Abstract
The patch-clamp technique of Mueller (1975, Ann. N.Y. Acad. Sci., 274:247-264) and Neher and Sakmann (1976, Nature (Lond.), 260:799-802) was modified to be suitable for single-channel measurements in lipid bilayers at potentials up to 500 mV. This method was used to study gramicidin A single-channel current-voltage characteristics. It was found that the sublinear current-voltage behavior normally observed at low permeant ion concentrations and rather low potentials (V less than or equal to 200 mV) continues to be seen all the way up to 500 mV. This phenomenon is characteristic of the low permeant ion situation in which the channel is far from saturation, and implies that the overall rate constant for association between ion and channel is very weakly, if at all, voltage dependent. The magnitude of the single channel currents at 500 mV is consistent with the notion that the aqueous convergence conductance is a significant factor in determining the permeability characteristics of the gramicidin A channel.
Full text
PDF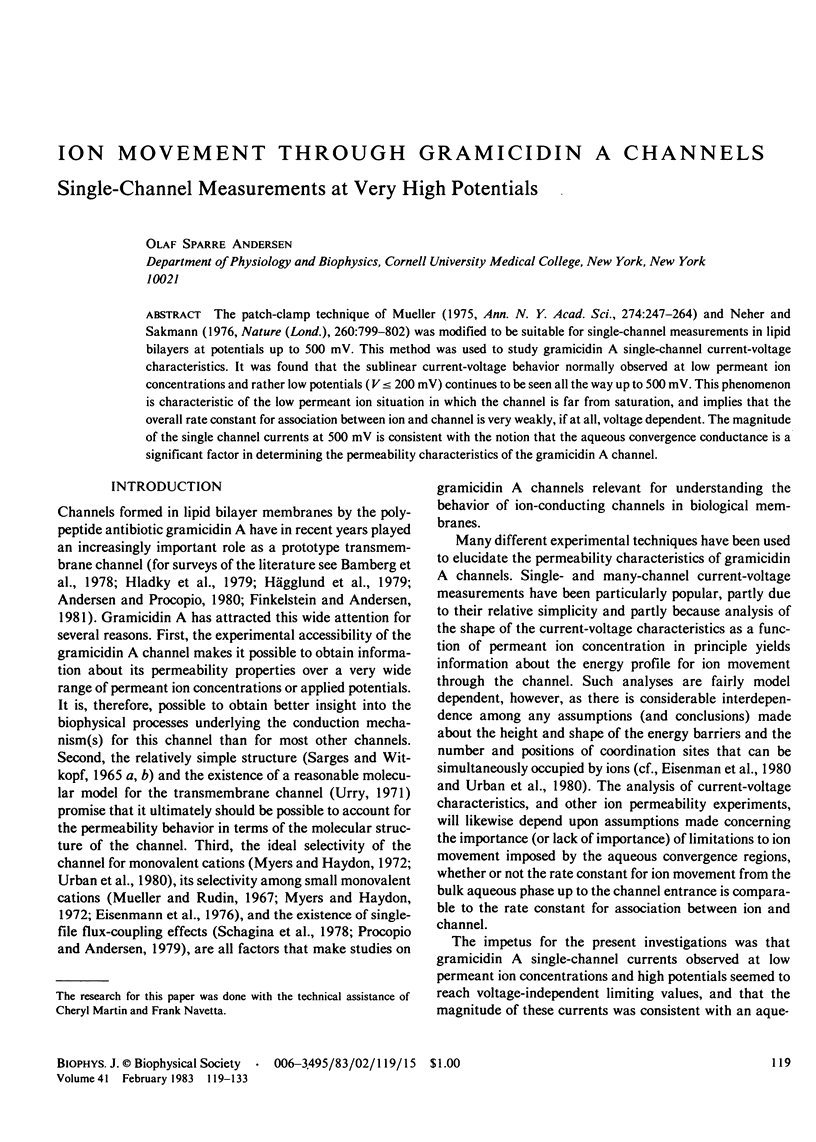
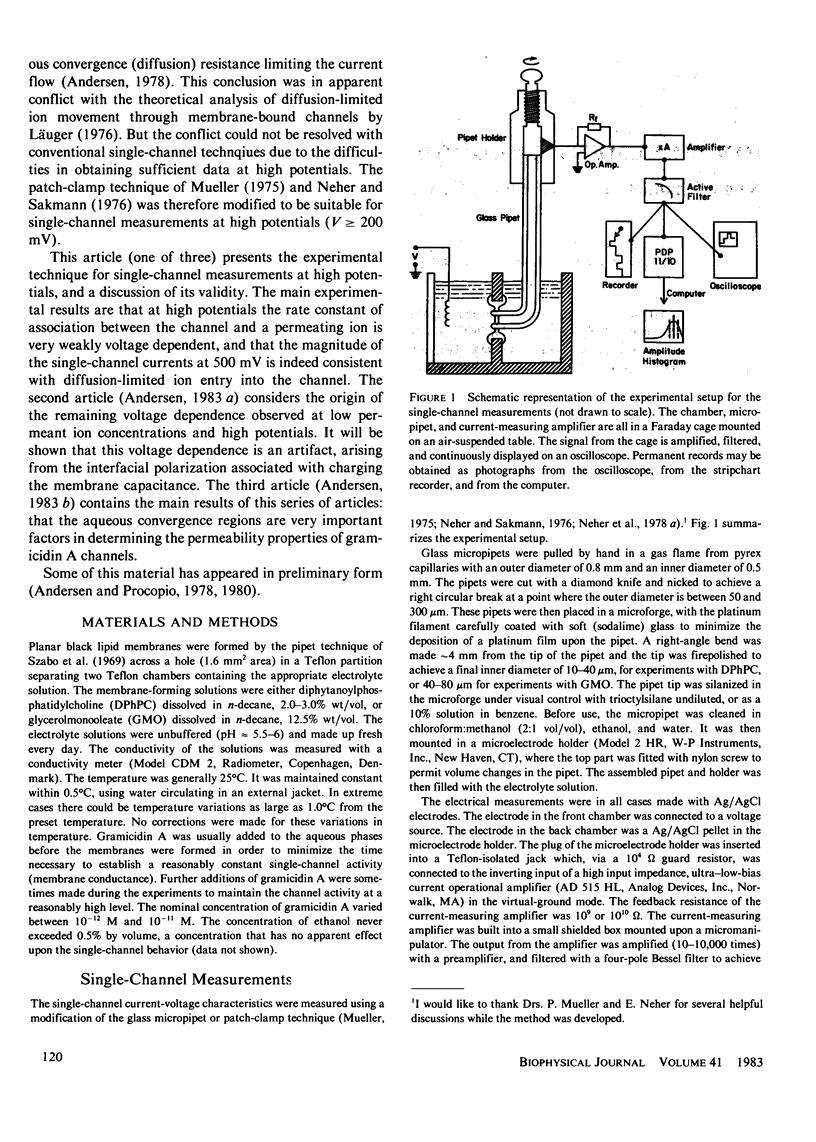
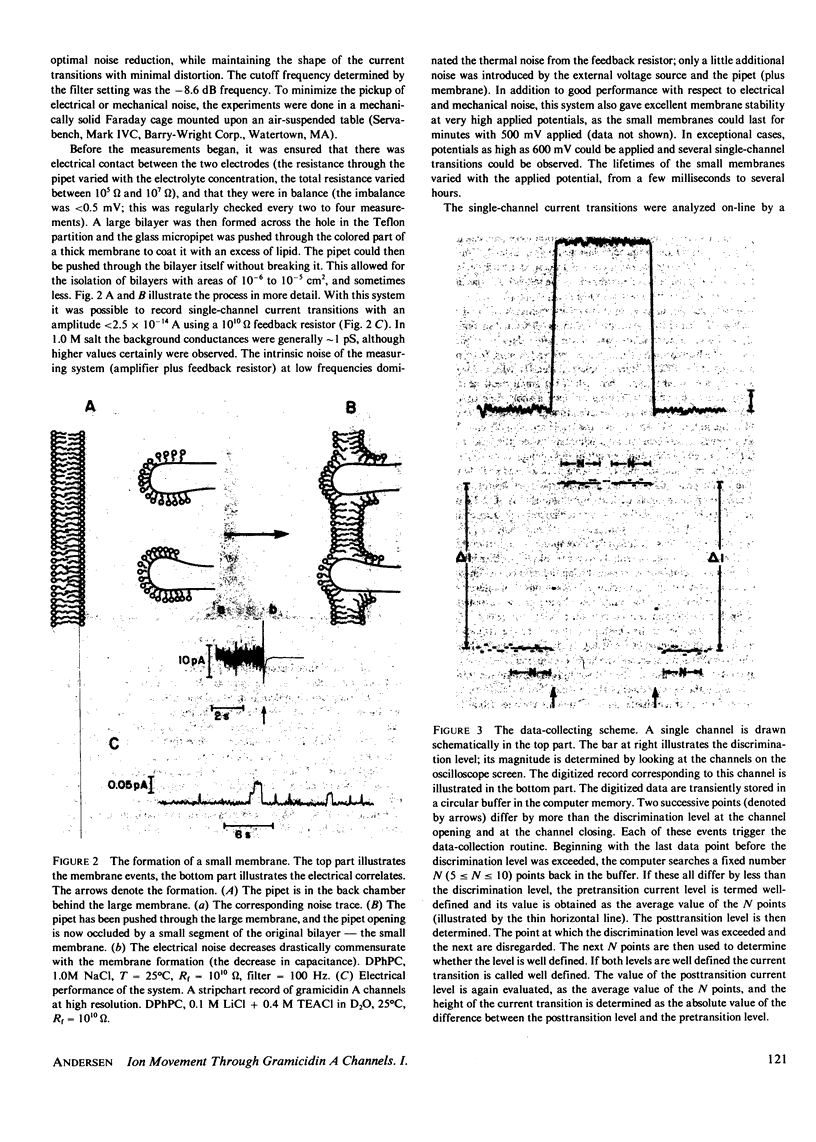
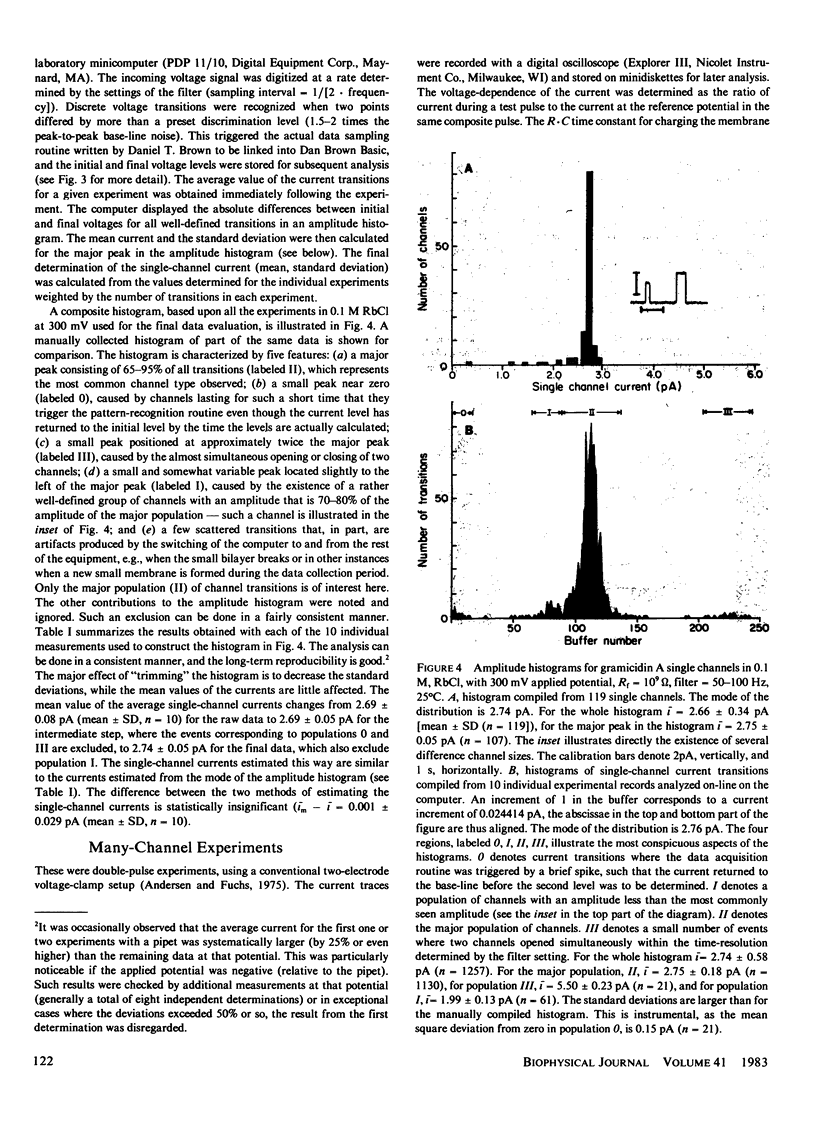
