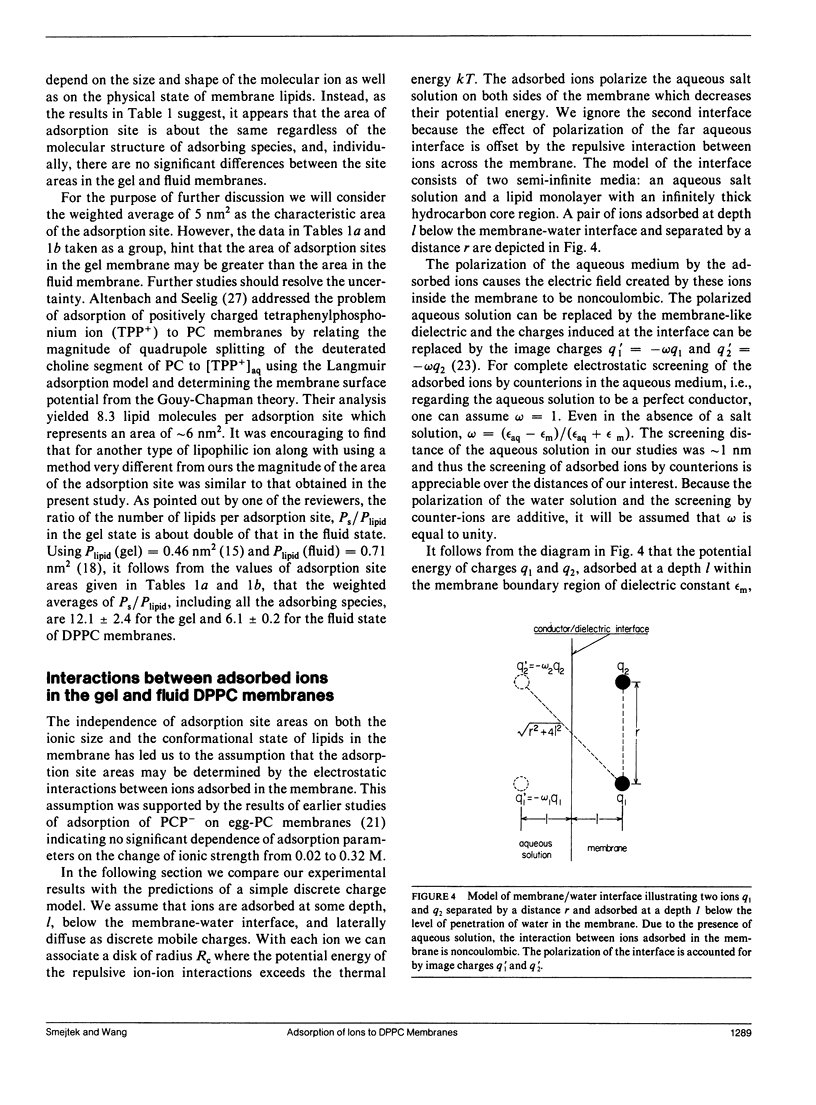
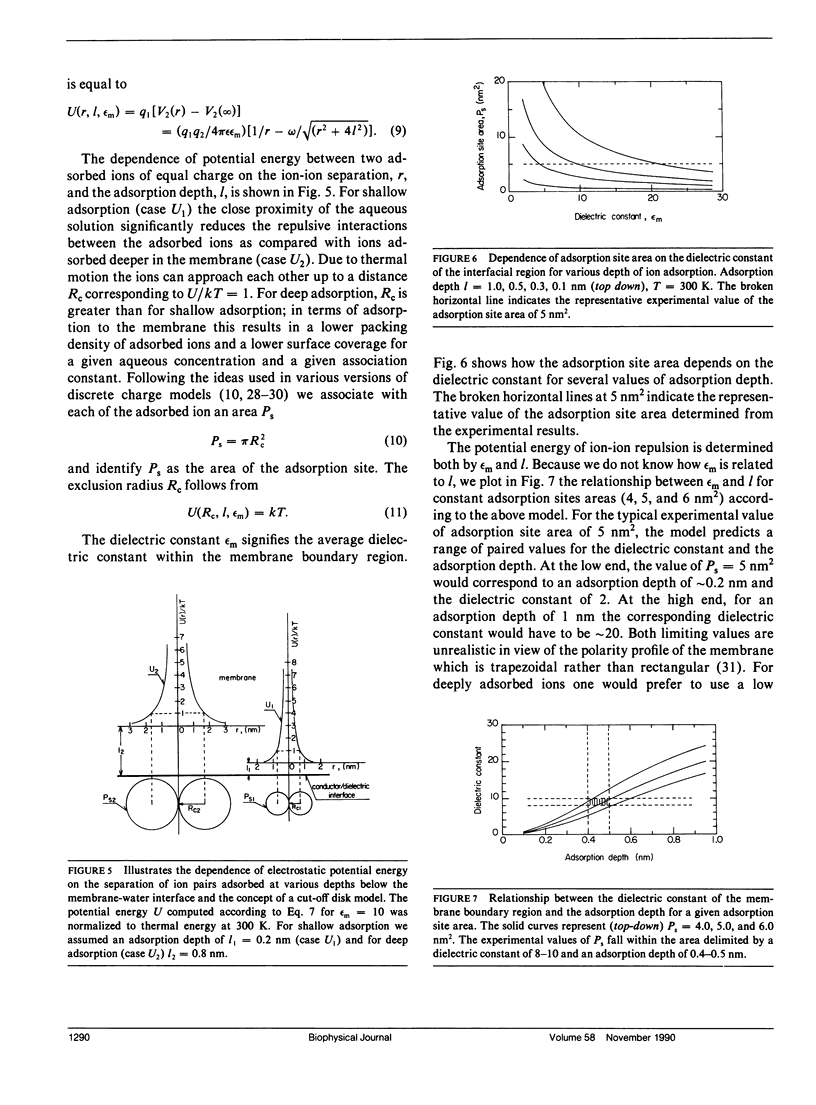
