Abstract
Potassium accumulation associated with outward membrane potassium current was investigated experimentally in myelinated fibers and analyzed in terms of two models-three-compartment and diffusion in an unstirred layer. In the myelinated fibers, as in squid giant axons, the three-compartment model satisfactorily describes potassium accumulation. Within this framework the average space thickness, theta, in frog was 5,900 +/- 700 A, while the permeability coefficient of the external barrier, PK, was (1.5 +/- 0.1) X 10(-2) cm/s. The model of ionic diffusion in an unstirred aqueous layer adjacent to the axolemma, as an alternative explanation for ion accumulation, was also consistent with the experimental data, provided that D, the diffusion constant, was (1.8 +/- 0.2) X 10(-6) cm/s and l, the unstirred layer thickness, was 1.4 +/- 0.1 micron, i.e., similar to the depth of the nodal gap. An empirical equation relating the extent of potassium accumulation to the amplitude and duration of depolarization is given.
Full text
PDF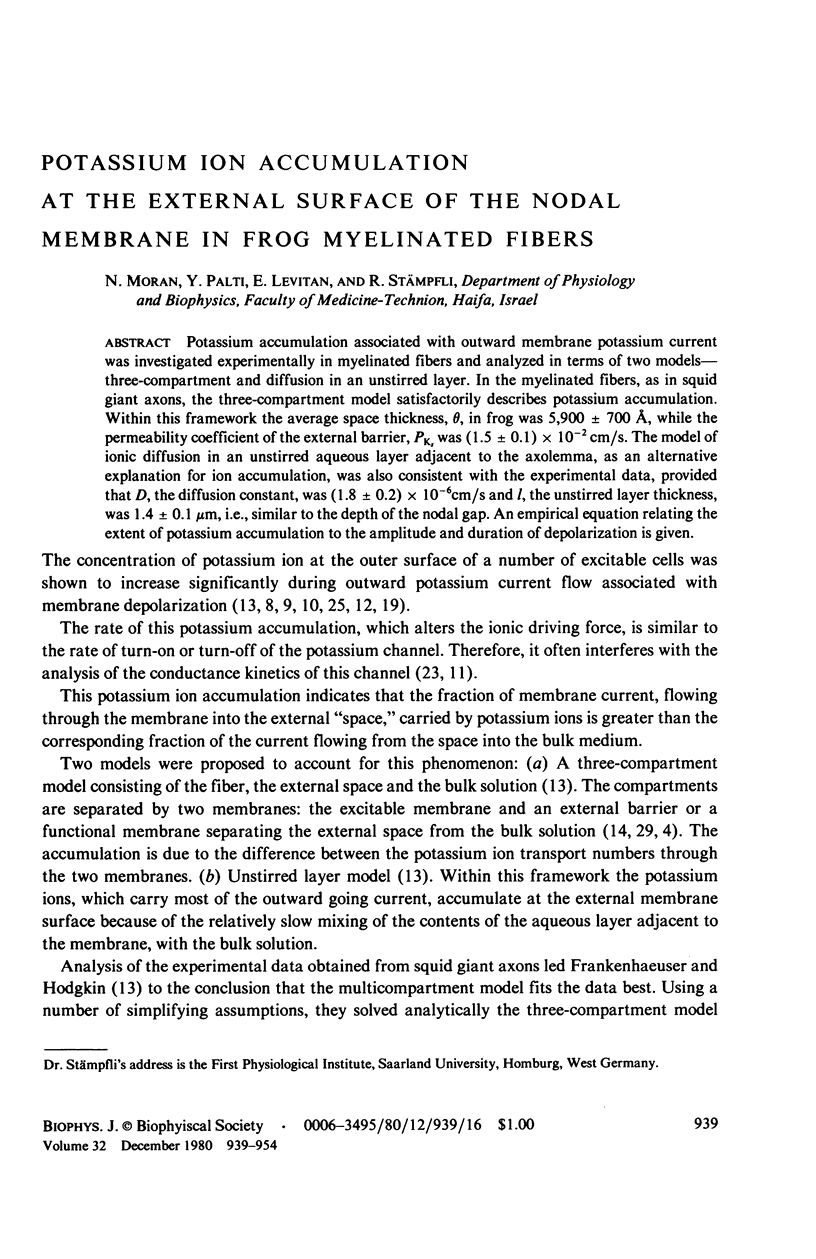
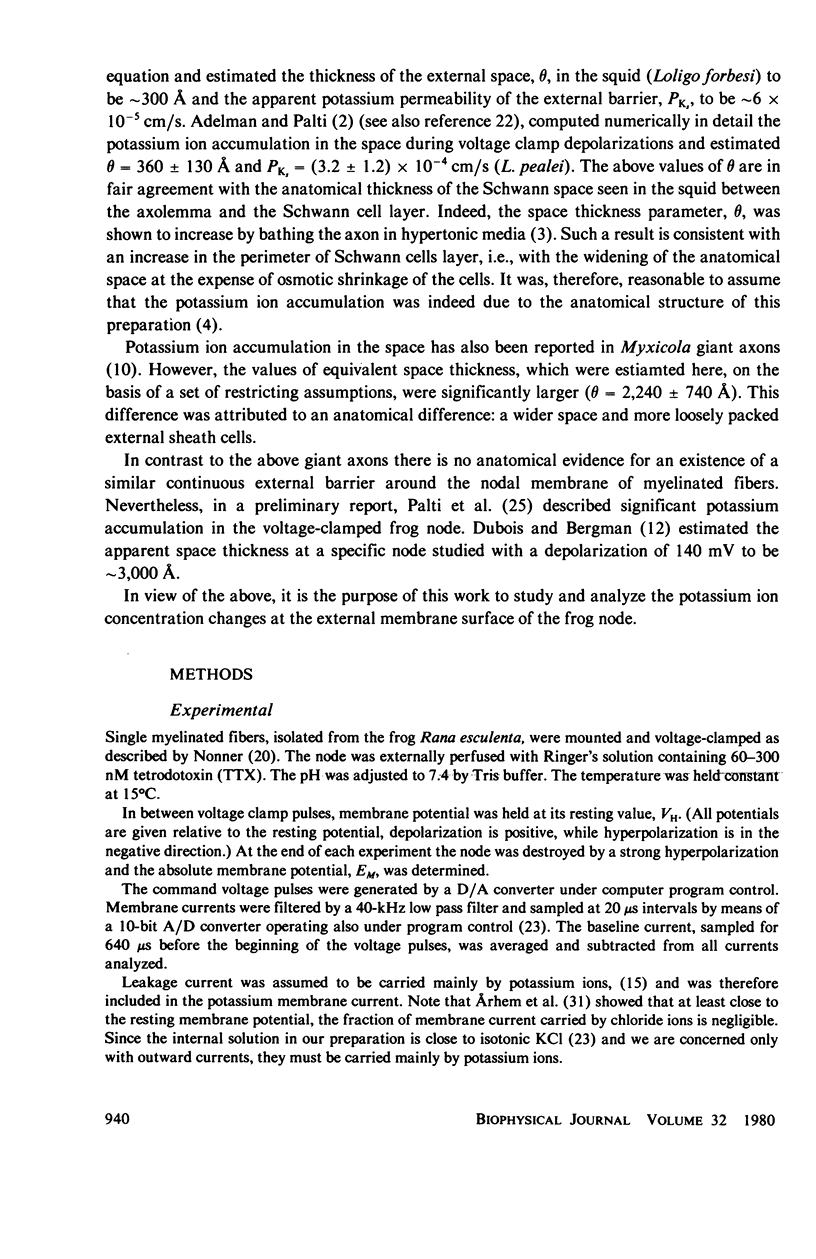
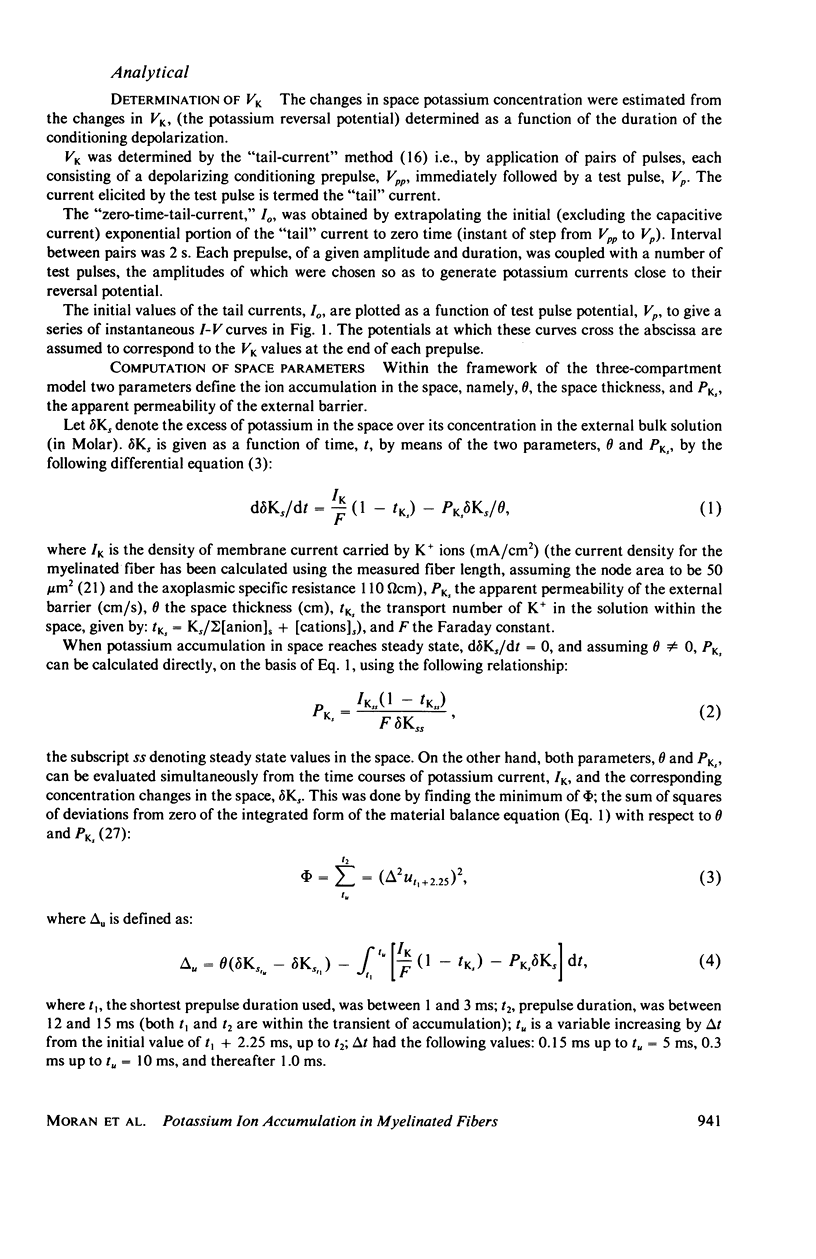
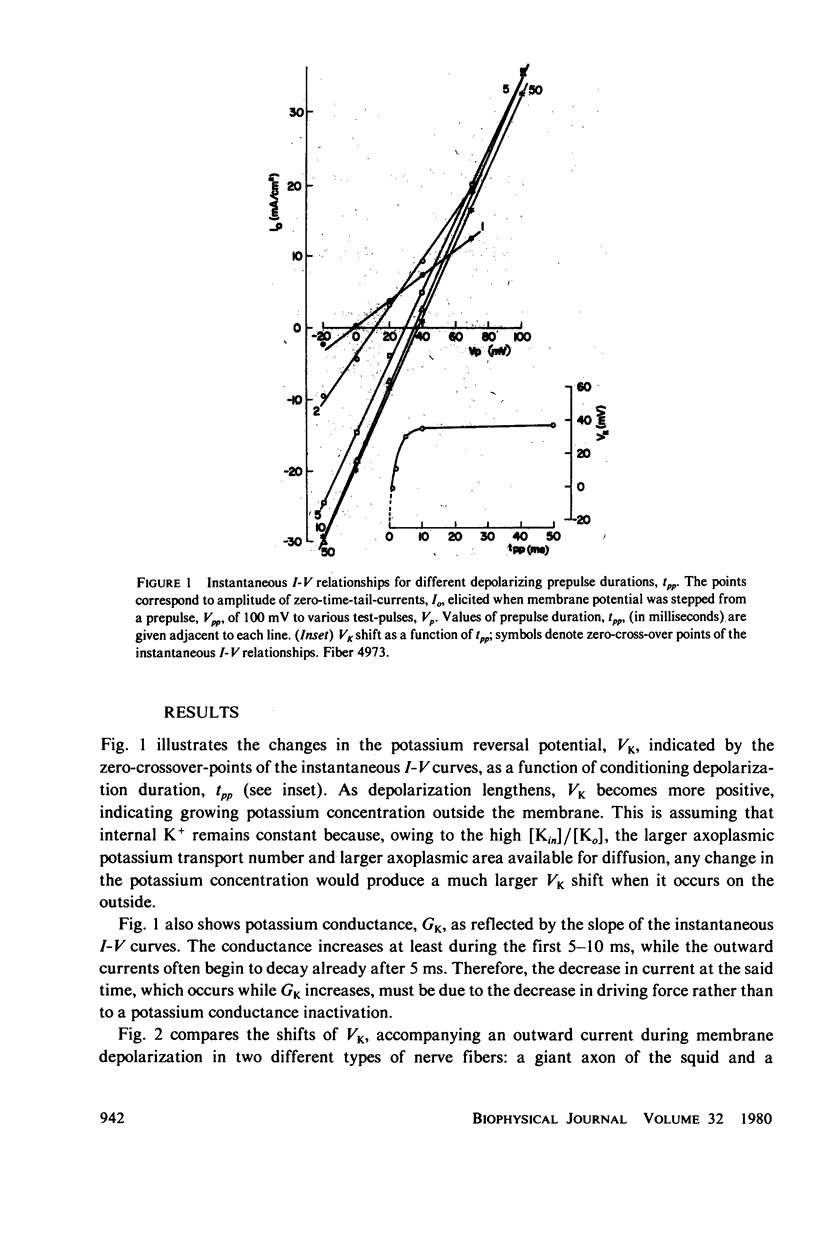
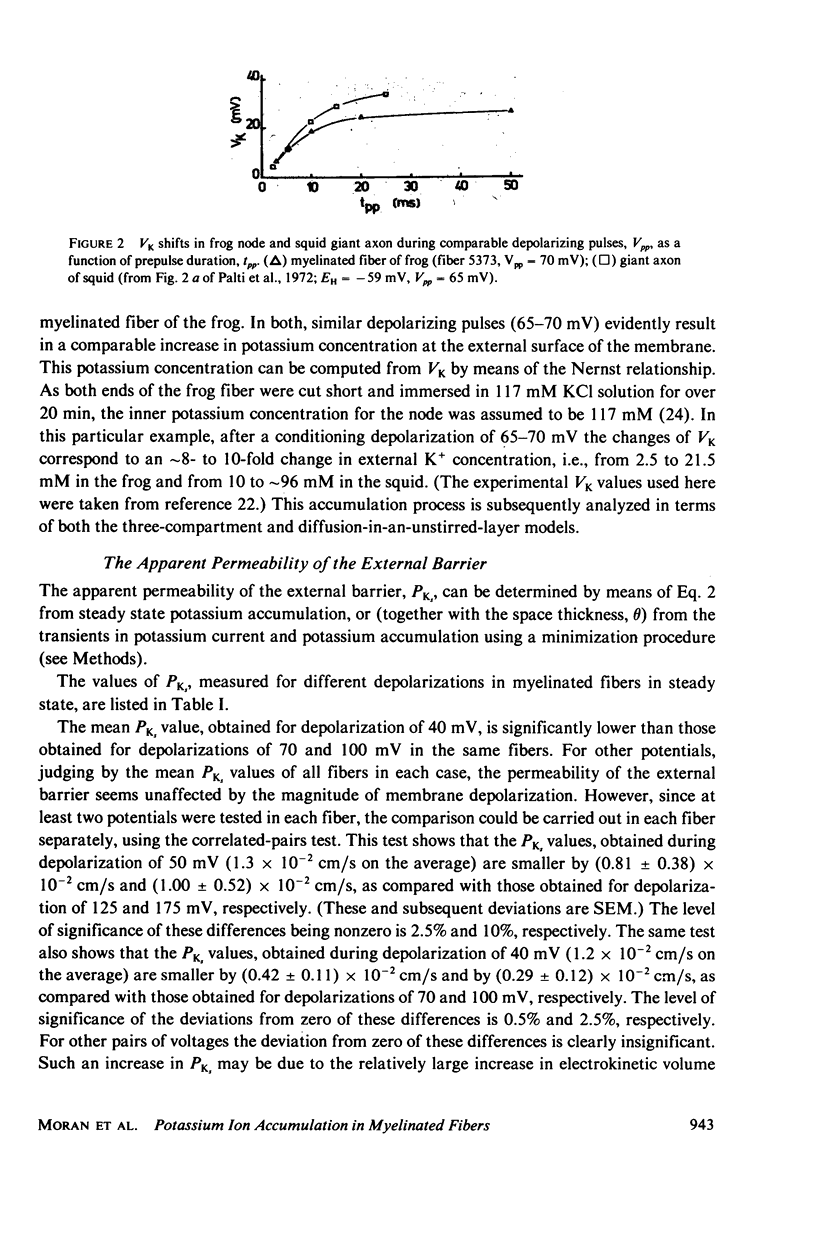
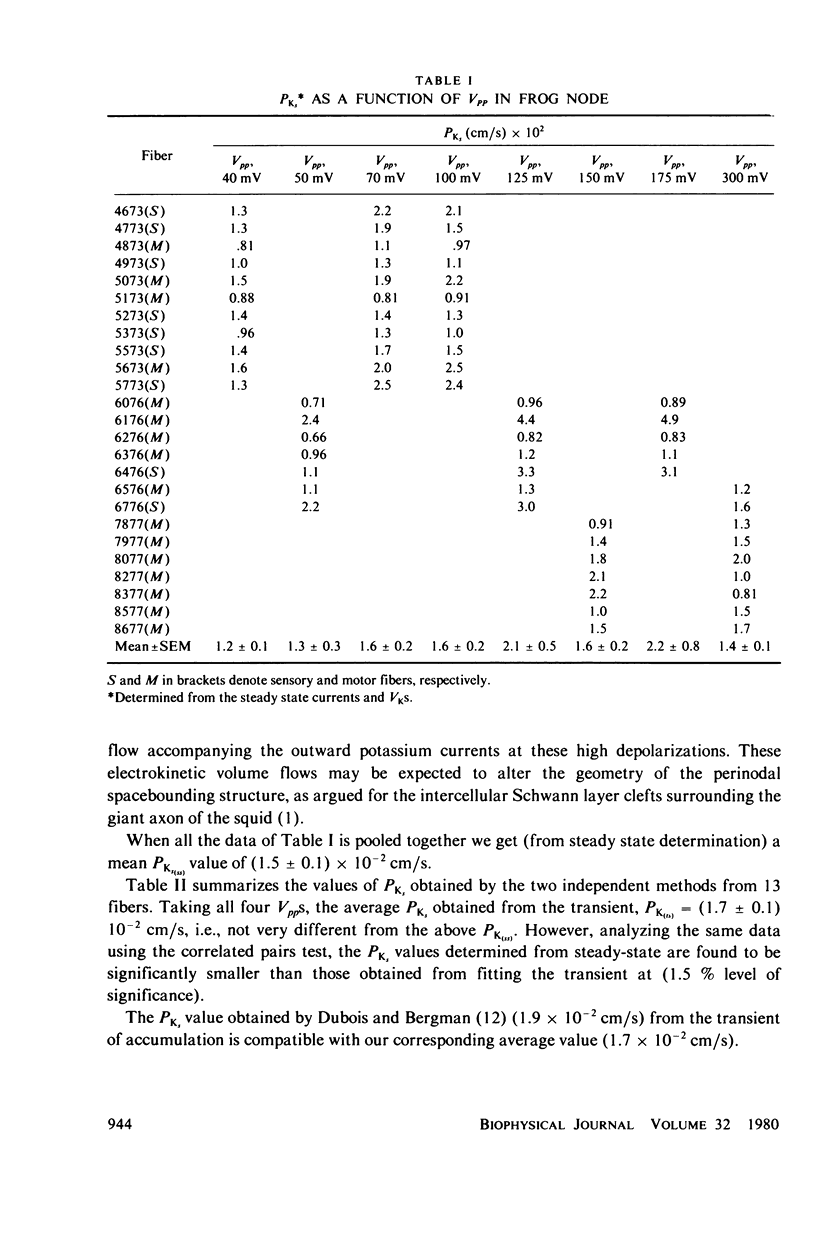