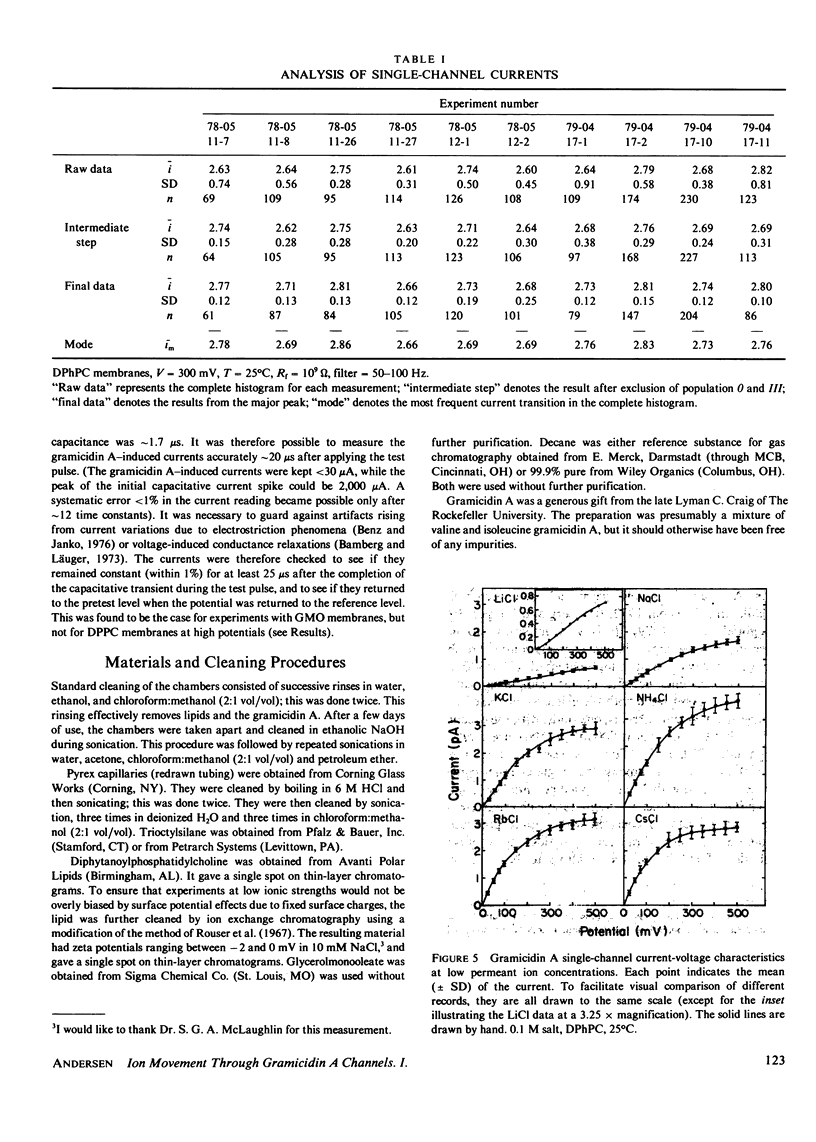
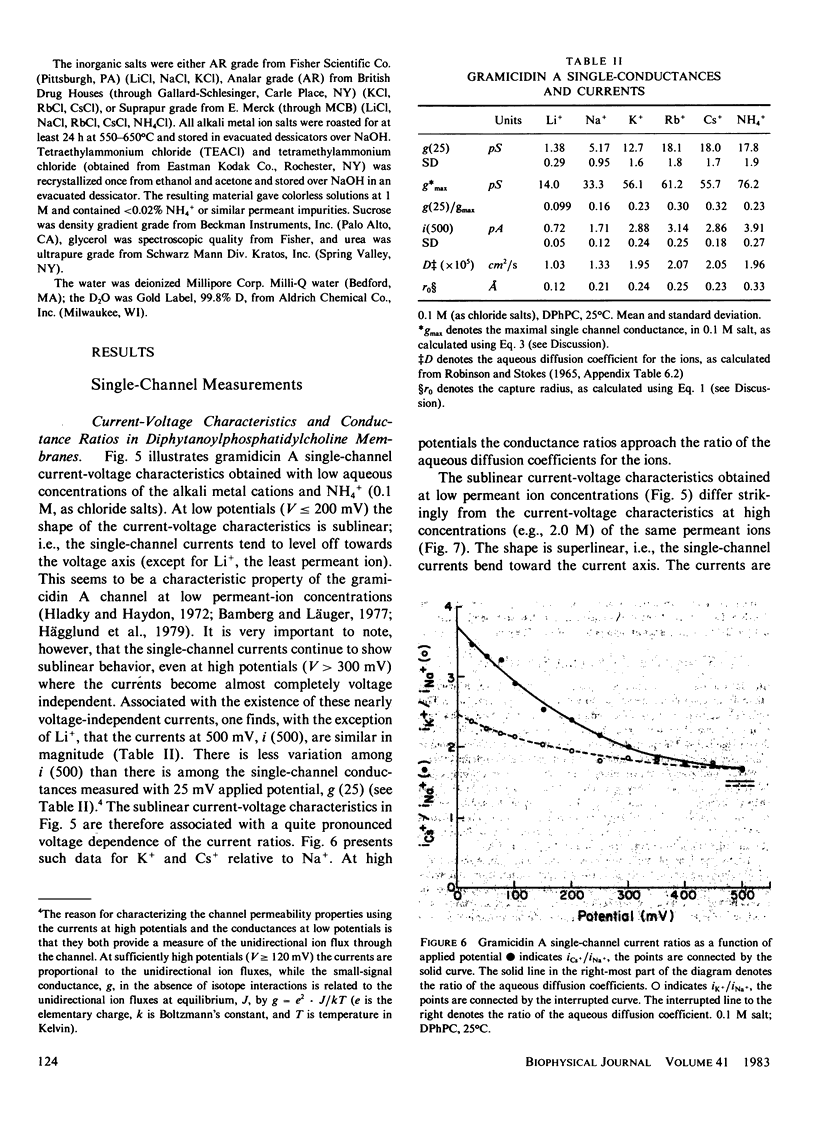
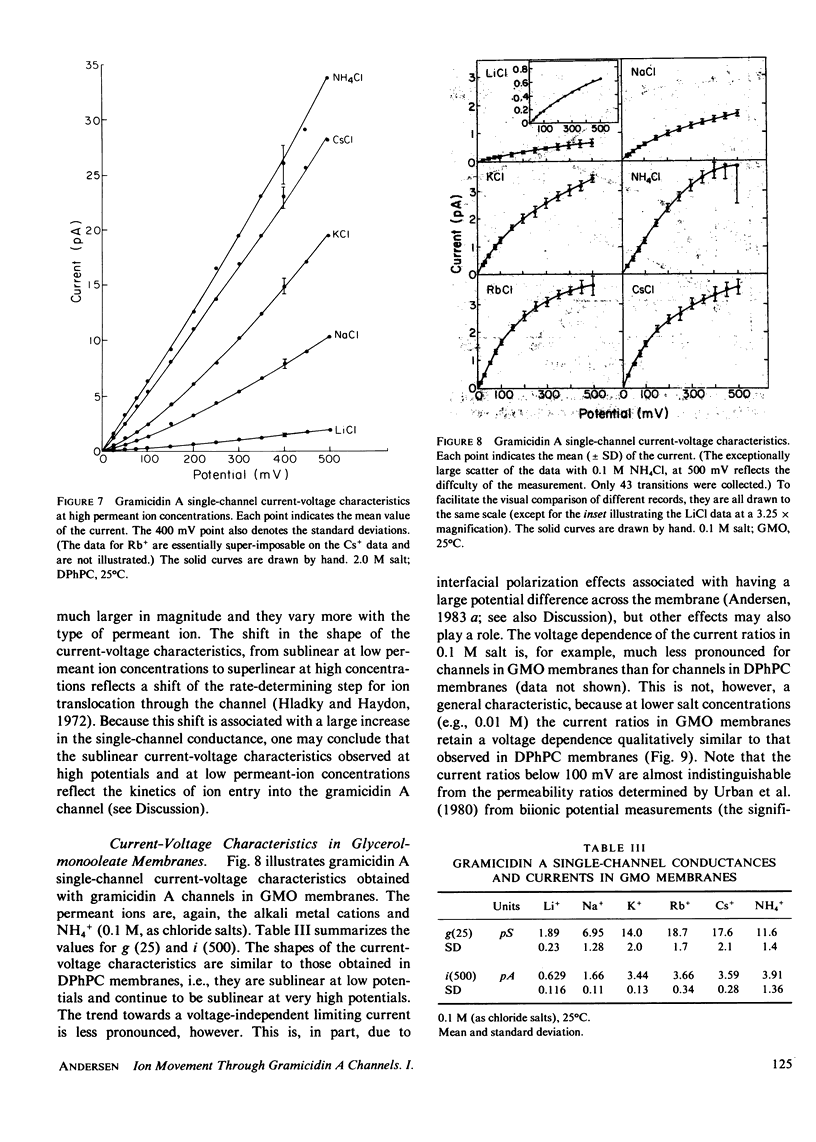
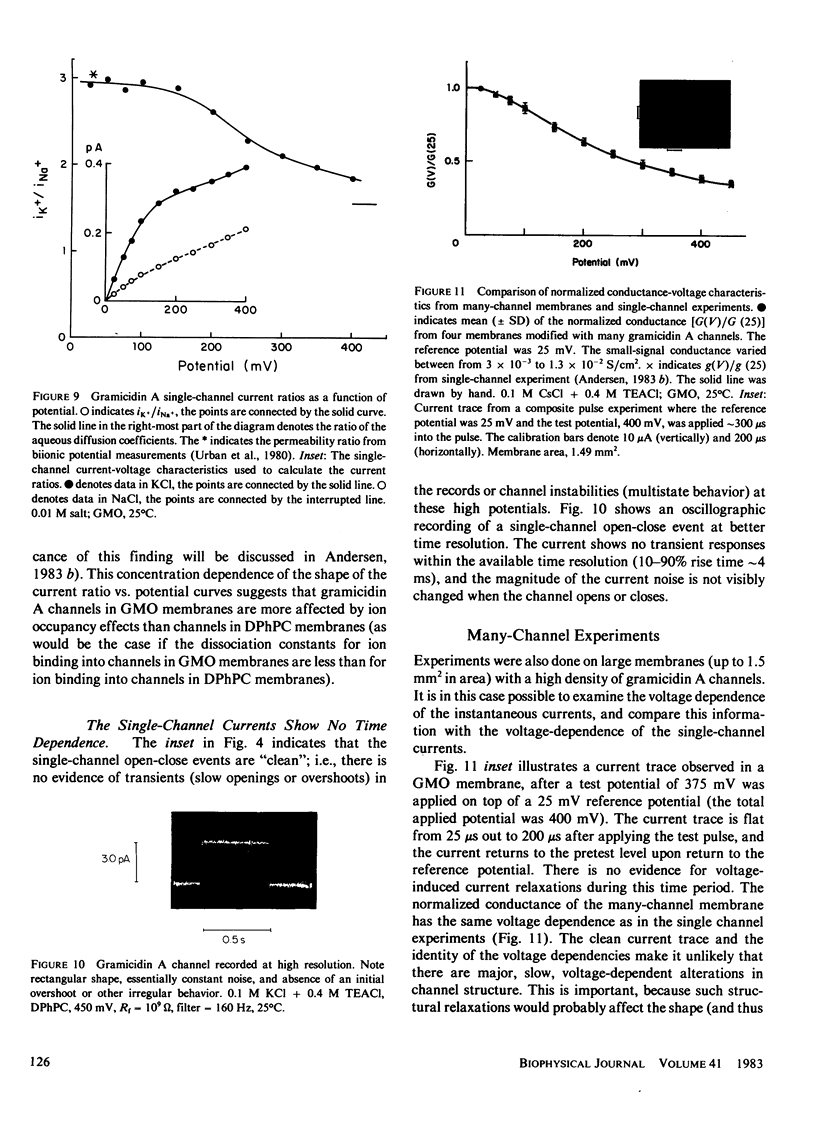
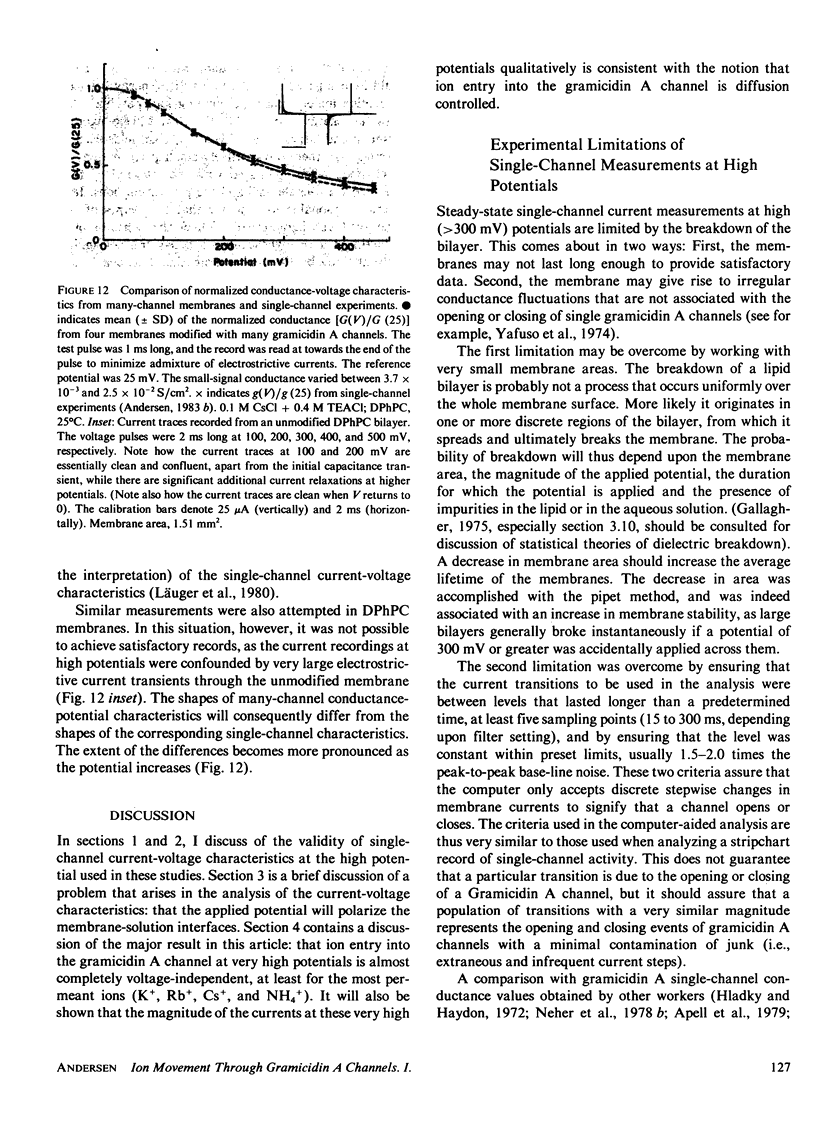
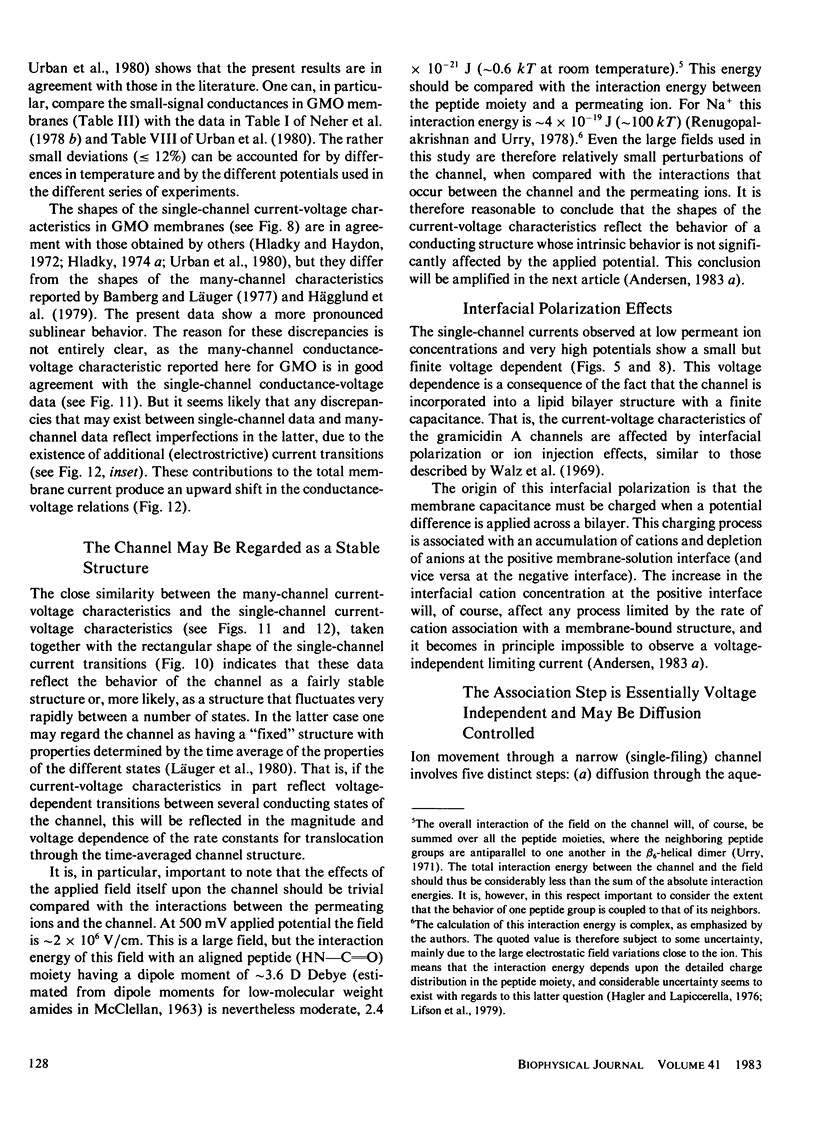
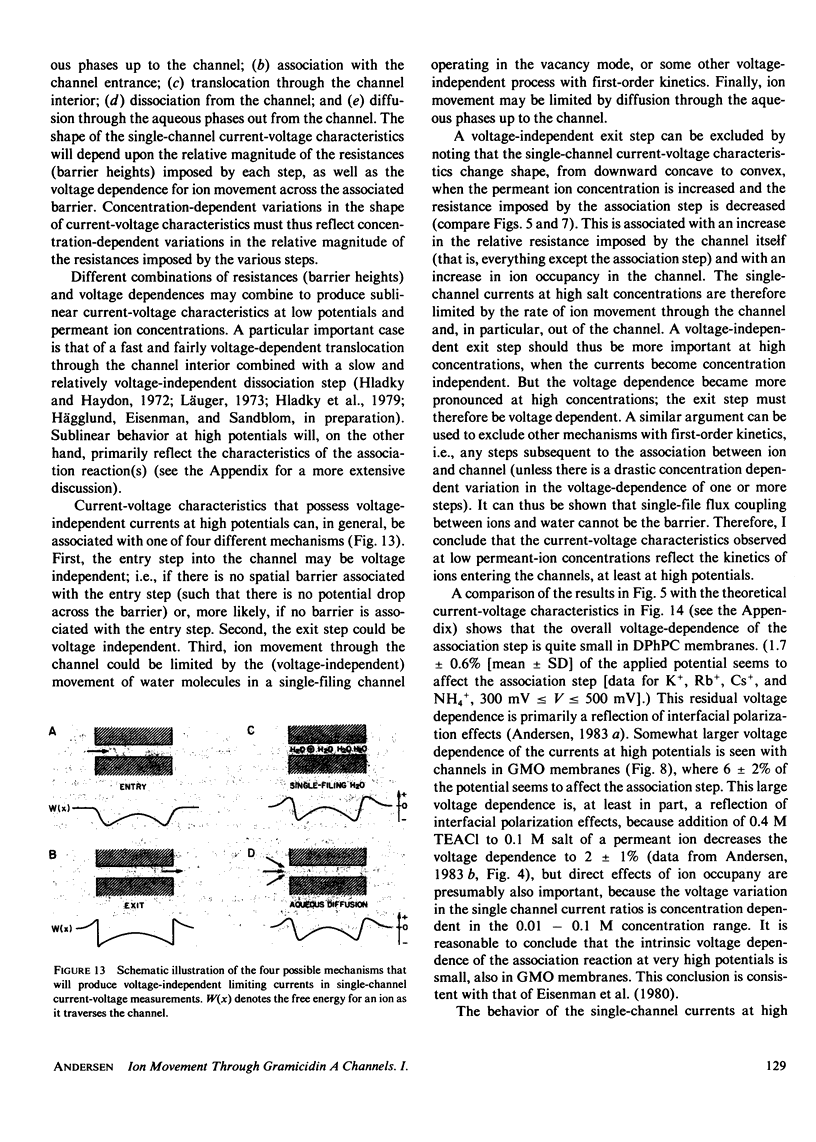
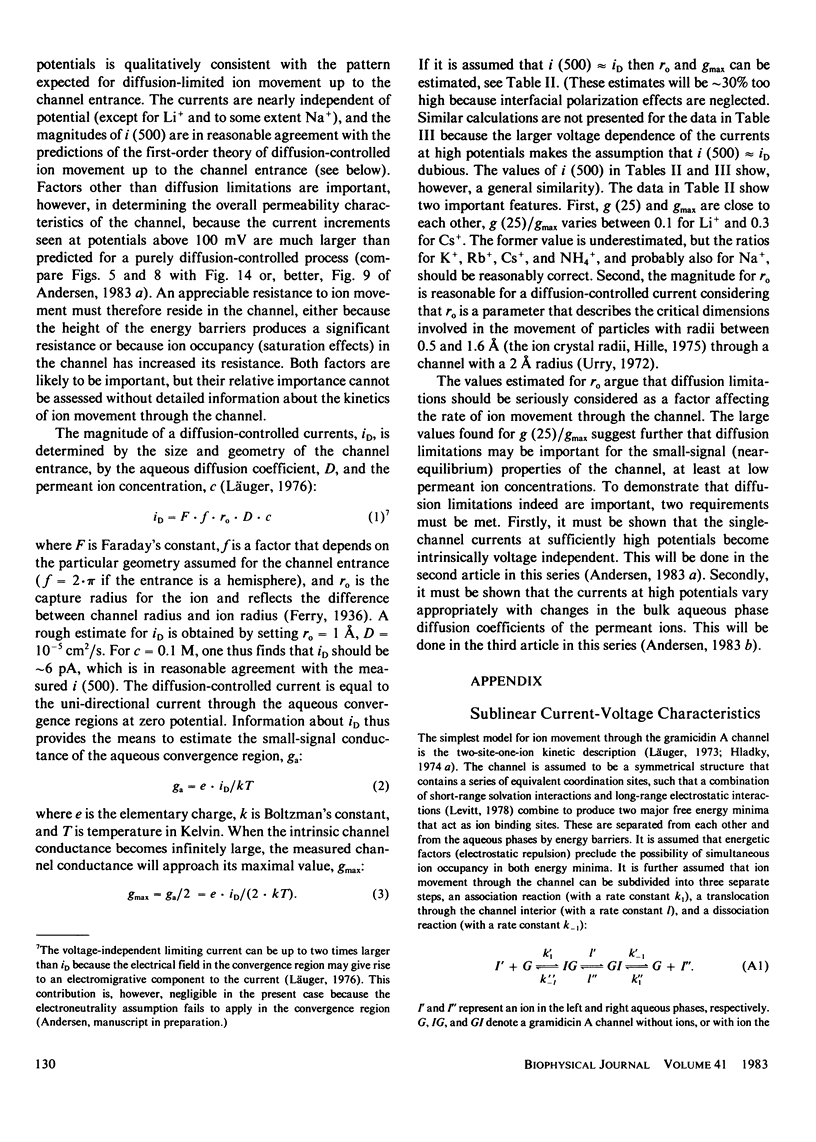
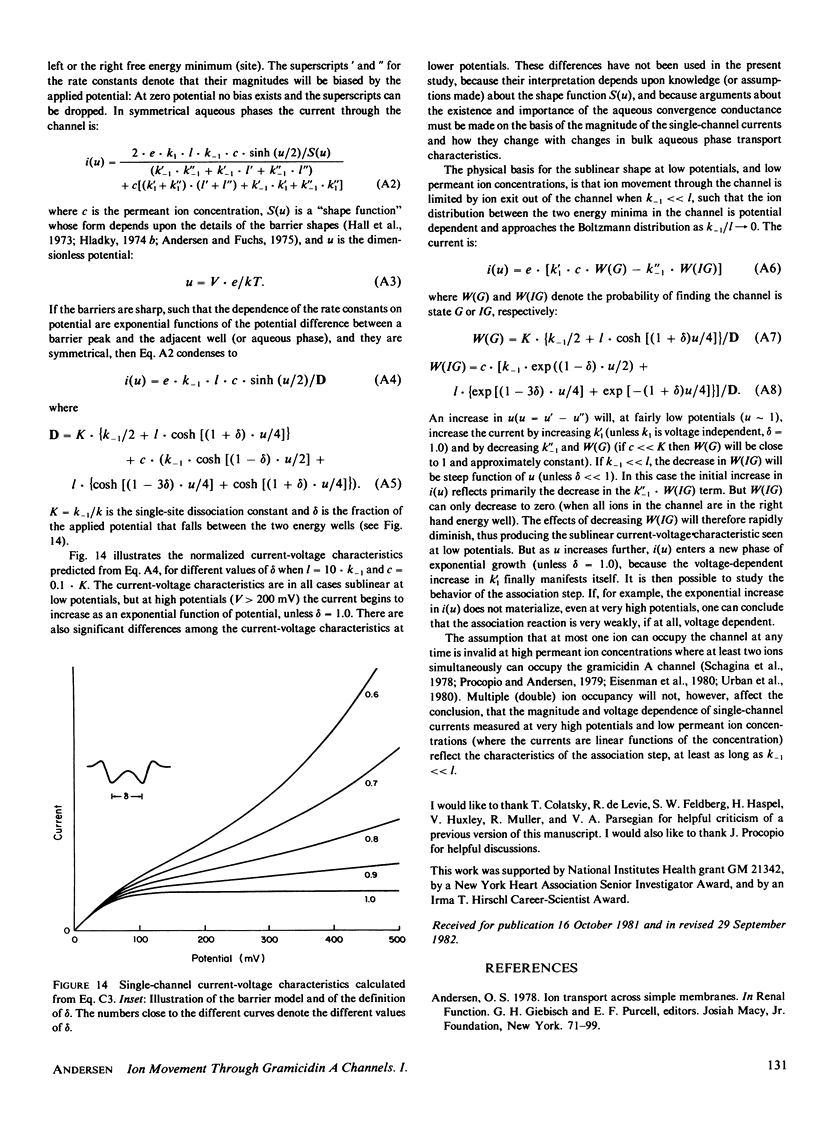
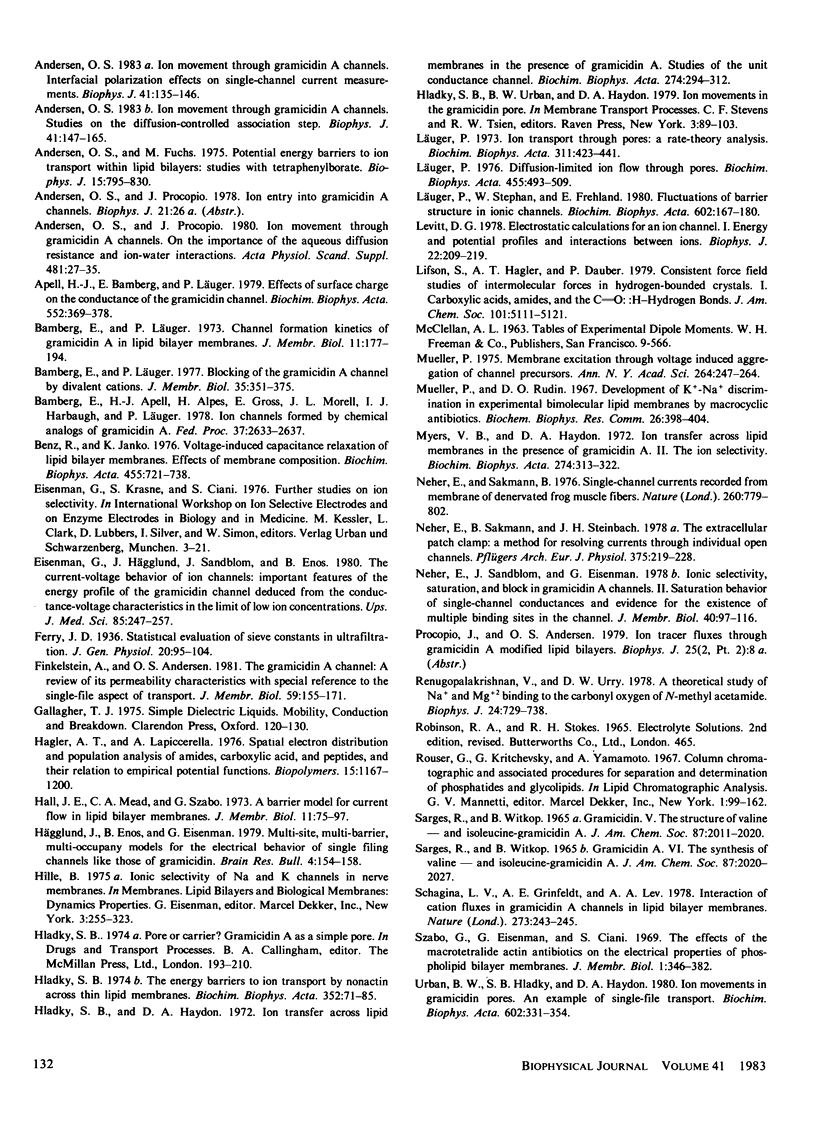
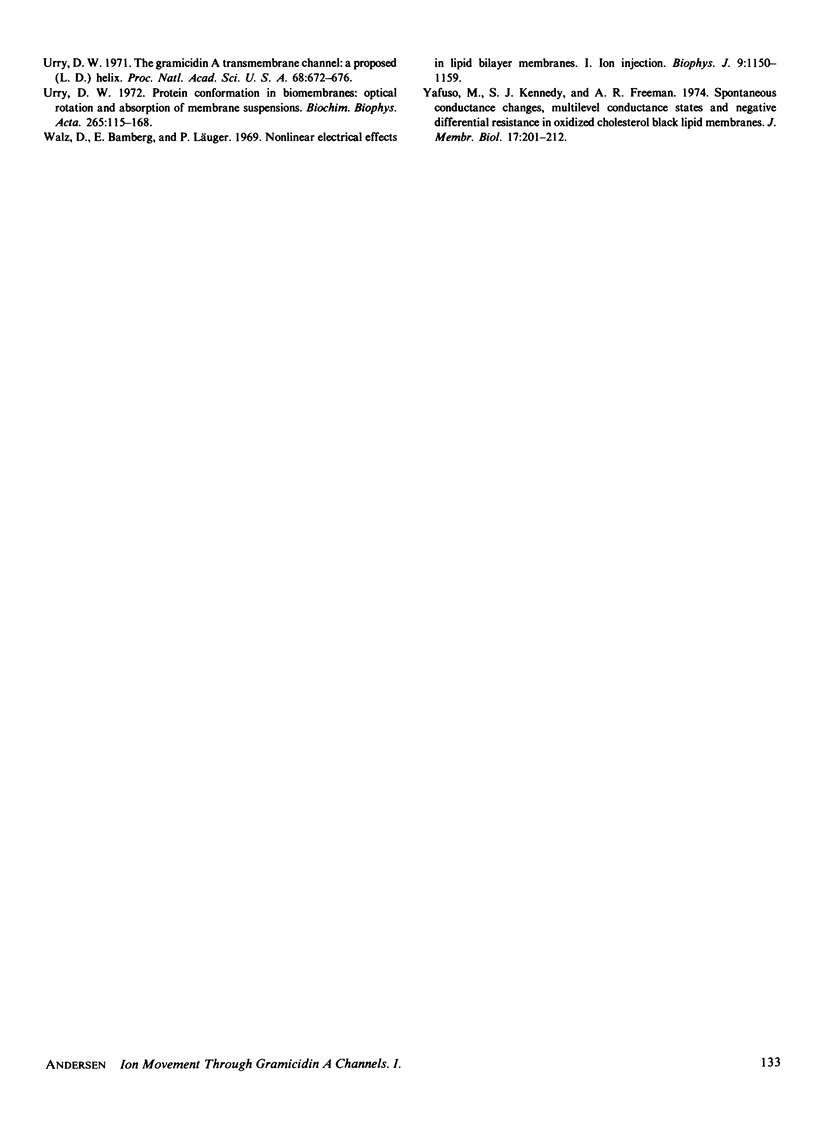
Selected References
These references are in PubMed. This may not be the complete list of references from this article.
- Andersen O. S., Fuchs M. Potential energy barriers to ion transport within lipid bilayers. Studies with tetraphenylborate. Biophys J. 1975 Aug;15(8):795–830. doi: 10.1016/S0006-3495(75)85856-5. [DOI] [PMC free article] [PubMed] [Google Scholar]
- Andersen O. S. Ion movement through gramicidin A channels. Interfacial polarization effects on single-channel current measurements. Biophys J. 1983 Feb;41(2):135–146. doi: 10.1016/S0006-3495(83)84415-4. [DOI] [PMC free article] [PubMed] [Google Scholar]