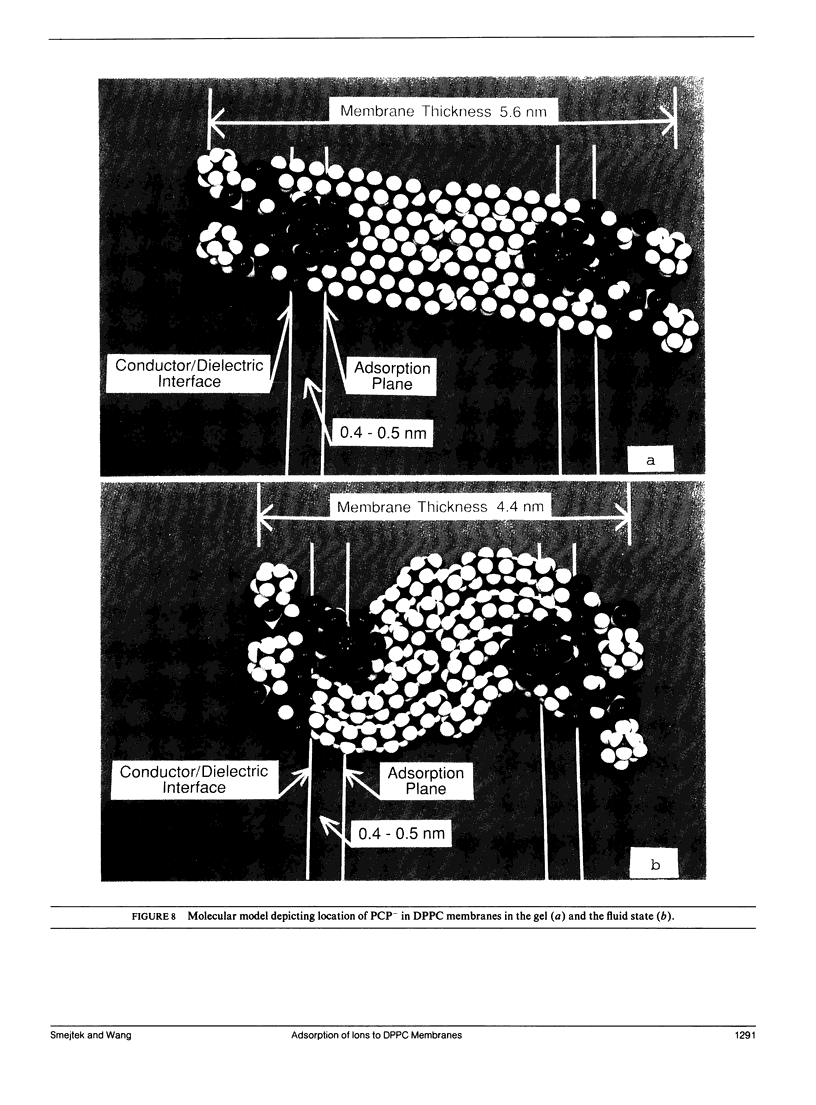
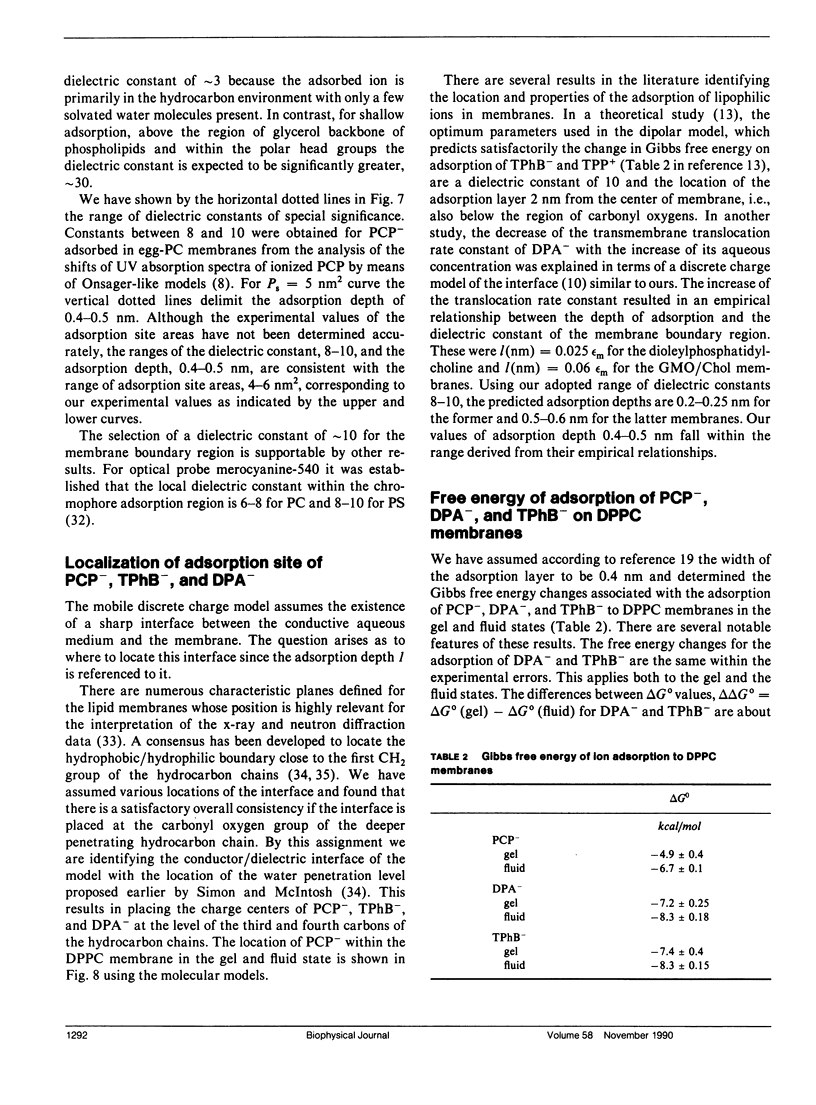
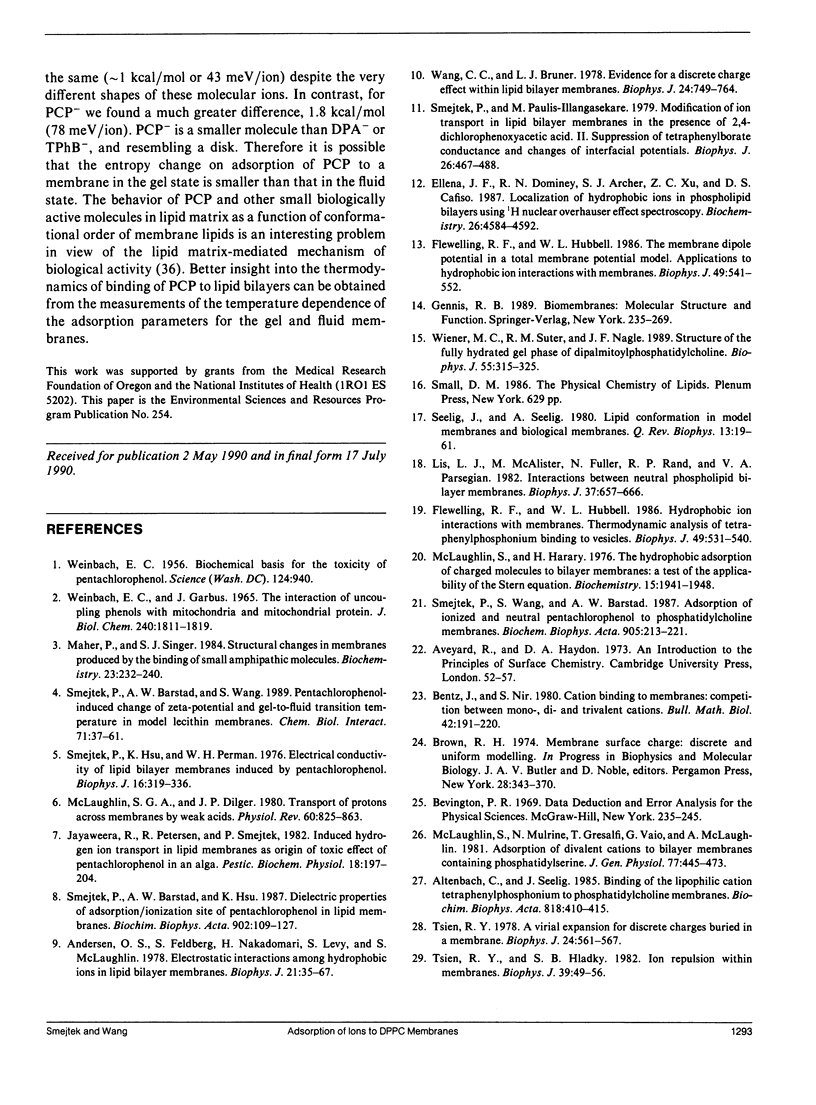
