Abstract
Diffusion is a phenomenon of very widespread importance in molecular biophysics. Diffusion can determine the rates and character of the assembly of multisubunit structures, the binding of ligands to receptors, and the internal motions of molecules and assemblies that involve solvent surface displacements. Current computer simulation techniques provide much more detailed descriptions of diffusional processes than have been available in the past. Models can be constructed to include such realistic features as structural subunits at the submolecular level (domains, monomers, or atoms); detailed electrostatic charge distributions and corresponding solvent-screened inter- and intramolecular interactions; and hydrodynamic interactions. The trajectories can be analyzed either to provide direct information on biomolecular function (e.g., the bimolecular rate constant for formation of an electron-transfer complex between two proteins), or to provide or test models for the interpretation of experimental data (e.g., the time dependence of fluorescence depolarization for segments of DNA). Here, we first review the theory of diffusional simulations, with special emphasis on new techniques such as those for obtaining transport properties of flexible assemblies and rate constants of diffusion-controlled reactions. Then we survey a variety of recent applications, including studies of large-scale motion in DNA segments and substrate "steering" in enzyme-substrate binding. We conclude with a discussion of current work (e.g., formation of protein complexes) and possible areas for future work.
Full text
PDF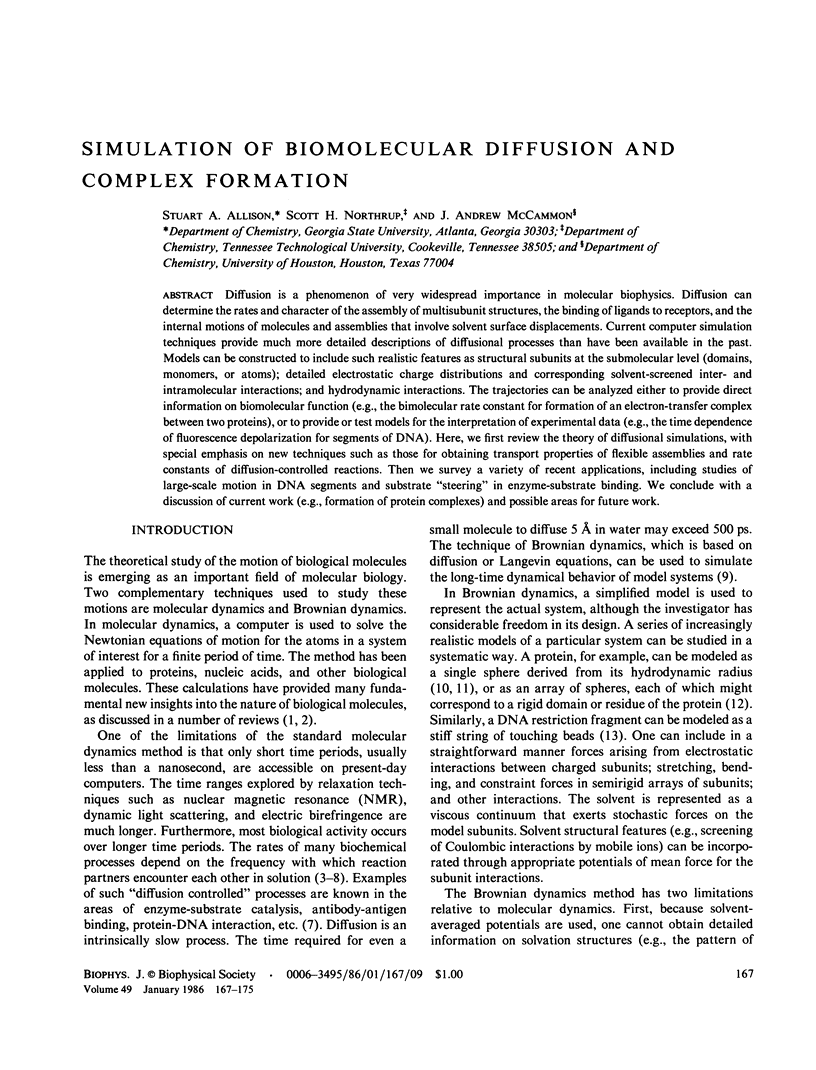
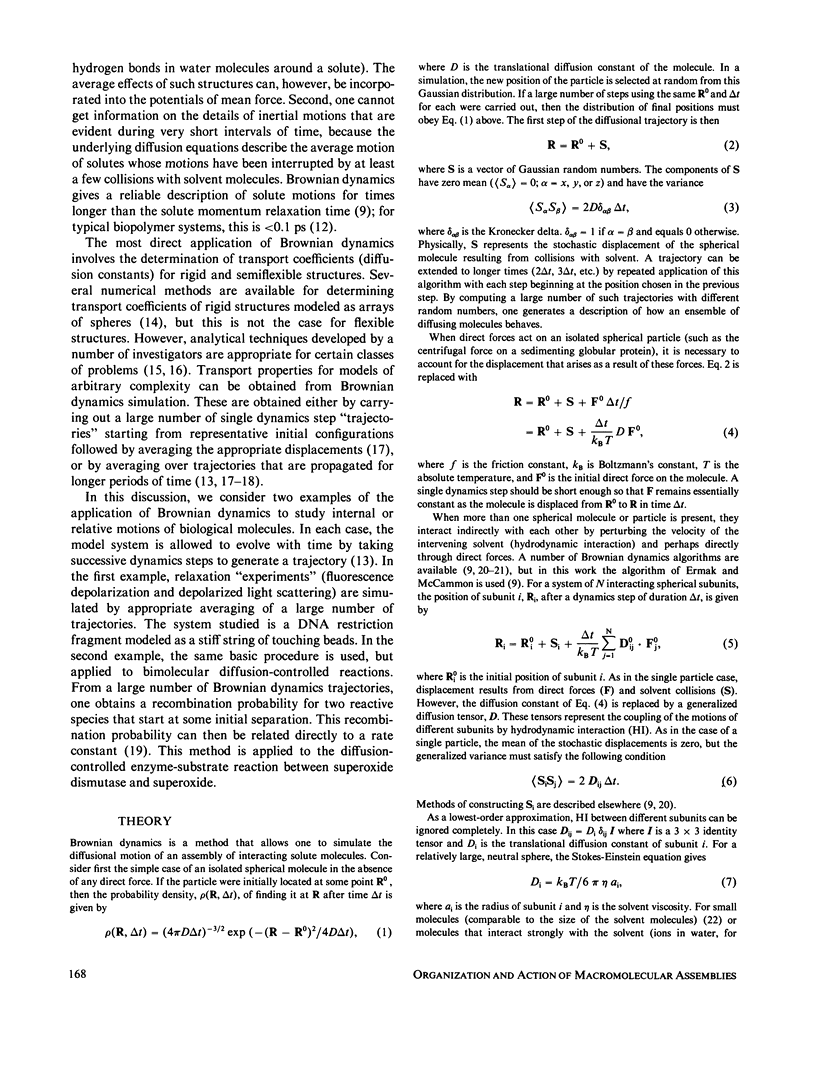
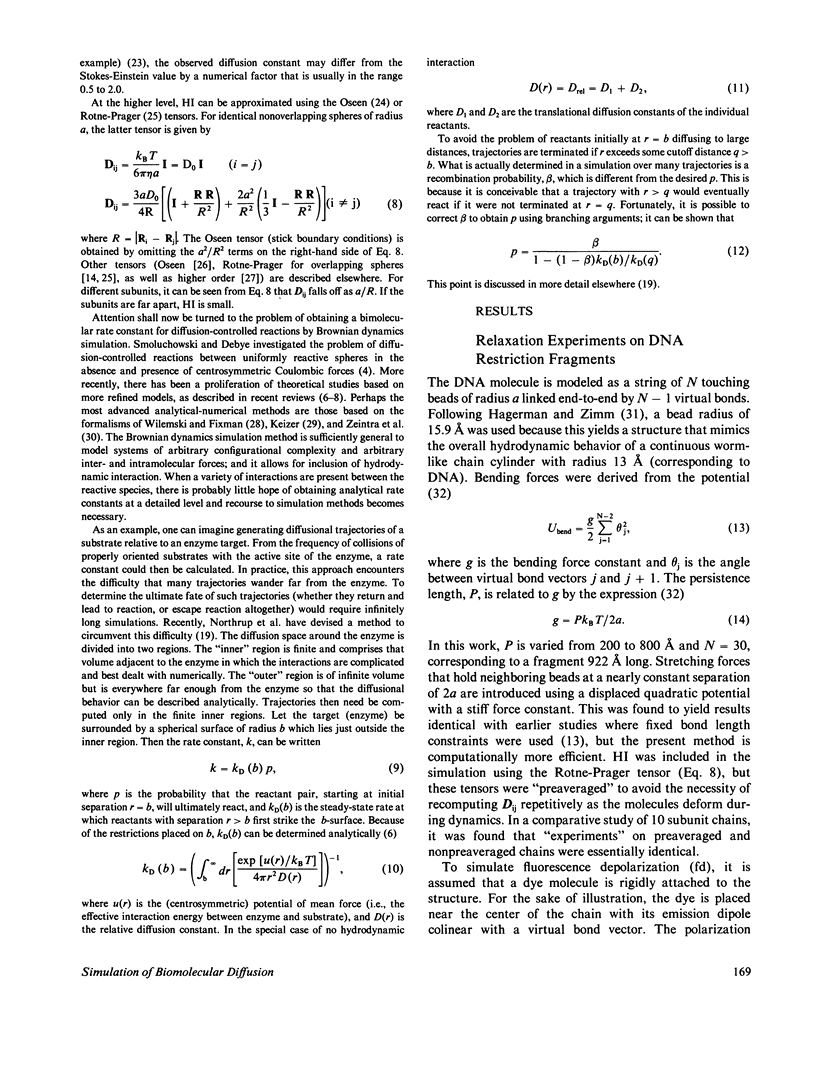
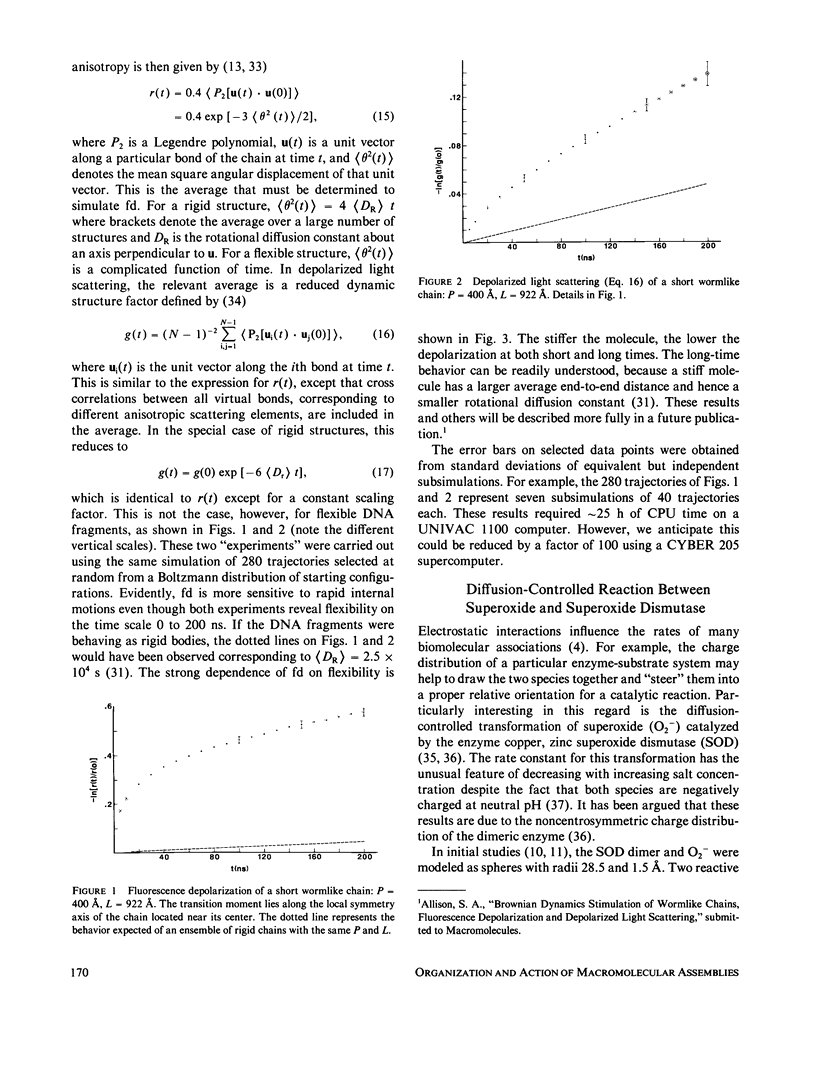
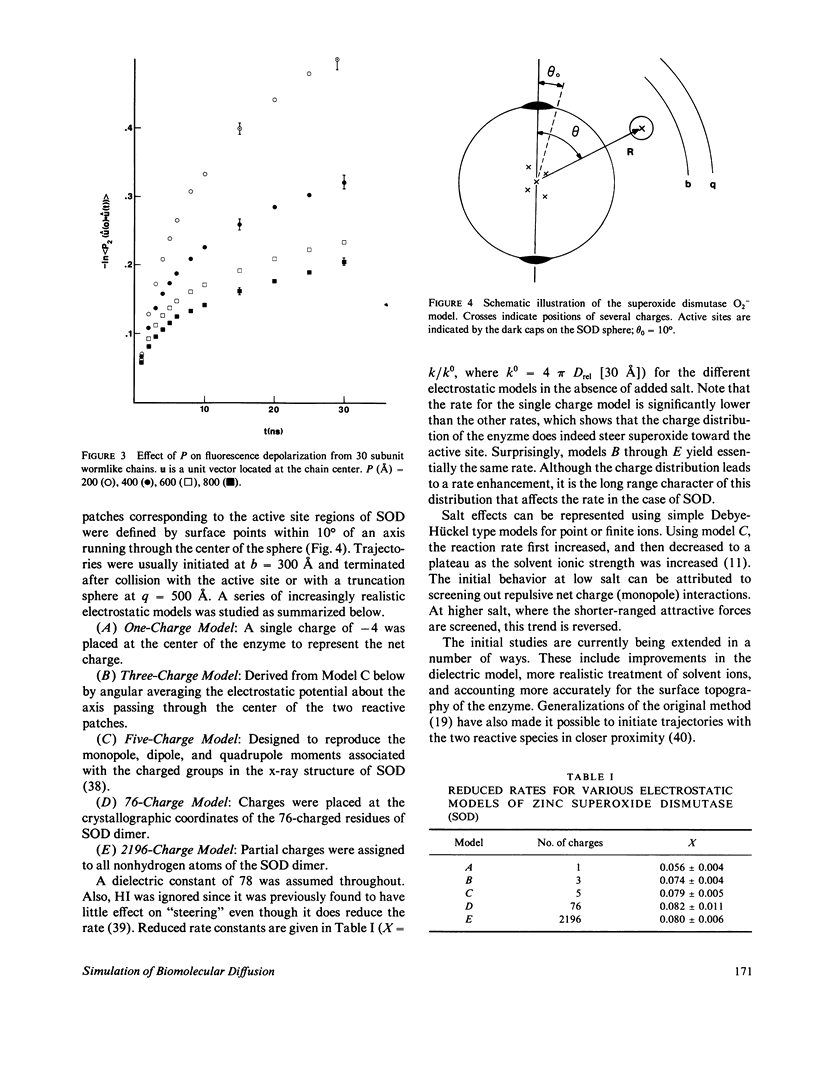
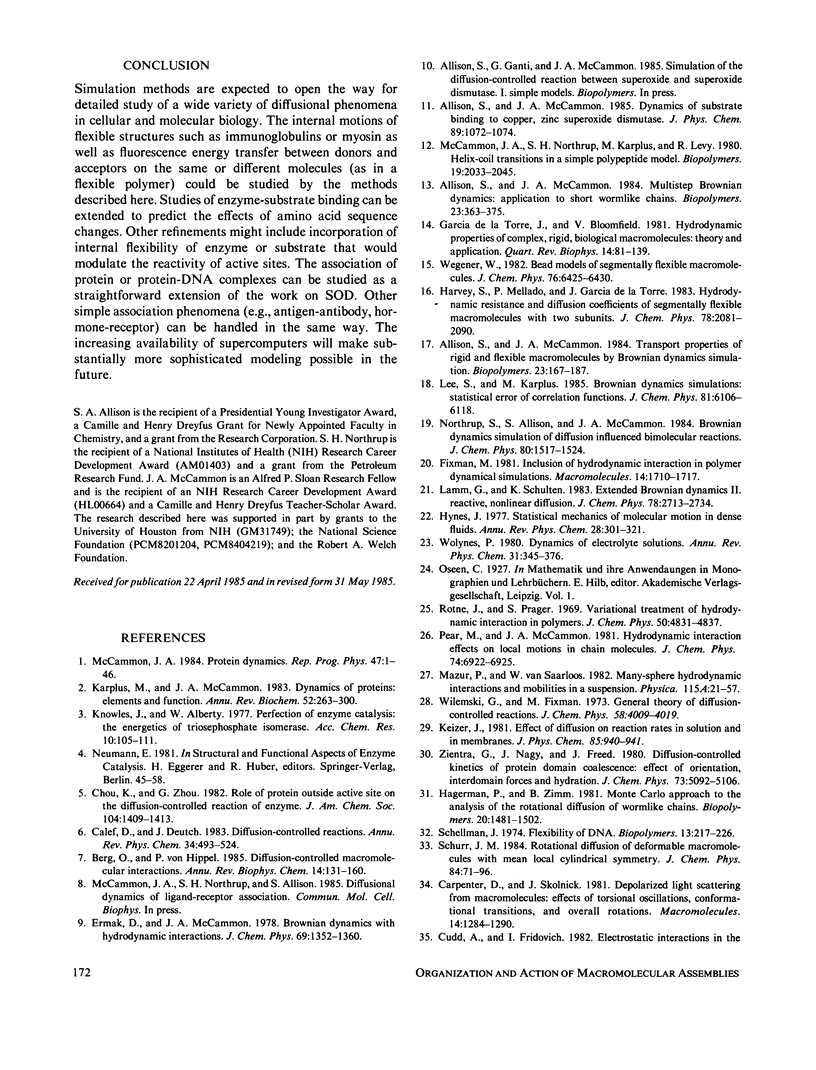
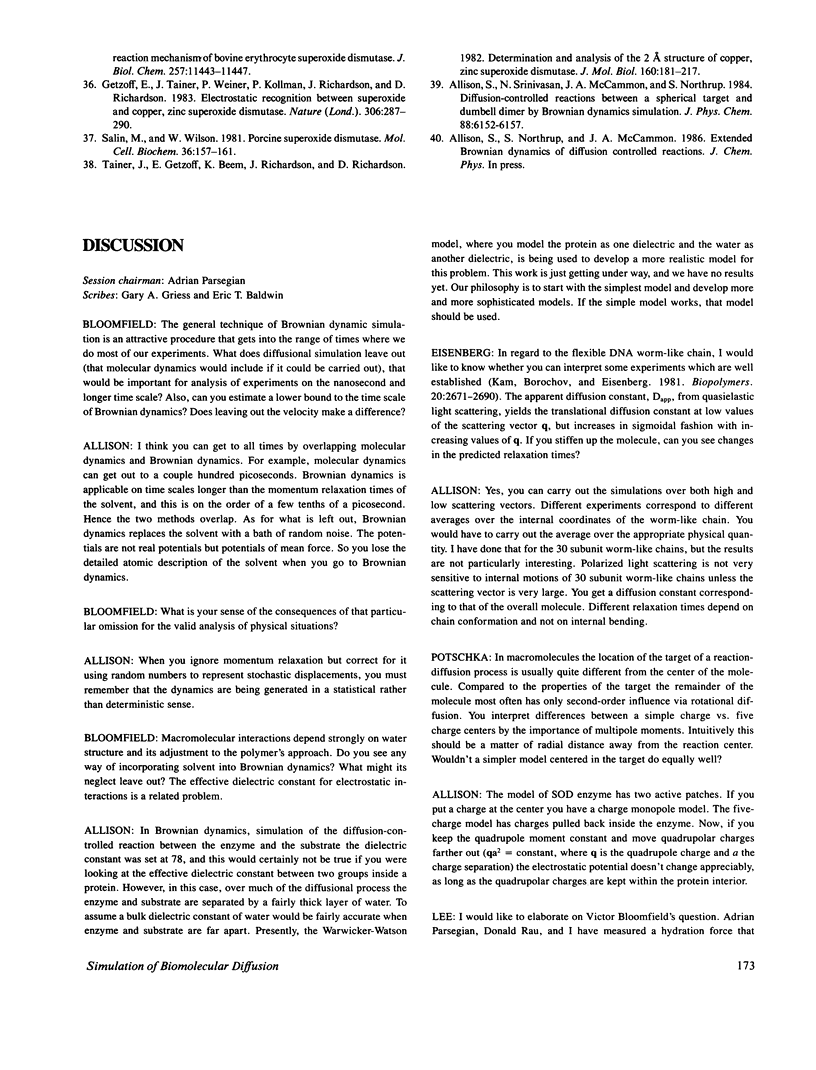
Selected References
These references are in PubMed. This may not be the complete list of references from this article.