- Andersen O. S. Ion movement through gramicidin A channels. Studies on the diffusion-controlled association step. Biophys J. 1983 Feb;41(2):147–165. doi: 10.1016/S0006-3495(83)84416-6. [DOI] [PMC free article] [PubMed] [Google Scholar]
- Andersen O. S., Procopio J. Ion movement through gramicidin A channels. On the importance of the aqueous diffusion resistance and ion-water interactions. Acta Physiol Scand Suppl. 1980;481:27–35. [PubMed] [Google Scholar]
- Apell H. J., Bamberg E., Läuger P. Effects of surface charge on the conductance of the gramicidin channel. Biochim Biophys Acta. 1979 Apr 19;552(3):369–378. doi: 10.1016/0005-2736(79)90181-0. [DOI] [PubMed] [Google Scholar]
- Bamberg E., Apell H. J., Alpes H., Gross E., Morell J. L., Harbaugh J. F., Janko K., Läuger P. Ion channels formed by chemical analogs of gramicidin A. Fed Proc. 1978 Oct;37(12):2633–2638. [PubMed] [Google Scholar]
- Bamberg E., Läuger P. Channel formation kinetics of gramicidin A in lipid bilayer membranes. J Membr Biol. 1973;11(2):177–194. doi: 10.1007/BF01869820. [DOI] [PubMed] [Google Scholar]
- Benz R., Janko K. Voltage-induce capacitance relaxation of lipid bilayer membranes. Effects of membrane composition. Biochim Biophys Acta. 1976 Dec 14;455(3):721–738. doi: 10.1016/0005-2736(76)90043-2. [DOI] [PubMed] [Google Scholar]