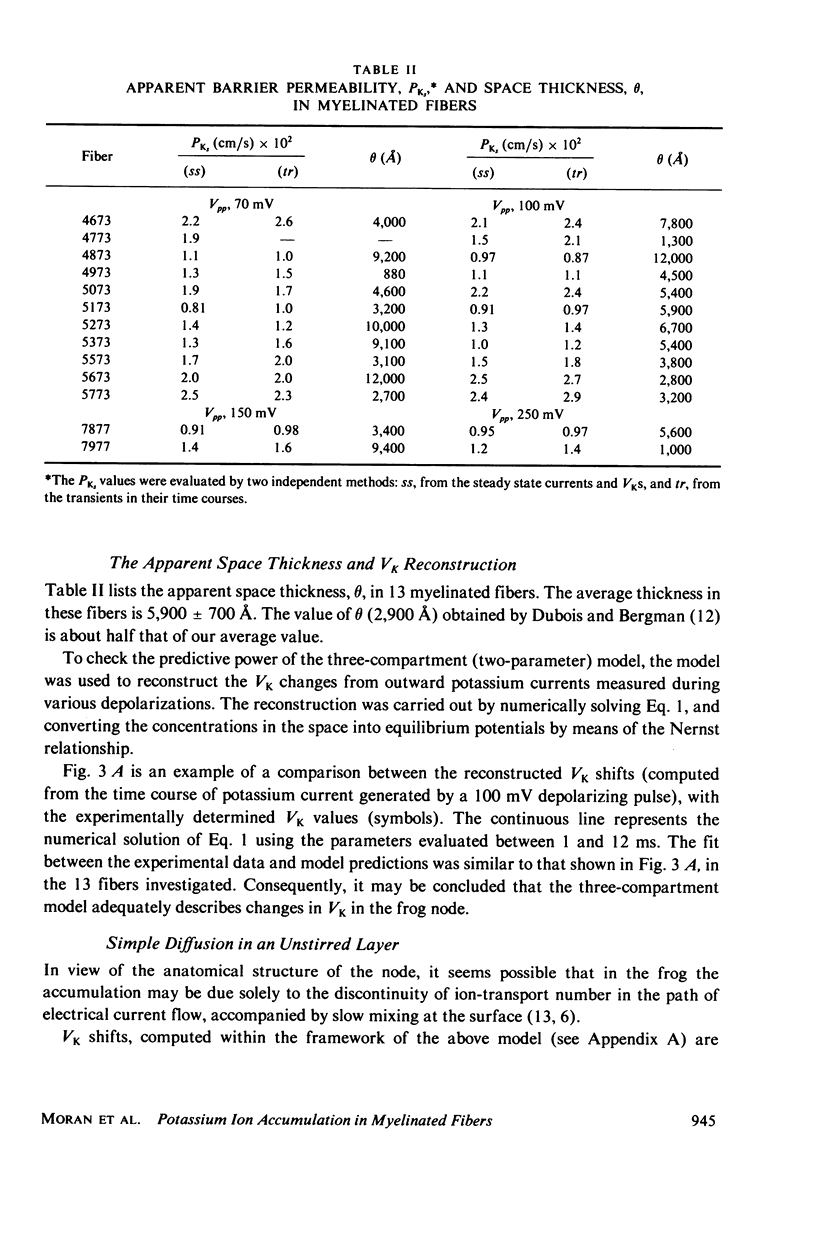
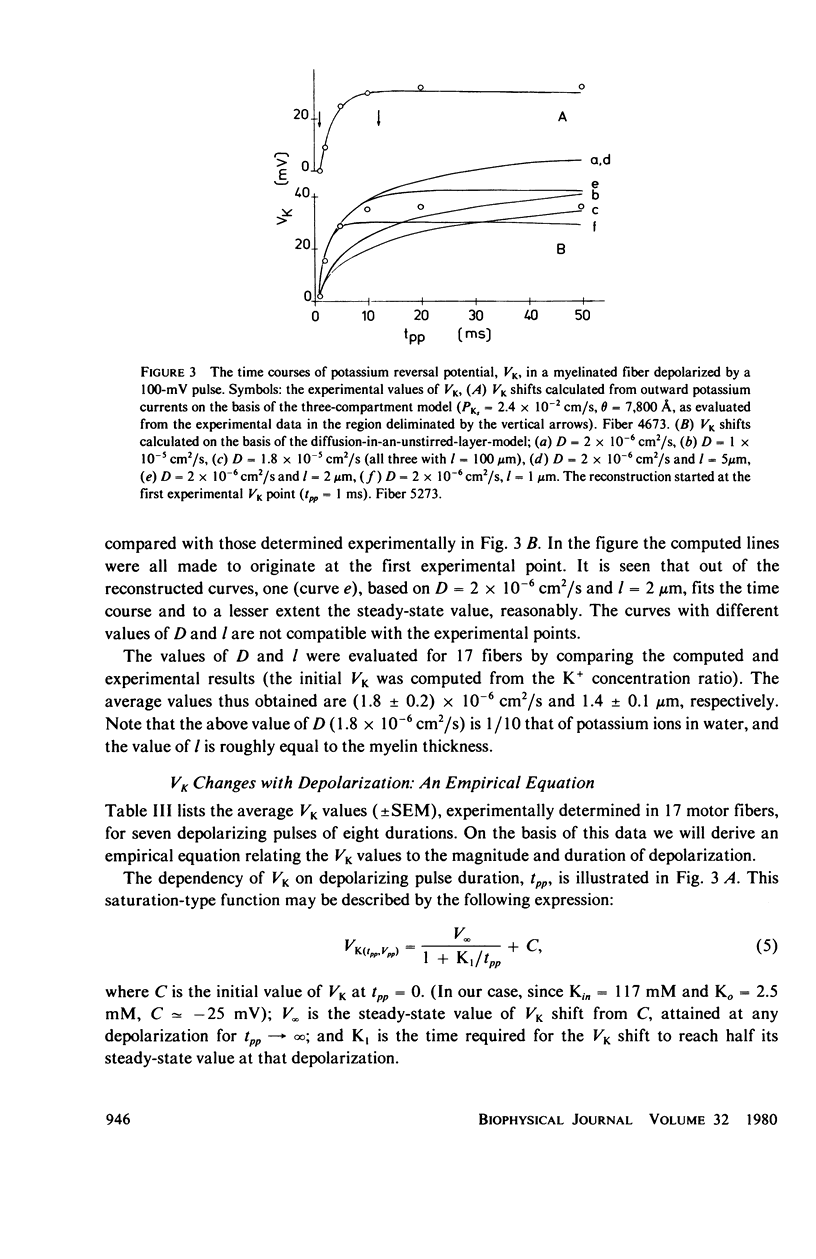
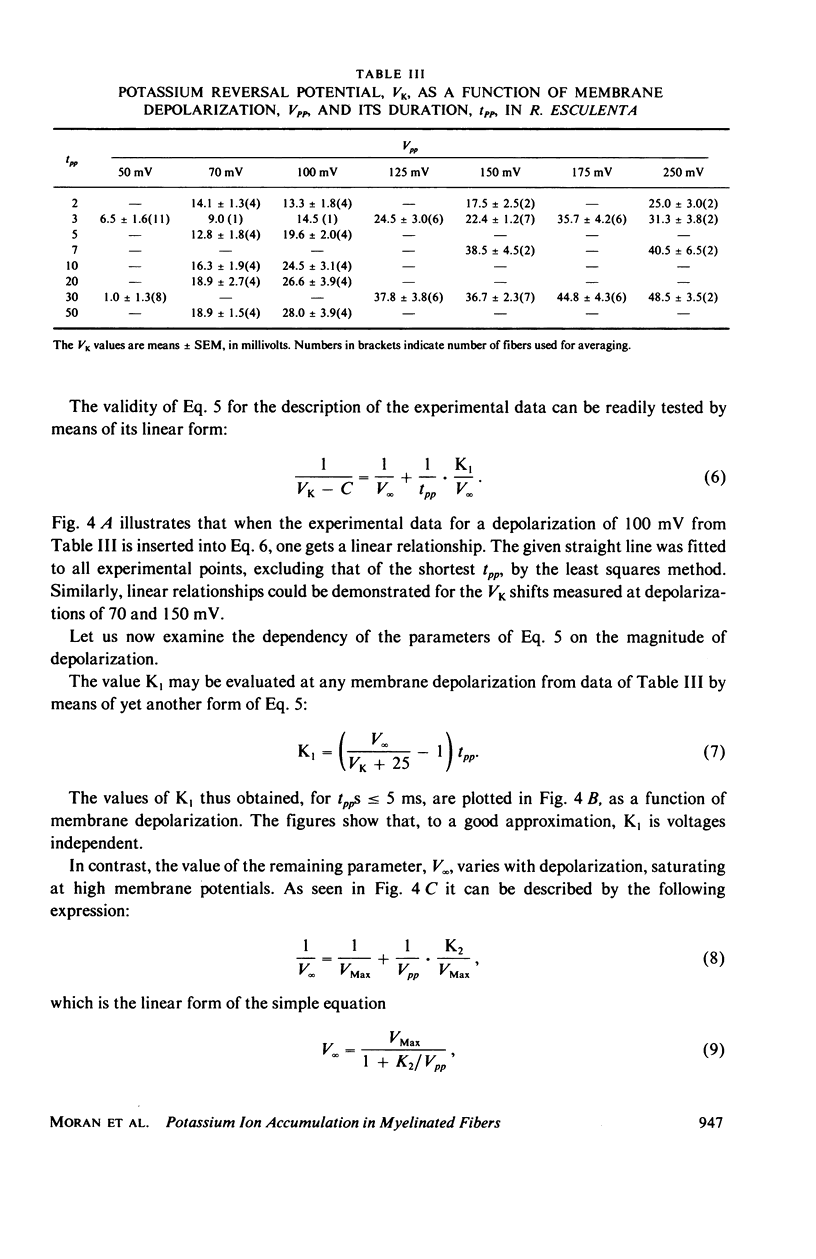
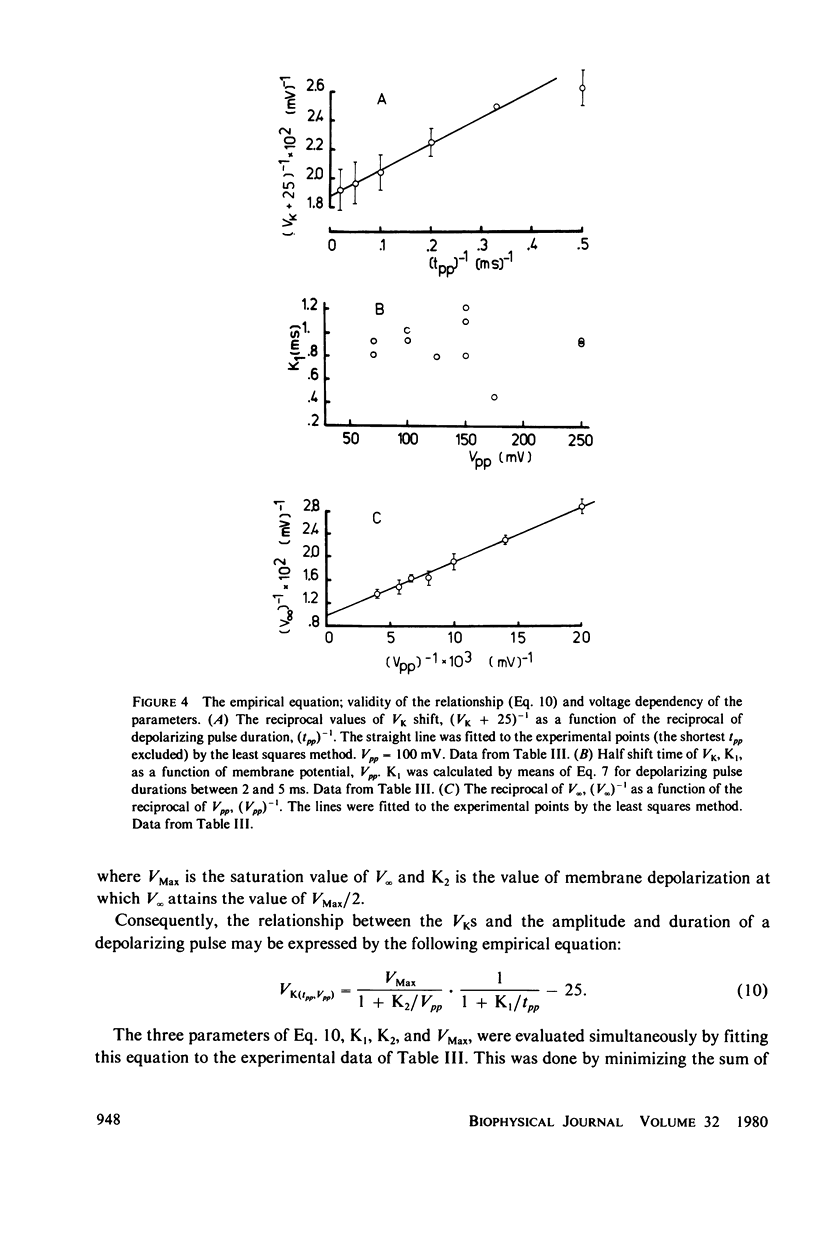
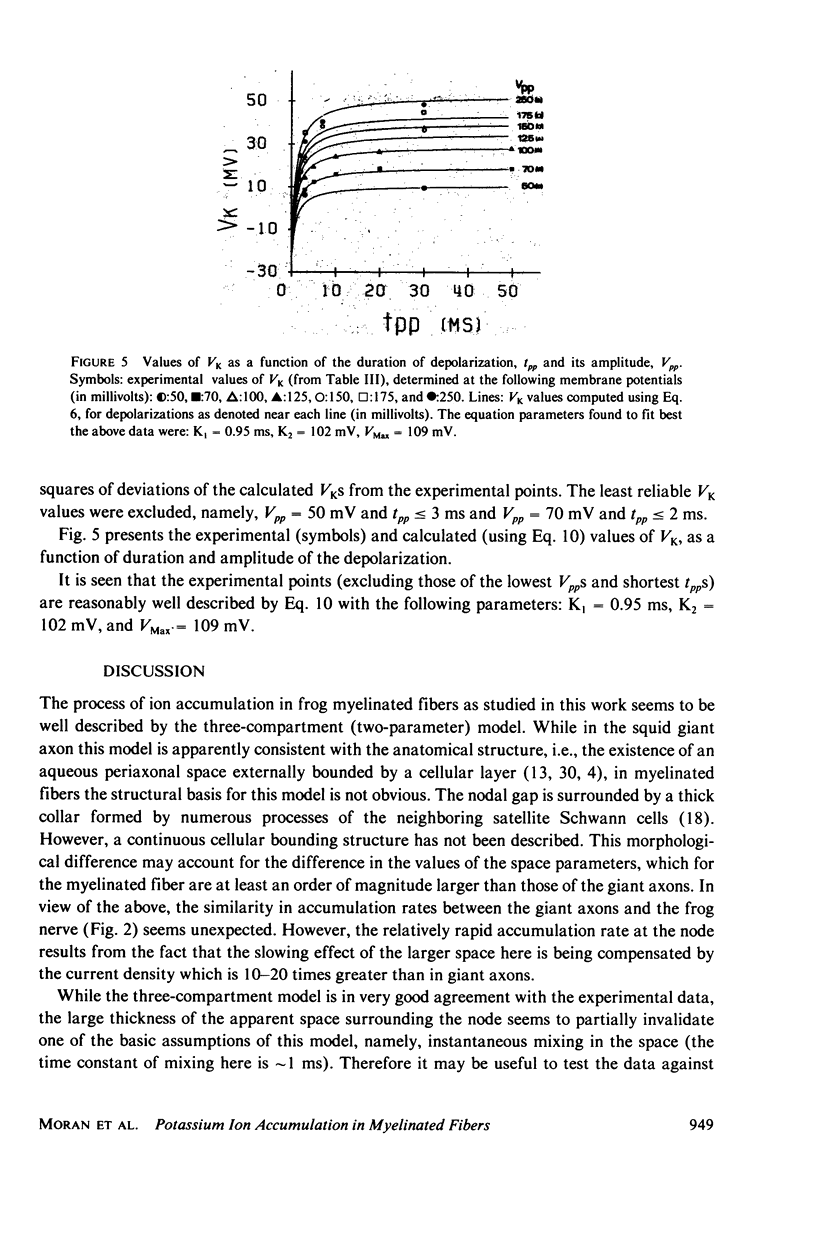
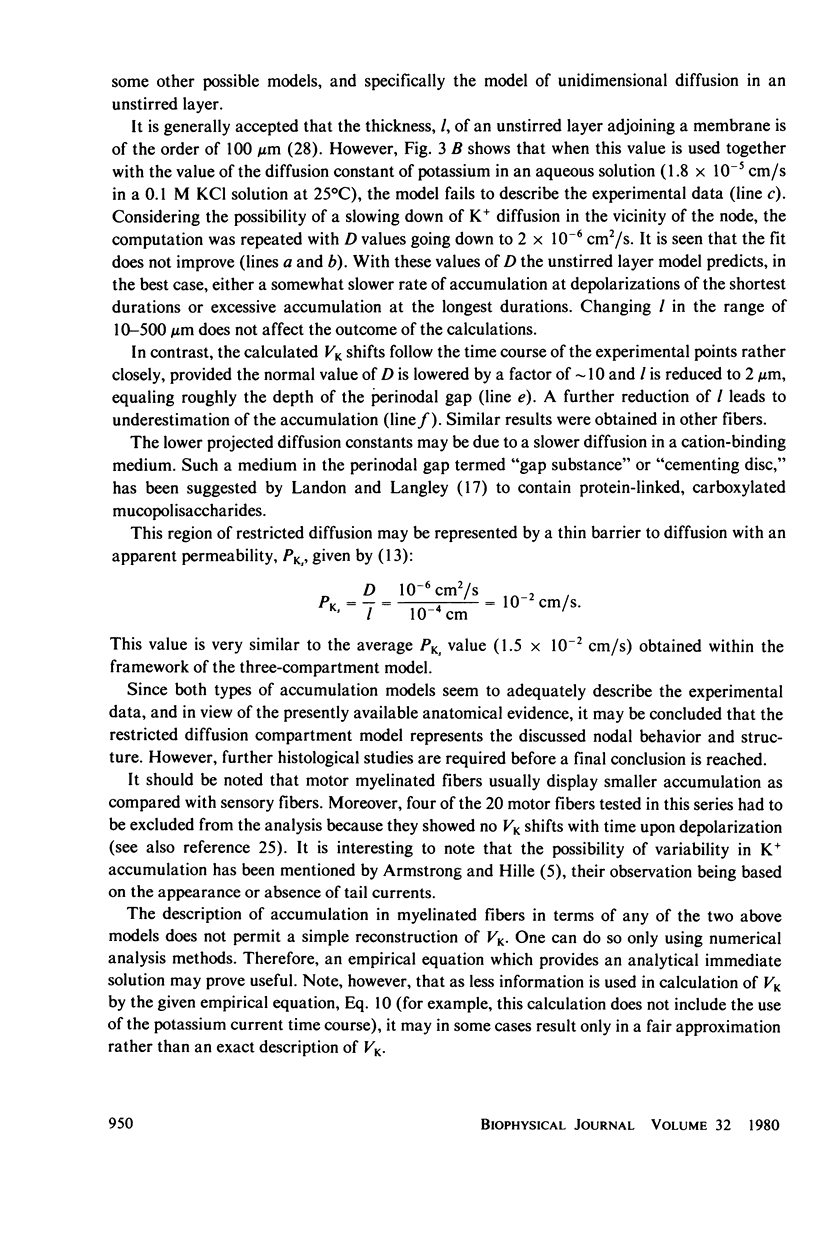
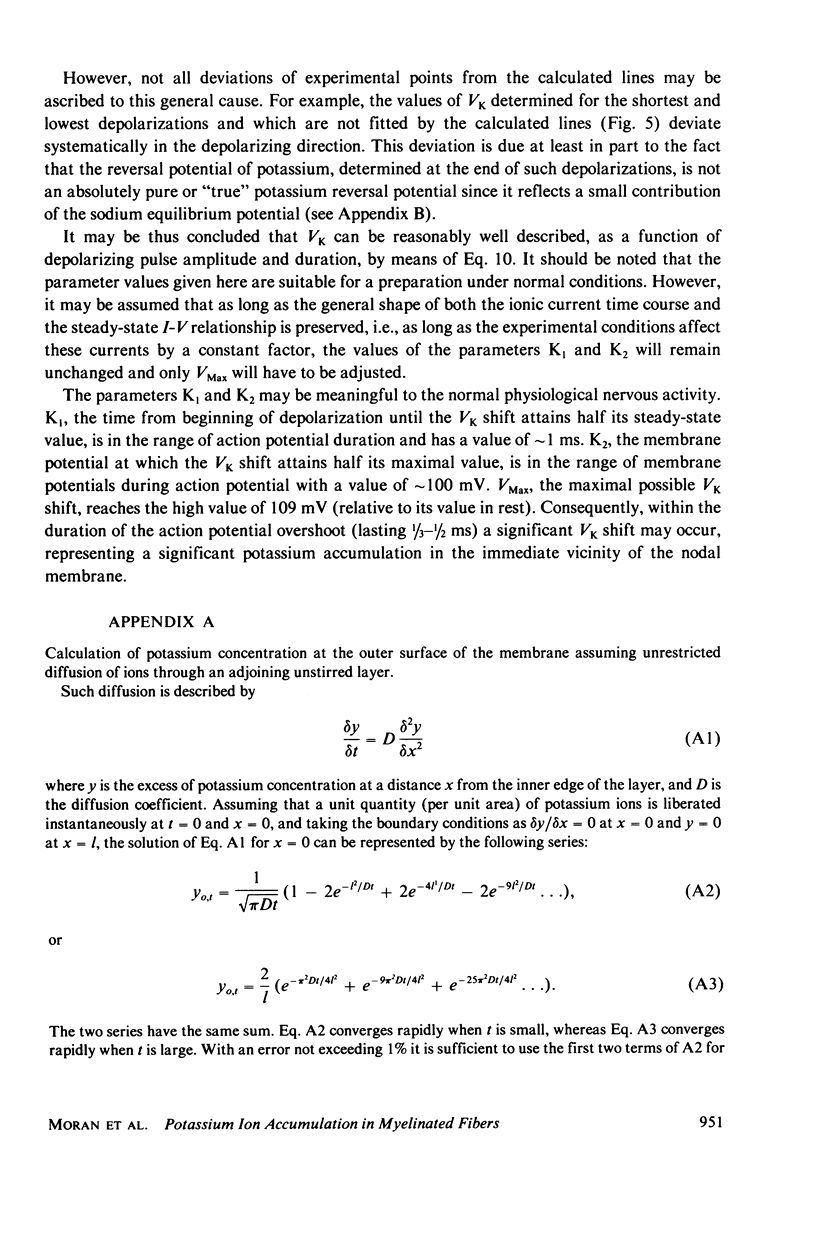