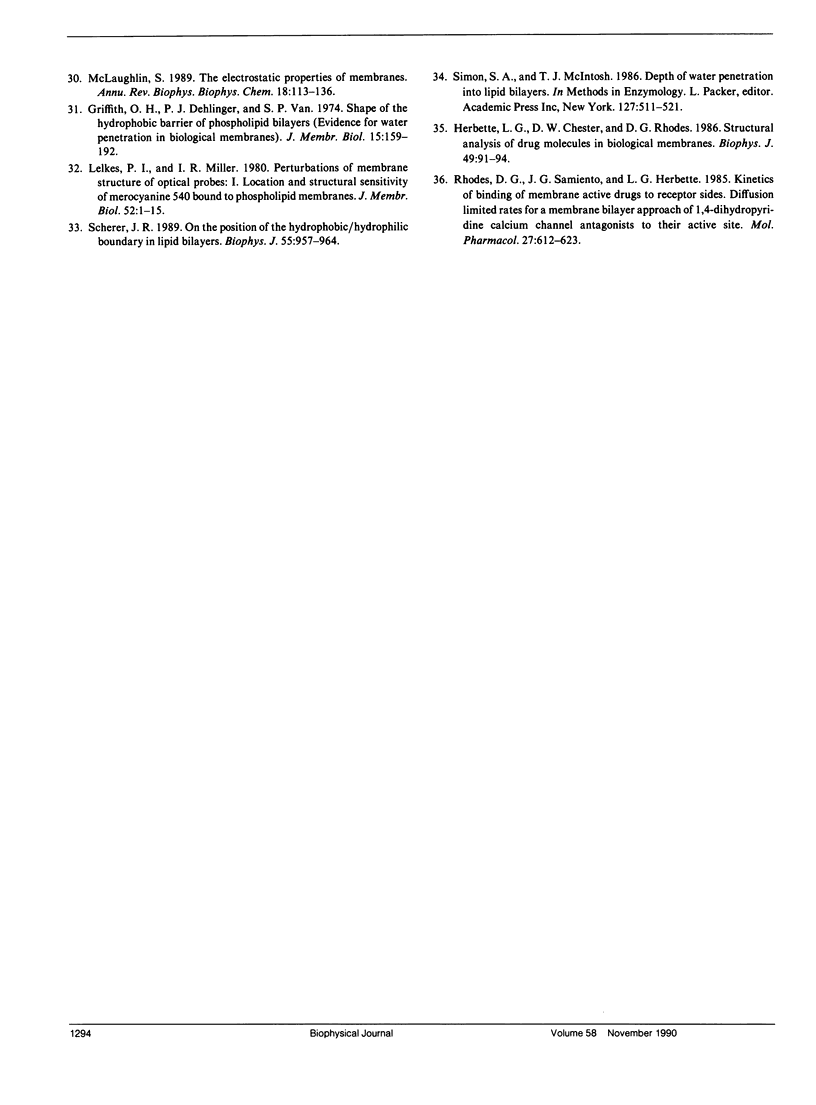
Images in this article
Selected References
These references are in PubMed. This may not be the complete list of references from this article.