- Eisenman G., Hägglund J., Sandblom J., Enos B. The current-voltage behavior of ion channels: important features of the energy profile of the gramicidin channel deduced from the conductance-voltage characteristic in the limit of low ion concentration. Ups J Med Sci. 1980;85(3):247–257. doi: 10.3109/03009738009179195. [DOI] [PubMed] [Google Scholar]
- Finkelstein A., Andersen O. S. The gramicidin A channel: a review of its permeability characteristics with special reference to the single-file aspect of transport. J Membr Biol. 1981 Apr 30;59(3):155–171. doi: 10.1007/BF01875422. [DOI] [PubMed] [Google Scholar]
- Hagler A. T., Lapiccirella A. Spatial electron distribution and population analysis of amides, carboxylic acid, and peptides, and their relation to empirical potential functions. Biopolymers. 1976 Jun;15(6):1167–1200. doi: 10.1002/bip.1976.360150612. [DOI] [PubMed] [Google Scholar]
- Hladky S. B., Haydon D. A. Ion transfer across lipid membranes in the presence of gramicidin A. I. Studies of the unit conductance channel. Biochim Biophys Acta. 1972 Aug 9;274(2):294–312. doi: 10.1016/0005-2736(72)90178-2. [DOI] [PubMed] [Google Scholar]