- Allison S. A., McCammon J. A. Multistep Brownian dynamics: application to short wormlike chains. Biopolymers. 1984 Feb;23(2):363–375. doi: 10.1002/bip.360230214. [DOI] [PubMed] [Google Scholar]
- Berg O. G., von Hippel P. H. Diffusion-controlled macromolecular interactions. Annu Rev Biophys Biophys Chem. 1985;14:131–160. doi: 10.1146/annurev.bb.14.060185.001023. [DOI] [PubMed] [Google Scholar]
- Cudd A., Fridovich I. Electrostatic interactions in the reaction mechanism of bovine erythrocyte superoxide dismutase. J Biol Chem. 1982 Oct 10;257(19):11443–11447. [PubMed] [Google Scholar]
- Garcia de la Torre J. G., Bloomfield V. A. Hydrodynamic properties of complex, rigid, biological macromolecules: theory and applications. Q Rev Biophys. 1981 Feb;14(1):81–139. doi: 10.1017/s0033583500002080. [DOI] [PubMed] [Google Scholar]
- Getzoff E. D., Tainer J. A., Weiner P. K., Kollman P. A., Richardson J. S., Richardson D. C. Electrostatic recognition between superoxide and copper, zinc superoxide dismutase. Nature. 1983 Nov 17;306(5940):287–290. doi: 10.1038/306287a0. [DOI] [PubMed] [Google Scholar]
- Getzoff E. D., Tainer J. A., Weiner P. K., Kollman P. A., Richardson J. S., Richardson D. C. Electrostatic recognition between superoxide and copper, zinc superoxide dismutase. Nature. 1983 Nov 17;306(5940):287–290. doi: 10.1038/306287a0. [DOI] [PubMed] [Google Scholar]
- Karplus M., McCammon J. A. Dynamics of proteins: elements and function. Annu Rev Biochem. 1983;52:263–300. doi: 10.1146/annurev.bi.52.070183.001403. [DOI] [PubMed] [Google Scholar]
- Quart A. M., Yamane G. Multifocal oral squamous cell carcinoma. Quintessence Int Dent Dig. 1983 Feb;14(2):139–144. [PubMed] [Google Scholar]
- Salin M. L., Wilson W. W. Porcine superoxide dismutase. Isolation and characterization of a relatively basic cuprozinc enzyme. Mol Cell Biochem. 1981 May 26;36(3):157–161. doi: 10.1007/BF02357032. [DOI] [PubMed] [Google Scholar]
- Schellman J. A. Flexibility of DNA. Biopolymers. 1974 Jan;13(1):217–226. doi: 10.1002/bip.1974.360130115. [DOI] [PubMed] [Google Scholar]
- Tainer J. A., Getzoff E. D., Beem K. M., Richardson J. S., Richardson D. C. Determination and analysis of the 2 A-structure of copper, zinc superoxide dismutase. J Mol Biol. 1982 Sep 15;160(2):181–217. doi: 10.1016/0022-2836(82)90174-7. [DOI] [PubMed] [Google Scholar]