- Andersen O. S., Feldberg S., Nakadomari H., Levy S., McLaughlin S. Electrostatic interactions among hydrophobic ions in lipid bilayer membranes. Biophys J. 1978 Jan;21(1):35–70. doi: 10.1016/S0006-3495(78)85507-6. [DOI] [PMC free article] [PubMed] [Google Scholar]
- Bentz J., Nir S. Cation binding to membranes: competition between mono-, di- and trivalent cations. Bull Math Biol. 1980;42(2):191–220. doi: 10.1007/BF02464638. [DOI] [PubMed] [Google Scholar]
- Brown R. H., Jr Membrane surface charge: discrete and uniform modelling. Prog Biophys Mol Biol. 1974;28:341–370. doi: 10.1016/0079-6107(74)90021-2. [DOI] [PubMed] [Google Scholar]
- Ellena J. F., Dominey R. N., Archer S. J., Xu Z. C., Cafiso D. S. Localization of hydrophobic ions in phospholipid bilayers using 1H nuclear Overhauser effect spectroscopy. Biochemistry. 1987 Jul 14;26(14):4584–4592. doi: 10.1021/bi00388a062. [DOI] [PubMed] [Google Scholar]
- Flewelling R. F., Hubbell W. L. Hydrophobic ion interactions with membranes. Thermodynamic analysis of tetraphenylphosphonium binding to vesicles. Biophys J. 1986 Feb;49(2):531–540. doi: 10.1016/S0006-3495(86)83663-3. [DOI] [PMC free article] [PubMed] [Google Scholar]
- Flewelling R. F., Hubbell W. L. The membrane dipole potential in a total membrane potential model. Applications to hydrophobic ion interactions with membranes. Biophys J. 1986 Feb;49(2):541–552. doi: 10.1016/S0006-3495(86)83664-5. [DOI] [PMC free article] [PubMed] [Google Scholar]
- Griffith O. H., Dehlinger P. J., Van S. P. Shape of the hydrophobic barrier of phospholipid bilayers (evidence for water penetration in biological membranes). J Membr Biol. 1974;15(2):159–192. doi: 10.1007/BF01870086. [DOI] [PubMed] [Google Scholar]
- Herbette L. G., Chester D. W., Rhodes D. G. Structural analysis of drug molecules in biological membranes. Biophys J. 1986 Jan;49(1):91–94. doi: 10.1016/S0006-3495(86)83605-0. [DOI] [PMC free article] [PubMed] [Google Scholar]
- Lelkes P. I., Miller I. R. Perturbations of membrane structure by optical probes: I. Location and structural sensitivity of merocyanine 540 bound to phospholipid membranes. J Membr Biol. 1980 Jan 31;52(1):1–15. doi: 10.1007/BF01869001. [DOI] [PubMed] [Google Scholar]
- Lis L. J., McAlister M., Fuller N., Rand R. P., Parsegian V. A. Interactions between neutral phospholipid bilayer membranes. Biophys J. 1982 Mar;37(3):657–665. [PMC free article] [PubMed] [Google Scholar]
- Maher P., Singer S. J. Structural changes in membranes produced by the binding of small amphipathic molecules. Biochemistry. 1984 Jan 17;23(2):232–240. doi: 10.1021/bi00297a010. [DOI] [PubMed] [Google Scholar]
- McLaughlin S. G., Dilger J. P. Transport of protons across membranes by weak acids. Physiol Rev. 1980 Jul;60(3):825–863. doi: 10.1152/physrev.1980.60.3.825. [DOI] [PubMed] [Google Scholar]
- McLaughlin S., Harary H. The hydrophobic adsorption of charged molecules to bilayer membranes: a test of the applicability of the stern equation. Biochemistry. 1976 May 4;15(9):1941–1948. doi: 10.1021/bi00654a023. [DOI] [PubMed] [Google Scholar]
- McLaughlin S., Mulrine N., Gresalfi T., Vaio G., McLaughlin A. Adsorption of divalent cations to bilayer membranes containing phosphatidylserine. J Gen Physiol. 1981 Apr;77(4):445–473. doi: 10.1085/jgp.77.4.445. [DOI] [PMC free article] [PubMed] [Google Scholar]