- Hladky S. B. The energy barriers to ion transport by nonactin across thin lipid membranes. Biochim Biophys Acta. 1974 May 30;352(1):71–85. doi: 10.1016/0005-2736(74)90180-1. [DOI] [PubMed] [Google Scholar]
- Hägglund J., Enos B., Eisenman G. Multi-site, multi-barrier, multi-occupancy models for the electrical behavior of single filing channels like those of gramicidin. Brain Res Bull. 1979 Jan-Feb;4(1):154–158. doi: 10.1016/0361-9230(79)90077-7. [DOI] [PubMed] [Google Scholar]
- Levitt D. G. Electrostatic calculations for an ion channel. I. Energy and potential profiles and interactions between ions. Biophys J. 1978 May;22(2):209–219. doi: 10.1016/S0006-3495(78)85485-X. [DOI] [PMC free article] [PubMed] [Google Scholar]
- Läuger P. Diffusion-limited ion flow through pores. Biochim Biophys Acta. 1976 Dec 2;455(2):493–509. doi: 10.1016/0005-2736(76)90320-5. [DOI] [PubMed] [Google Scholar]
- Läuger P. Ion transport through pores: a rate-theory analysis. Biochim Biophys Acta. 1973 Jul 6;311(3):423–441. doi: 10.1016/0005-2736(73)90323-4. [DOI] [PubMed] [Google Scholar]
- Läuger P., Stephan W., Frehland E. Fluctuations of barrier structure in ionic channels. Biochim Biophys Acta. 1980 Oct 16;602(1):167–180. doi: 10.1016/0005-2736(80)90299-0. [DOI] [PubMed] [Google Scholar]