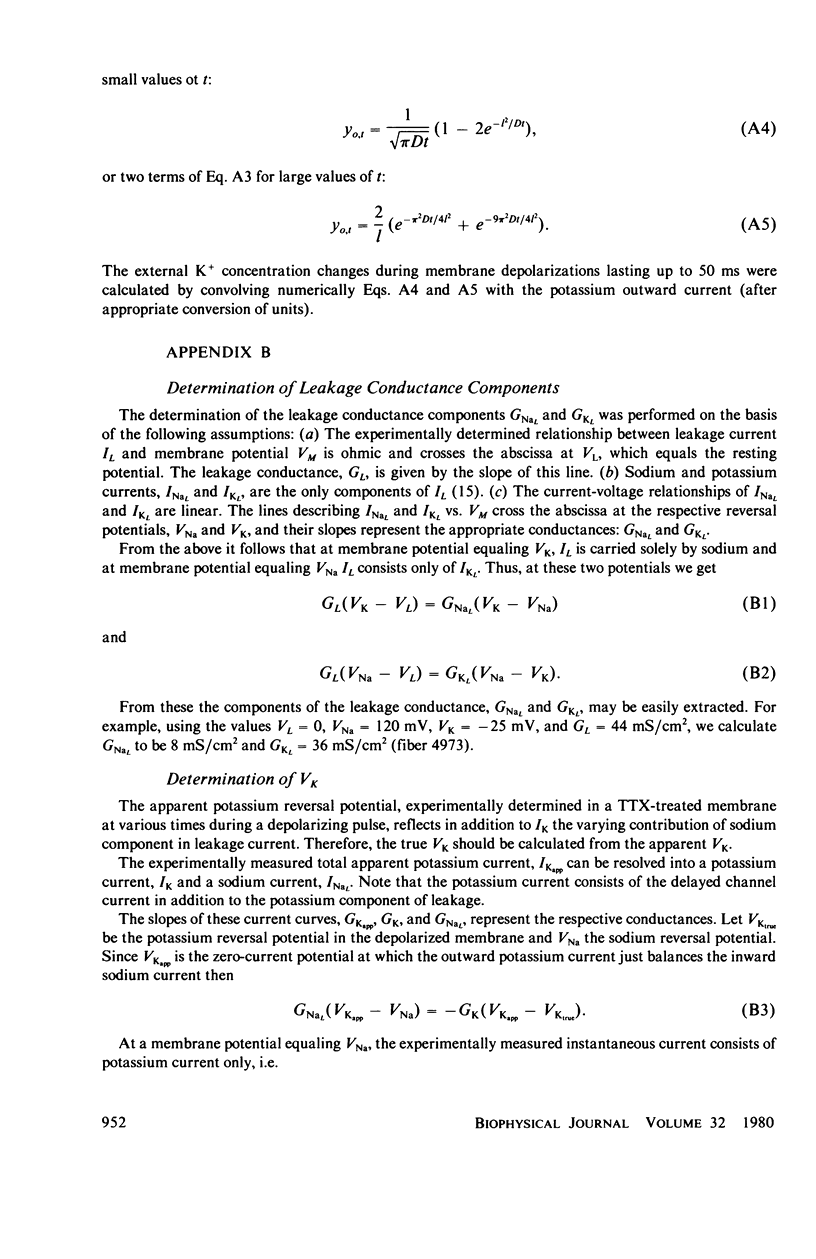
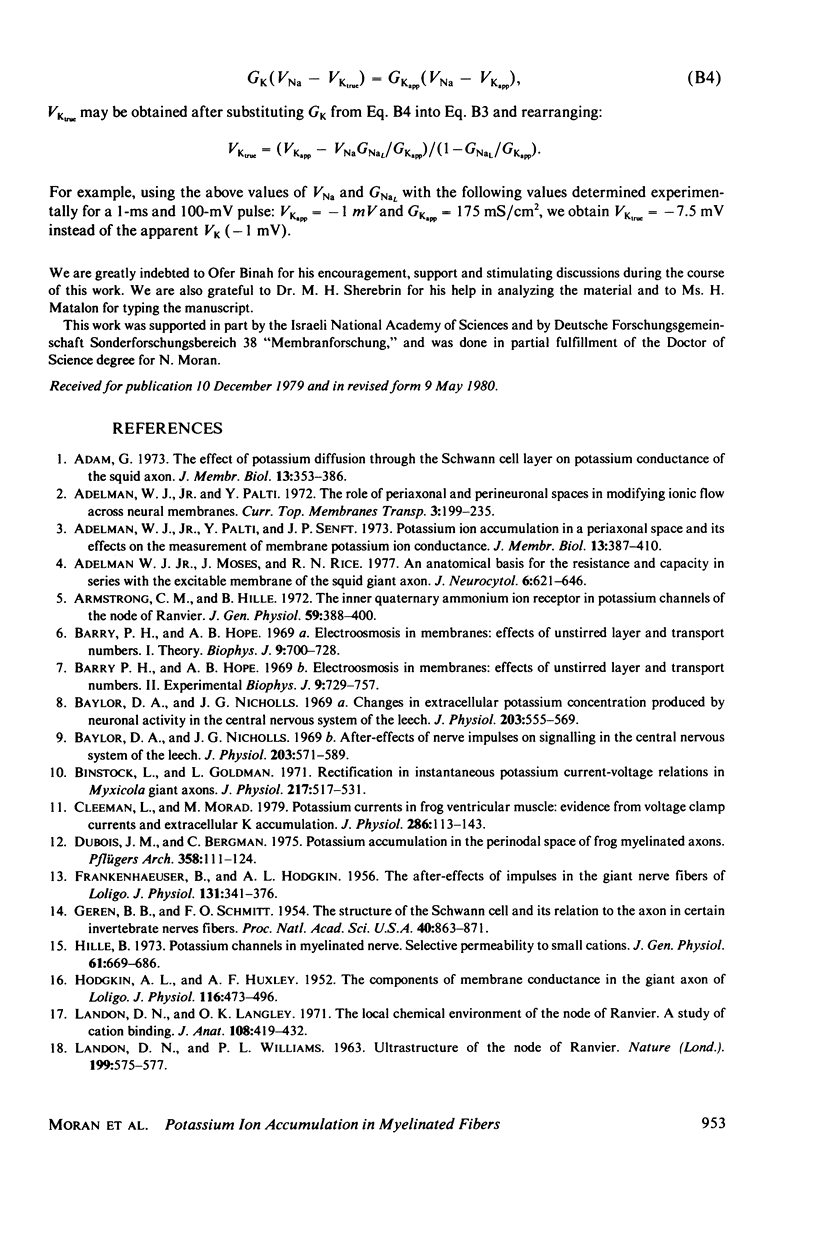
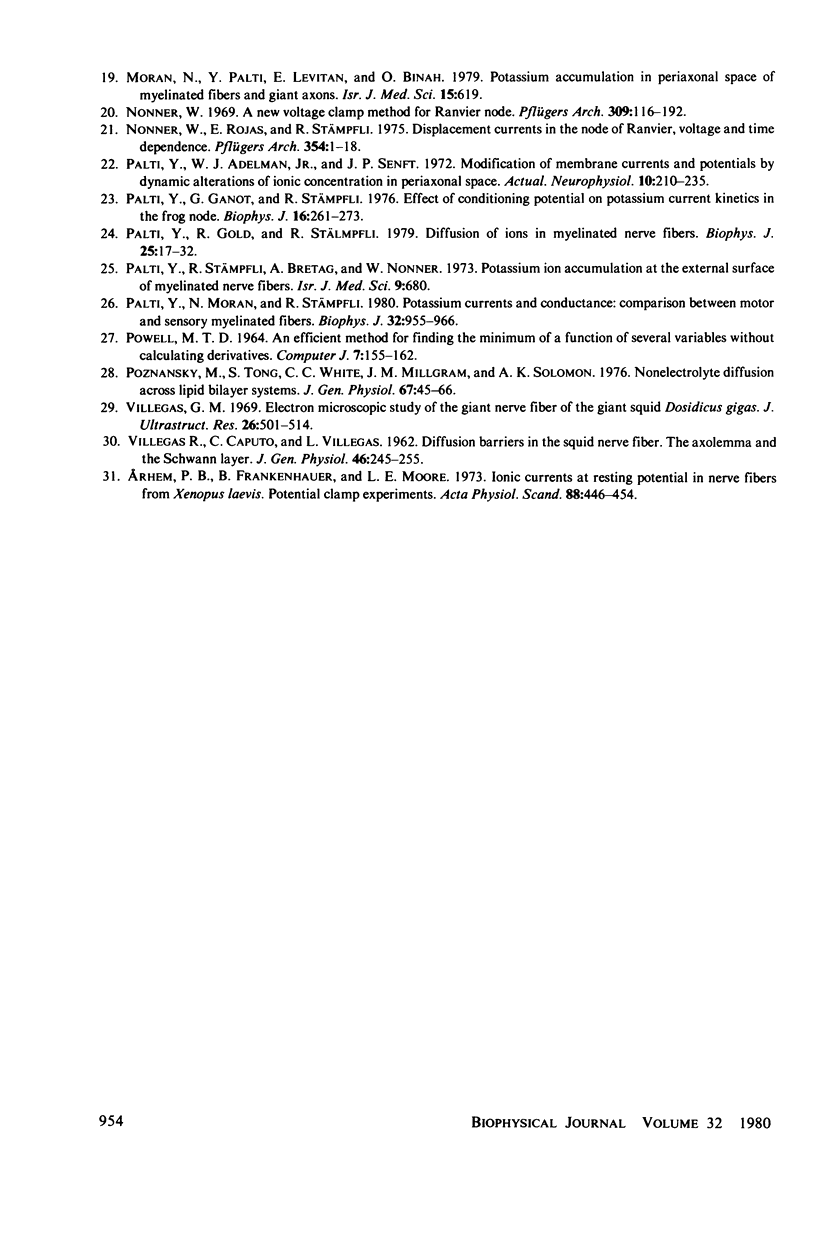
Selected References
These references are in PubMed. This may not be the complete list of references from this article.