- McLaughlin S. The electrostatic properties of membranes. Annu Rev Biophys Biophys Chem. 1989;18:113–136. doi: 10.1146/annurev.bb.18.060189.000553. [DOI] [PubMed] [Google Scholar]
- Rhodes D. G., Sarmiento J. G., Herbette L. G. Kinetics of binding of membrane-active drugs to receptor sites. Diffusion-limited rates for a membrane bilayer approach of 1,4-dihydropyridine calcium channel antagonists to their active site. Mol Pharmacol. 1985 Jun;27(6):612–623. [PubMed] [Google Scholar]
- Scherer J. R. On the position of the hydro-phobic/philic boundary in lipid bilayers. Biophys J. 1989 May;55(5):957–964. doi: 10.1016/S0006-3495(89)82894-2. [DOI] [PMC free article] [PubMed] [Google Scholar]
- Seelig J., Seelig A. Lipid conformation in model membranes and biological membranes. Q Rev Biophys. 1980 Feb;13(1):19–61. doi: 10.1017/s0033583500000305. [DOI] [PubMed] [Google Scholar]
- Simon S. A., McIntosh T. J. Depth of water penetration into lipid bilayers. Methods Enzymol. 1986;127:511–521. doi: 10.1016/0076-6879(86)27041-x. [DOI] [PubMed] [Google Scholar]
- Smejtek P., Barstad A. W., Hsu K. Dielectric properties of adsorption/ionization site of pentachlorophenol in lipid membranes. Biochim Biophys Acta. 1987 Aug 7;902(1):109–127. doi: 10.1016/0005-2736(87)90141-6. [DOI] [PubMed] [Google Scholar]
- Smejtek P., Barstad A. W., Wang S. Pentachlorophenol-induced change of zeta-potential and gel-to-fluid transition temperature in model lecithin membranes. Chem Biol Interact. 1989;71(1):37–61. doi: 10.1016/0009-2797(89)90089-6. [DOI] [PubMed] [Google Scholar]
- Smejtek P., Hsu K., Perman W. H. Electrical conductivity in lipid bilayer membranes induced by pentachlorophenol. Biophys J. 1976 Apr;16(4):319–336. doi: 10.1016/S0006-3495(76)85691-3. [DOI] [PMC free article] [PubMed] [Google Scholar]
- Smejtek P., Paulis-Illangasekare M. Modification of ion transport in lipid bilayer membranes in the presence of 2,4-dichlorophenoxyacetic acid. II. Suppression of tetraphenylborate conductance and changes of interfacial potentials. Biophys J. 1979 Jun;26(3):467–487. doi: 10.1016/S0006-3495(79)85265-0. [DOI] [PMC free article] [PubMed] [Google Scholar]
- Smejtek P., Wang S. R., Barstad A. W. Adsorption of ionized and neutral pentachlorophenol to phosphatidylcholine membranes. Biochim Biophys Acta. 1987 Nov 27;905(1):213–221. doi: 10.1016/0005-2736(87)90025-3. [DOI] [PubMed] [Google Scholar]
- Tsien R. Y. A virial expansion for discrete charges buried in a membrane. Biophys J. 1978 Nov;24(2):561–567. doi: 10.1016/S0006-3495(78)85402-2. [DOI] [PMC free article] [PubMed] [Google Scholar]
- Tsien R. Y., Hladky S. B. Ion repulsion within membranes. Biophys J. 1982 Jul;39(1):49–56. doi: 10.1016/S0006-3495(82)84489-5. [DOI] [PMC free article] [PubMed] [Google Scholar]
- WEINBACH E. C., GARBUS J. THE INTERACTION OF UNCOUPLING PHENOLS WITH MITOCHONDRIA AND WITH MITOCHONDRIAL PROTEIN. J Biol Chem. 1965 Apr;240:1811–1819. [PubMed] [Google Scholar]
- Wang C. C., Bruner L. J. Evidence for a discrete charge effect within lipid bilayer membranes. Biophys J. 1978 Dec;24(3):749–764. doi: 10.1016/S0006-3495(78)85418-6. [DOI] [PMC free article] [PubMed] [Google Scholar]
- Wiener M. C., Suter R. M., Nagle J. F. Structure of the fully hydrated gel phase of dipalmitoylphosphatidylcholine. Biophys J. 1989 Feb;55(2):315–325. doi: 10.1016/S0006-3495(89)82807-3. [DOI] [PMC free article] [PubMed] [Google Scholar]