- Mueller P. Membrane excitation through voltage-induced aggregation of channel precursors. Ann N Y Acad Sci. 1975 Dec 30;264:247–264. doi: 10.1111/j.1749-6632.1975.tb31487.x. [DOI] [PubMed] [Google Scholar]
- Mueller P., Rudin D. O. Development of K+-Na+ discrimination in experimental bimolecular lipid membranes by macrocyclic antibiotics. Biochem Biophys Res Commun. 1967 Feb 21;26(4):398–404. doi: 10.1016/0006-291x(67)90559-1. [DOI] [PubMed] [Google Scholar]
- Myers V. B., Haydon D. A. Ion transfer across lipid membranes in the presence of gramicidin A. II. The ion selectivity. Biochim Biophys Acta. 1972 Aug 9;274(2):313–322. doi: 10.1016/0005-2736(72)90179-4. [DOI] [PubMed] [Google Scholar]
- Neher E., Sakmann B. Single-channel currents recorded from membrane of denervated frog muscle fibres. Nature. 1976 Apr 29;260(5554):799–802. doi: 10.1038/260799a0. [DOI] [PubMed] [Google Scholar]
- Neher E., Sakmann B., Steinbach J. H. The extracellular patch clamp: a method for resolving currents through individual open channels in biological membranes. Pflugers Arch. 1978 Jul 18;375(2):219–228. doi: 10.1007/BF00584247. [DOI] [PubMed] [Google Scholar]