- Adam G. The effect of potassium diffusion through the Schwann cell layer on potassium conductance of the squid axon. J Membr Biol. 1973 Nov 8;13(4):353–386. doi: 10.1007/BF01868236. [DOI] [PubMed] [Google Scholar]
- Adelman W. J., Jr, Moses J., Rive R. V. An anatomical basis for the resistance and capacitance in series with excitable membrane of the squid giant axon. J Neurocytol. 1977 Dec;6(6):621–646. doi: 10.1007/BF01176377. [DOI] [PubMed] [Google Scholar]
- Adelman W. J., Jr, Palti Y., Senft J. P. Potassium ion accumulation in a periaxonal space and its effect on the measurement of membrane potassium ion conductance. J Membr Biol. 1973 Nov 8;13(4):387–410. doi: 10.1007/BF01868237. [DOI] [PubMed] [Google Scholar]
- Arhem P., Frankenhaeuser B., Moore L. E. Ionic currents at resting potential in nerve fibres from Xenopus laevis. Potential clamp experiments. Acta Physiol Scand. 1973 Aug;88(4):446–454. doi: 10.1111/j.1748-1716.1973.tb05474.x. [DOI] [PubMed] [Google Scholar]
- Armstrong C. M., Hille B. The inner quaternary ammonium ion receptor in potassium channels of the node of Ranvier. J Gen Physiol. 1972 Apr;59(4):388–400. doi: 10.1085/jgp.59.4.388. [DOI] [PMC free article] [PubMed] [Google Scholar]
- Barry P. H., Hope A. B. Electroosmosis in membranes: effects of unstirred layers and transport numbers. I. Theory. Biophys J. 1969 May;9(5):700–728. doi: 10.1016/S0006-3495(69)86413-1. [DOI] [PMC free article] [PubMed] [Google Scholar]
- Barry P. H., Hope A. B. Electroosmosis in membranes: effects of unstirred layers and transport numbers. II. Experimental. Biophys J. 1969 May;9(5):729–757. doi: 10.1016/S0006-3495(69)86414-3. [DOI] [PMC free article] [PubMed] [Google Scholar]
- Baylor D. A., Nicholls J. G. After-effects of nerve impulses on signalling in the central nervous system of the leech. J Physiol. 1969 Aug;203(3):571–589. doi: 10.1113/jphysiol.1969.sp008880. [DOI] [PMC free article] [PubMed] [Google Scholar]
- Baylor D. A., Nicholls J. G. Changes in extracellular potassium concentration produced by neuronal activity in the central nervous system of the leech. J Physiol. 1969 Aug;203(3):555–569. doi: 10.1113/jphysiol.1969.sp008879. [DOI] [PMC free article] [PubMed] [Google Scholar]
- Binstock L., Goldman L. Rectification in instantaneous potassium current-voltage relations in Myxicola giant axons. J Physiol. 1971 Sep;217(3):517–531. doi: 10.1113/jphysiol.1971.sp009583. [DOI] [PMC free article] [PubMed] [Google Scholar]
- Cleemann L., Morad M. Potassium currents in frog ventricular muscle: evidence from voltage clamp currents and extracellular K accumulation. J Physiol. 1979 Jan;286:113–143. doi: 10.1113/jphysiol.1979.sp012609. [DOI] [PMC free article] [PubMed] [Google Scholar]
- Dubois J. M., Bergman C. Potassium accumulation in the perinodal space of frog myelinated axons. Pflugers Arch. 1975 Jul 21;358(2):111–124. doi: 10.1007/BF00583922. [DOI] [PubMed] [Google Scholar]
- FRANKENHAEUSER B., HODGKIN A. L. The after-effects of impulses in the giant nerve fibres of Loligo. J Physiol. 1956 Feb 28;131(2):341–376. doi: 10.1113/jphysiol.1956.sp005467. [DOI] [PMC free article] [PubMed] [Google Scholar]
- Geren B. B., Schmitt F. O. THE STRUCTURE OF THE SCHWANN CELL AND ITS RELATION TO THE AXON IN CERTAIN INVERTEBRATE NERVE FIBERS. Proc Natl Acad Sci U S A. 1954 Sep;40(9):863–870. doi: 10.1073/pnas.40.9.863. [DOI] [PMC free article] [PubMed] [Google Scholar]
- HODGKIN A. L., HUXLEY A. F. The components of membrane conductance in the giant axon of Loligo. J Physiol. 1952 Apr;116(4):473–496. doi: 10.1113/jphysiol.1952.sp004718. [DOI] [PMC free article] [PubMed] [Google Scholar]
- Hille B. Potassium channels in myelinated nerve. Selective permeability to small cations. J Gen Physiol. 1973 Jun;61(6):669–686. doi: 10.1085/jgp.61.6.669. [DOI] [PMC free article] [PubMed] [Google Scholar]
- LANDON D. N., WILLIAMS P. L. ULTRASTRUCTURE OF THE NODE OF RANVIER. Nature. 1963 Aug 10;199:575–577. doi: 10.1038/199575a0. [DOI] [PubMed] [Google Scholar]
- Landon D. N., Langley O. K. The local chemical environment of nodes of Ranvier: a study of cation binding. J Anat. 1971 Apr;108(Pt 3):419–432. [PMC free article] [PubMed] [Google Scholar]
- Nonner W. A new voltage clamp method for Ranvier nodes. Pflugers Arch. 1969;309(2):176–192. doi: 10.1007/BF00586967. [DOI] [PubMed] [Google Scholar]
- Nonner W., Rojas E., Stämpfli H. Displacement currents in the node of Ranvier. Voltage and time dependence. Pflugers Arch. 1975;354(1):1–18. doi: 10.1007/BF00584499. [DOI] [PubMed] [Google Scholar]
- Palti Y., Ganot G., Stämpfli R. Effect of conditioning potential on potassium current kinetics in the frog node. Biophys J. 1976 Mar;16(3):261–273. doi: 10.1016/S0006-3495(76)85686-X. [DOI] [PMC free article] [PubMed] [Google Scholar]
- Palti Y., Gold R., Stämpfli R. Diffusion of ions in myelinated nerve fibers. Biophys J. 1979 Jan;25(1):17–31. doi: 10.1016/S0006-3495(79)85275-3. [DOI] [PMC free article] [PubMed] [Google Scholar]
- Palti Y., Moran N., Stämpfli R. Potassium currents and conductance. Comparison between motor and sensory myelinated fibers. Biophys J. 1980 Dec;32(3):955–966. doi: 10.1016/S0006-3495(80)85029-6. [DOI] [PMC free article] [PubMed] [Google Scholar]
- Poznansky M., Tong S., White P. C., Milgram J. M., Solomon A. K. Nonelectrolyte diffusion across lipid bilayer systems. J Gen Physiol. 1976 Jan;67(1):45–66. doi: 10.1085/jgp.67.1.45. [DOI] [PMC free article] [PubMed] [Google Scholar]
- VILLEGAS R., CAPUTO C., VILLEGAS L. Diffusion barrieres in the squid nerve fiber. The axolemma and the Schwann layer. J Gen Physiol. 1962 Nov;46:245–255. doi: 10.1085/jgp.46.2.245. [DOI] [PMC free article] [PubMed] [Google Scholar]
- Villegas G. M. Electron microscopic study of the giant nerve fiber of the giant squid Dosidicus gigas. J Ultrastruct Res. 1969 Mar;26(5):501–504. doi: 10.1016/s0022-5320(69)90054-9. [DOI] [PubMed] [Google Scholar]