- Neher E., Sandblom J., Eisenman G. Ionic selectivity, saturation, and block in gramicidin A channels. II. Saturation behavior of single channel conductances and evidence for the existence of multiple binding sites in the channel. J Membr Biol. 1978 Apr 26;40(2):97–116. doi: 10.1007/BF01871143. [DOI] [PubMed] [Google Scholar]
- Renugopalakrishnan V., Urry D. W. A theoretical study of Na+ and Mg+2 binding to the carbonyl oxygen of N-methyl acetamide. Biophys J. 1978 Dec;24(3):729–738. doi: 10.1016/S0006-3495(78)85416-2. [DOI] [PMC free article] [PubMed] [Google Scholar]
- SARGES R., WITKOP B. GRAMICIDIN A. V. THE STRUCTURE OF VALINE- AND ISOLEUCINE-GRAMICIDIN A. J Am Chem Soc. 1965 May 5;87:2011–2020. doi: 10.1021/ja01087a027. [DOI] [PubMed] [Google Scholar]
- SARGES R., WITKOP B. GRAMICIDIN A. VI. THE SYNTHESIS OF VALINE- AND ISOLEUCINE-GRAMICIDIN A. J Am Chem Soc. 1965 May 5;87:2020–2027. doi: 10.1021/ja01087a028. [DOI] [PubMed] [Google Scholar]
- Schagina L. V., Grinfeldt A. E., Lev A. A. Interaction of cation fluxes in gramicidin A channels in lipid bilayer membranes. Nature. 1978 May 18;273(5659):243–245. doi: 10.1038/273243a0. [DOI] [PubMed] [Google Scholar]
- Urban B. W., Hladky S. B., Haydon D. A. Ion movements in gramicidin pores. An example of single-file transport. Biochim Biophys Acta. 1980 Nov 4;602(2):331–354. doi: 10.1016/0005-2736(80)90316-8. [DOI] [PubMed] [Google Scholar]
- Urry D. W. Protein conformation in biomembranes: optical rotation and absorption of membrane suspensions. Biochim Biophys Acta. 1972 Feb 14;265(1):115–168. doi: 10.1016/0304-4157(72)90021-4. [DOI] [PubMed] [Google Scholar]
- Urry D. W. The gramicidin A transmembrane channel: a proposed pi(L,D) helix. Proc Natl Acad Sci U S A. 1971 Mar;68(3):672–676. doi: 10.1073/pnas.68.3.672. [DOI] [PMC free article] [PubMed] [Google Scholar]
- Walz D., Bamberg E., Läuger P. Nonlinear electrical effects in lipid bilayer membranes. I. Ion injection. Biophys J. 1969 Sep;9(9):1150–1159. doi: 10.1016/S0006-3495(69)86442-8. [DOI] [PMC free article] [PubMed] [Google Scholar]
- Yafuso M., Kennedy S. J., Freeman A. R. Spontaneous conductance changes, multilevel conductance states and negative differential resistance in oxidized cholesterol black lipid membranes. J Membr Biol. 1974 Jul 12;17(3):201–212. doi: 10.1007/BF01870182. [DOI] [PubMed] [Google Scholar]